Objective
In this study, we evaluated the correlation of retinal thickness with peripheral nerve conduction and autonomic nerve function in diabetic patients.
Design
Cross-sectional study.
Methods
Medical records of 160 patients (mean age, 63.61 ± 12.52 years) with diabetes without diabetic retinopathy or mild nonproliferative diabetic retinopathy (NPDR) were reviewed. The mean retinal thickness of the parafoveal area and ganglion cell/inner plexiform layer (GC-IPL) thickness in 6 macular regions were measured using optical coherence tomography. Peripheral nerve conduction studies were conducted on peroneal and posterior tibial motor nerves and the sural sensory nerve. Five cardiovascular autonomic function tests were performed. We classified patients into groups by severity of peripheral neuropathy and autonomic dysfunction and analyzed the correlations with mean retinal thickness.
Results
The mean retinal thickness of the parafovea was 315.05 ± 12.70 μm and mean macular GC-IPL thickness was 79.89 ± 4.70 μm. Macular GC-IPL thickness showed significant correlation with peripheral nerve conduction (no peripheral neuropathy vs definite peripheral neuropathy: 82.0 ± 4.8 μm vs 75.2 ± 3.8 μm, P < .001). GC-IPL thickness decreased with severity of autonomic nerve dysfunction (no/mild dysfunction vs severe dysfunction: 81.2 ± 6.6 μm vs 77.6 ± 5.9 μm, P = .005). There was no significant correlation between the retinal thickness of the parafovea and electrodiagnostic tests.
Conclusion
The decrease of GC-IPL thickness was positively correlated with both peripheral nerve conduction and autonomic nerve function in diabetic patients who presented with no diabetic retinopathy or mild NPDR.
Diabetic retinal neurodegeneration is a progressive and degenerative process in the retina and is thought to occur prior to clinically detectable microvascular damage. Histologically, this can lead to neural apoptosis and glial cell activation that may predominately affect the retinal ganglion cells. Cell death of the ganglion cell layer may lead to thinning of the ganglion cell–inner plexiform layer (GC-IPL) that might be detected on optical coherence tomography (OCT) examination.
Recent studies have revealed that inflammation and consequent neuronal dysfunction have significant roles in the early pathogenesis of diabetic retinopathy. Genetic predisposition, which influences various cellular pathways, is also responsible for the development and progression of diabetic retinopathy. Some epigenetic modifications have been found to be prospective markers of proliferative diabetic retinopathy (PDR). However, to date, the underlying mechanism and association between retinal neurodegeneration and vascular complications remain unclear.
Fifty percent of diabetic patients develop diabetic polyneuropathy (DPN), but only about 20% have clinical features of neuropathy at the time of diabetes diagnosis, since it is often underdiagnosed owing to diverse and vague symptoms. Cardiac autonomic neuropathy (CAN), defined as impairment of autonomic control of the cardiovascular system, is the most common known manifestation due to life-threatening complications. Assessment with a CAN score at a subclinical stage can lead to earlier detection and treatment, preventing further systemic complications. DPN probably results from metabolic pathways triggered by hyperglycemia, which share features with microvascular abnormality in the retina in diabetic retinopathy. Consequently, a neuronal apoptotic signal is activated and glial reactivity increases, in addition to the endothelial injury and microvascular dysfunction, leading to nerve ischemia.
The purpose of this study was to investigate the clinical correlation between retinal GC-IPL thickness and the severity of peripheral neuropathy and autonomic nerve dysfunction in patients with no or minimal diabetic retinopathy. Furthermore, we hypothesized that OCT finding of thinner GC-IPL can be an early sign of diabetic neurodegeneration and requires careful clinical evaluation regarding diabetic neuropathy.
Methods
Study Design and Population
In this study, the medical records of 160 diabetic patients from Kyung Hee University Hospital, Seoul, South Korea, were retrospectively reviewed. The study was approved by the Kyung Hee University Hospital Institutional Review Board and conformed to the Declaration of Helsinki.
Inclusion criteria included known diagnosis of type 2 diabetes, age between 55 and 76 years, no sign of diabetic retinopathy or mild nonproliferative diabetic retinopathy (NPDR), and patients whose OCT examination and both peripheral and autonomic nerve function test were performed within a 6-month time frame. Exclusion criteria were duration of diabetes >10 years, diagnosis of any peripheral neurologic disease except diabetes-related neuropathy, medication for peripheral neuropathy prior to the nerve conduction test, cardiovascular disease, clinically significant diabetic macular edema, previous diagnosis of glaucoma, ocular hypertension, uveitis, other retinal diseases, any history of retinal treatment (laser photocoagulation, intravitreal injection, vitrectomy), and having been diagnosed as moderate/severe NPDR or PDR. Additionally, 60 age-matched patients without diabetes were included for comparison of GC-IPL thickness as a control group.
Alasil and associates reported an average reduction of 1.5 μm in the thickness of global retinal nerve fiber layer (RNFL) per increasing decade of age. To minimize the effect of age-related inner retinal thinning, we included patients aged 55–75 years. Because the duration of diabetes contributes to the prevalence of various systemic complications that might influence electrodiagnostic nerve tests, we excluded long-standing diabetes to reduce confounding effects.
Ophthalmic Examination
In each patient, the eye with the lowest visual acuity was selected for examination, unless this eye did not meet the eligibility criteria. Patients were classified as no diabetic retinopathy or mild NPDR on the basis of fundus photography images. No diabetic retinopathy was defined as the absence of all features of diabetic retinopathy, and mild NPDR was defined as the presence of a microaneurysm, retinal dot hemorrhage, or hard exudates according to the ETDRS severity scale.
We analyzed the mean retinal thickness (μm) of the parafoveal area within diameters of 1–3 mm and the GC-IPL thickness in 6 macular regions was analyzed using Cirrus HD-OCT (Carl Zeiss Meditec, Dublin, California, USA). A color-coded macular thickness map, that is, the macular cube (512 × 128 scan), was obtained with Cirrus HD-OCT. The alignment was properly positioned to the macula in the center of the scan using the iris and fundus viewports. The 9 standard ETDRS subfields consisted of 3 concentric circular areas centered on the fovea/fixation points. The diameters of the circular areas were 1, 3, and 6 mm ( Figure 1 ). The built-in algorithms of the Cirrus HD-OCT software (version 6.5.0.772) are capable of automatically identifying the outer boundary of the macular RNFL and the outer boundary of the inner plexiform layer (IPL). The difference between the RNFL and the IPL outer boundary segmentation yields the GC-IPL thickness ( Figure 2 , Top left). The average, minimum, and 6-sectoral (superior temporal, superior, superior nasal, inferior nasal, inferior, and inferior temporal) GC-IPL thicknesses are measured in an elliptical annulus with a vertical outer diameter of 4.0 mm and horizontal diameter of 4.8 mm ( Figure 2 , Top right, Bottom). In this study, the average GC-IPL thickness of 6 sectors was used for statistical analysis. Intrasession and interexaminer variability of GC-IPL thickness measurement were determined by calculating coefficients of variance (CV).
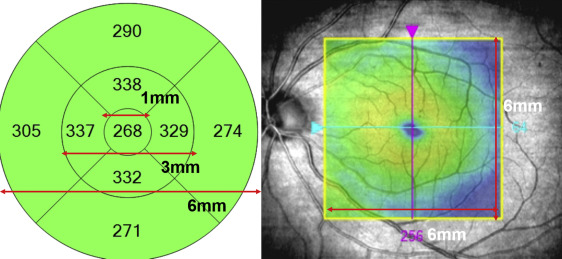
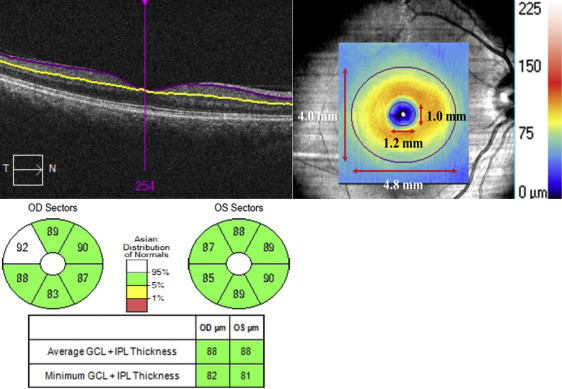
Assessment of Neuropathy
Diagnosis of DPN by clinical tests alone is not accurate and it is difficult to detect mild neuropathy in early DPN patients. It is generally accepted that the most accurate diagnostic method uses a combination of clinical symptoms, physical examination, and electrodiagnostic findings. Electrodiagnostic evaluation generally includes a nerve conduction study (NCS), which is most informative and highly specific. Motor NCSs are performed by applying electrical stimulation at points along the course of a motor nerve while recording the electrical response from an appropriate muscle. Sensory NCSs are performed by applying electrical stimulation near a nerve and recording the response from a distant site along the nerve.
In our study, NCS was reviewed in the peroneal motor nerve and the posterior tibial motor nerve by measuring velocity, terminal latency, and F-wave latency and the velocity in the sural sensory nerve. The normal range of terminal latency is 3.2–4.5 ms in both the peroneal motor and posterior tibial motor nerve. The normal ranges of conduction velocity in the peroneal motor, the posterior tibial motor, and the sural sensory nerve are 43–62 m/s, 41–61 m/s, and 34–49 m/s, respectively. The normal range of F-wave latency is 44.7 ± 4.7 ms in the peroneal motor and 44.9 ± 6.2 ms in the posterior tibial motor nerve.
Despite the previously proposed NCS criteria for the diagnosis of peripheral neuropathy, no established guidelines exist to date. The minimal criterion for electrodiagnostic confirmation of DPN is an abnormality of any value of nerve conduction in 2 separate nerves, 1 of which must be the sural nerve. In addition, the diagnosis of DPN was established and classified by electrophysiological criteria modified by Kwon and associates subsequent to the Diabetes Control and Complication Trial.
Based on previously reported classification, subjects in this study were subdivided into 3 groups: no neuropathy, probable neuropathy, and definite neuropathy. Patients with normal sural nerve velocity were classified as the no neuropathy group ( Table 1 ). Peripheral nerve involvement was defined when any 2 parameters (among terminal latency, F-wave latency, and conduction velocity) were abnormal in each motor nerve. To evaluate autonomic nerve function, 5 parameters of CAN were analyzed. CAN was diagnosed according to the American Diabetes Association (ADA) guidelines. They consisted of the Valsalva ratio (changes in heart rate during the Valsalva maneuver), lying to standing heart rate (30/15 ratio), R-R interval variation (maximum-minimum heart rate during expiration and inspiration), postural hypotension (change in systolic blood pressure after standing), and sustained handgrip (increase in diastolic blood pressure after the handgrip exercise). ADA recommendations of value ranges are normal Valsalva ratio >1.2; normal lying to standing heart rate >1.04, abnormal <1.00; normal R-R interval variation >15 beats per minute (bpm), abnormal <10 bpm; normal postural hypotension <10 mm Hg, abnormal >30 mm Hg; and normal sustained handgrip >16 mm Hg, abnormal <10 mm Hg. The result of each test was sorted into either normal, borderline, or abnormal, which matched a score of 0, 1 and 2, respectively. Borderline was scored when values were between the normal and abnormal ranges. Patients were then subdivided into 3 groups based on the total CAN score: no/mild dysfunction 0–3, moderate dysfunction 4–6, and severe dysfunction 7–10 ( Table 2 ).
Group | Criteria |
---|---|
No neuropathy | No sural nerve involvement |
Probable neuropathy | Sural nerve + any 1 nerve involvement |
Definite neuropathy | Sural nerve + any 2 nerve involvement |
Group | Sum of Score (0–10) |
---|---|
No or mild dysfunction | 0–3 |
Moderate dysfunction | 4–6 |
Severe dysfunction | 7–10 |
Statistical Analysis
Statistical analysis was performed with SPSS software version 14.0 (SPSS Inc, Chicago, Illinois, USA). Intrasession and interexaminer repeatability of GC-IPL thickness measurement was assessed based on the intraclass correlation coefficient (ICC) and CV. The linear relationships between the GC-IPL thickness and the 3 peripheral nerve velocities were analyzed by the Pearson correlation method and the results were displayed in combined plots. Pearson correlation coefficient is used to identify linear correlation (dependence) between 2 variables, giving a value between +1 and −1, where 1 is complete positive correlation, 0 is no correlation, and −1 is total negative correlation, with P value < .05 considered as statistical significance.
Demographic data of patients were compared by 1-way and 2-ways analysis of variance (ANOVA), χ 2 , and Fisher exact test. In cases of significant results ( P < .05), the ANOVA was followed by a Bonferroni multiple-comparisons post hoc test. Multinomial logistic regression analysis was performed to evaluate the role of confounding factors in correlation between GC-IPL thickness and peripheral and autonomic neuropathy.
Results
In total, 160 patients with diabetes were included in the analysis. Baseline demographic and metabolic/neurologic data were shown according to peripheral neuropathy and autonomic nerve function, respectively ( Tables 3 and 4 ). The mean time interval between the OCT examination and nerve function tests in each patient was 3.88 ± 1.69 months (range 0–6 months). The mean age was 63.6 ± 9.43 years, the mean retinal thickness of the parafovea was 315.05 ± 12.70 μm, and mean macular GC-IPL thickness was 79.89 ± 4.70 μm.
No Neuropathy | Probable Neuropathy | Definite Neuropathy | P Value | |
---|---|---|---|---|
Number of subjects | 96 | 21 | 43 | |
Age (y) | 63.2 ± 5.9 | 66.19 ± 5.7 | 67.05 ± 6.1 | .054 |
BMI (kg/m 2 ) | 24.6 ± 3.3 | 24.5 ± 2.8 | 24.9 ± 3.3 | .870 |
Presence of hypertension (n) | 71 | 17 | 26 | .753 |
Presence of stroke (n) | 4 | 3 | 3 | .811 |
History of insulin treatment (n) | 11 | 3 | 5 | .862 |
Diabetic duration (y) | 5.3 ± 4.1 | 6.3 ± 4.1 | 5.5 ± 4.4 | .619 |
HbA1c (%) | 7.2 ± 1.1 | 7.1 ± 0.8 | 7.3 ± 1.2 | .732 |
Total cholesterol (mmol/L) | 159.5 ± 36.8 | 159.7 ± 30.4 | 160.8 ± 33.9 | .978 |
Retinal thickness of parafoveal 1–3 mm (μm) | 314.9 ± 20.7 | 314.2 ± 20.8 | 314.5 ± 17.2 | .873 |
GC-IPL thickness (μm) | 82.0 ± 5.8 | 78.8 ± 5.0 | 75.2 ± 4.2 | .002 a |
LogMAR BCVA | 0.08 ± 0.12 | 0.13 ± 0.13 | 0.12 ± 0.13 | .129 |
BUN (mg/dL) | 15.4 ± 5.9 | 17.4 ± 6.6 | 16.7 ± 4.9 | .226 |
Creatinine (mg/dL) | 0.83 ± 0.47 | 0.76 ± 0.24 | 0.83 ± 0.25 | .770 |
CAN score | 3.75 ± 2.63 | 4.14 ± 2.21 | 4.61 ± 2.33 | .172 |
Peroneal terminal latency (ms) | 3.5 ± 0.73 | 3.7 ± 0.61 | 4.6 ± 1.3 | <.0001 a |
Peroneal velocity (m/s) | 45.4 ± 5.3 | 41.7 ± 3.9 | 37.7 ± 3.0 | <.0001 a |
Peroneal F-wave | 48.4 ± 5.2 | 49.6 ± 4.4 | 57.4 ± 6.1 | <.0001 a |
Posterior tibial latency (ms) | 3.3 ± 0.49 | 4.0 ± 0.94 | 4.3 ± 0.82 | <.0001 a |
Posterior tibial velocity (m/s) | 44.2 ± 5.4 | 40.4 ± 3.5 | 37.8 ± 3.9 | <.0001 a |
Posterior tibial F-wave | 48.8 ± 5.3 | 49.8 ± 3.5 | 56.6 ± 3.9 | <.0001 a |
Sural sensory velocity (m/s) | 39.7 ± 3.0 | 33.7 ± 2.5 | 32.3 ± 2.7 | <.0001 a |
No/Mild Dysfunction | Moderate Dysfunction | Severe Dysfunction | P Value | |
---|---|---|---|---|
Number of patients | 42 | 69 | 49 | |
Age (y) | 63.5 ± 6.0 | 64.5 ± 5.4 | 66.7 ± 5.7 | .06 |
BMI (kg/m 2 ) | 25.3 ± 3.8 | 24.3 ± 3.1 | 24.7 ± 2.9 | .309 |
Presence of hypertension (n) | 26 | 51 | 37 | .105 |
Presence of stroke (n) | 2 | 4 | 4 | .872 |
History of insulin treatment (n) | 4 | 10 | 5 | .625 |
Diabetic duration (y) | 4.2 ± 4.1 | 6.0 ± 4.1 | 6.0 ± 4.2 | .052 |
HbA1c (%) | 7.0 ± 0.81 | 7.2 ± 1.2 | 7.5 ± 1.2 | .165 |
Total cholesterol (mmol/L) | 166.5 ± 34.4 | 161.5 ± 28.6 | 151.8 ± 42.3 | .119 |
Retinal thickness of parafoveal 1–3 mm (μm) | 317.4 ± 20.0 | 314.1 ± 18.8 | 314.5 ± 20.9 | .674 |
GC-IPL thickness (μm) | 82.2 ± 6.6 | 81.8 ± 3.9 | 76.6 ± 5.9 | .005 a |
LogMAR BCVA | 0.04 ± 0.05 | 0.08 ± 0.10 | 0.16 ± 0.17 | <.0001 a |
BUN (mg/dL) | 14.8 ± 4.2 | 15.6 ± 5.4 | 17.7 ± 7.00 | .033 a |
Creatinine (mg/dL) | 0.73 ± 0.23 | 0.76 ± 0.31 | 0.98 ± 0.53 | .002 a |
Peroneal terminal latency (ms) | 3.50 ± 0.83 | 3.92 ± 1.03 | 3.91 ± 1.06 | .07 |
Peroneal velocity (m/s) | 45.1 ± 5.3 | 42.4 ± 5.5 | 41.5 ± 5.8 | .007 a |
Peroneal F-wave | 48.7 ± 5.1 | 50.9 ± 6.4 | 53.1 ± 7.6 | .624 |
Posterior tibial latency (ms) | 3.59 ± 0.81 | 3.74 ± 0.71 | 3.69 ± 0.88 | .028 a |
Posterior tibial velocity (m/s) | 43.1 ± 5.5 | 41.4 ± 5.9 | 41.1 ± 4.8 | .176 |
Posterior tibial F-wave | 49.7 ± 5.8 | 51.1 ± 6.1 | 52.0 ± 5.8 | <.0001 a |
Sural sensory velocity (m/s) | 39.5 ± 3.8 | 35.9 ± 4.3 | 36.3 ± 4.5 | <.0001 a |
Of the 160 patients, 96 (60%) were classified as no neuropathy, 21 (13%) as probable neuropathy, and 43 (27%) as definite neuropathy ( Table 3 ). As GC-IPL thickness showed significant thinning with age (R value = −0.600, P < .001), an age-adjusted analysis of covariance (ANCOVA) method was applied. Age, past medical history, and anthropometric data were not significantly different among the 3 groups. GC-IPL thickness and peripheral nerve conduction values differed significantly between the 3 peripheral neuropathy groups ( P = .002), but the retinal thickness of the parafoveal area, within 1–3 mm, showed no significant difference among the 3 groups ( P = .873). Of the 160 patients, 42 (26%) were classified as no/mild autonomic dysfunction, 69 (43%) as moderate autonomic dysfunction, and 49 (31%) as severe autonomic dysfunction ( Table 4 ). GC-IPL thickness also showed significant difference between the 3 autonomic nerve function groups ( P = .005), whereas retinal thickness of parafoveal area, within 1–3 mm, was not significantly different ( P = .674).
Additionally, factorial 2-way ANOVA was performed to measure the effect of DPN and CAN grade on GC-IPL thickness simultaneously. The result revealed both significant main effect of DPN grade (F = 30.11, P < .001) and CAN grade (F = 4.183, P = .017) on GC-IPL thickness and the interaction effect was shown to be insignificant (F = 0.72, P = .58).
Analysis of GC-IPL thickness and each of the peripheral nerve velocities of the peroneal motor, posterior tibial motor, and sural sensory nerve indicated significant positive correlations between GC-IPL thickness and all 3 peripheral nerve velocities (R values were 0.330, 0.298, and 0.292, respectively, Figure 3 ).
