7 Regenerative Therapies for Sensorineural Hearing Loss: Current Research Implications for Future Treatment
Introduction
Hearing impairment is one of the most common maladies affecting older adults. Almost two thirds of individuals 70 years of age and older have some level of hearing loss.1 The prevalence of hearing loss increases over time and is generally associated with aging; however, it is often untreated.2 Hearing loss can cause difficulty communicating with others, localizing sound, and perceiving warnings, all contributing to a poorer quality of life.3 The National Institute on Deafness and Other Communication Disorders estimates there are nearly 36 million adults in the United States with some degree of hearing loss, most cases of which are caused by the loss of cochlear sensory hair cells.4 Cochlear hair cells are highly specialized mechanosensory receptors that are responsible for converting mechanical sound information into an electrical signal, amplifying it and transmitting it to the brain via auditory nerve fibers. In humans and other higher vertebrates, the inability of cochlear hair cells to regenerate after damage is the primary reason for the permanence of hearing loss. This chapter describes the multiple approaches being taken in pursuit of novel regenerative treatments for sensorineural hearing loss in addition to discussing the most critical challenges in the field.
Background
The organ of Corti is highly organized and consists of many cell types, including cochlear hair cells, supporting cells, and auditory nerve fibers (Fig. 7.1). At birth, the human cochlea contains ~ 15,000 sensory hair cells. There are two types of sensory hair cells—inner hair cells and outer hair cells—both of which are important for hearing. Inner hair cells are responsible for converting sound information into an electrical signal, whereas outer hair cells are responsible for amplifying the signal. Auditory nerve fibers are responsible for sending sound information from cochlear hair cells to the brain for processing. Loss or damage of cochlear hair cells and auditory nerve fibers has been estimated to account for ~ 80% of cases of hearing loss.5
Fig. 7.1 The organization of the organ of Corti. Outer hair cells and inner hair cells are arranged in a 3:1 ratio surrounded by supporting cells. Stereocilia on the apical portion of the hair cells are embedded in the tectorial membrane. Outer and inner hair cells are properly innervated at the base and attach to auditory nerve fibers, which send the auditory signal to the brain for processing.
Hair Cell Regeneration
In mammals, it is known that skin and bone marrow cells are continually replenished throughout life.6,7 Furthermore, taste buds and olfactory bulb interneurons are constantly renewed in adult mammals8–11; however, such regeneration is not seen in the mammalian cochlea. It was previously thought that auditory hair cell regeneration did not occur in any context until 1988 when investigators found functional recovery of hearing due to regenerated hair cells after noise trauma in birds.12–14 The mammalian inner ear was thought to lack this regenerative capacity to replace damaged hair cells until 1993, when investigators found evidence of hair cell regeneration in the vestibular sensory epithelia of adult mammals.15,16 Although replacement of vestibular hair cells was observed, the newly regenerated cells occurred infrequently, and the amount of functional recovery that the regenerated cells may produce has been questioned.17
In the avian inner ear, hair cell regeneration begins after an auditory insult. Subsequent signaling after the insult begins a process whereby supporting cells divide and differentiate into immature hair cells and supporting cells.18–20 Alternatively, supporting cells may transform directly into immature hair cells,19,21,22 a process referred to as transdifferentiation. Using molecular, genetic and environmental cues, these immature hair cells continue to become morphologically distinct as they mature over the course of several weeks.23,24 When compared with that of birds, fish, and amphibians, the cochlear sensory epithelium in mammals appears to have lost its ability to regenerate after hair cell loss. Exactly why this loss of regenerative ability has occurred with evolution remains unclear. Regardless, many investigators have focused attention on using what is known of mammalian hair cell development to guide efforts focused on regenerating hair cells after loss.
Treatments based on regenerating lost or damaged tissue are inevitably complex, and efforts to regenerate hair cells in the mammalian cochlea are subject to unique challenges beyond those seen in other organs. First, one needs to consider the complexity of an auditory hair cell. Hair cells are morphologically distinct, with a round base and thinner apex. They contain small hair cell-like bundles called stereocilia, which extend from the apex of the cell and are embedded in the tectorial membrane. These hair cell bundles are mechanosensitive, responding to ionic flow, which transforms sound vibrations into electrical impulses. The electrical signal is then sent to the brain via auditory nerve fibers for further processing. Because of this, proper neural integration needs to be established to transmit sound information to the brain. Moreover, the cochlea has a distinct cytoarchitecture that is highly organized. This cytoarchitectural organization of the cochlea is critical to its proper function. Newly generated hair cells would need to integrate in the proper location within the cochlea and furthermore within the auditory sensory epithelium (the organ of Corti) to encode sound information accurately. Last, the cochlea is a delicate, membranous, and fluid-filled structure that is surrounded by dense otic capsular bone, making surgical access to the organ of Corti challenging. Efforts to outline meaningful regenerative therapies for hearing loss in humans need to address these unique challenges, and numerous laboratories worldwide are actively engaged in this exciting area of research.
Mechanisms for Avian Hair Cell Regeneration
Currently, there are two proposed mechanisms for cochlear hair cell regeneration in the avian inner ear: supporting cell proliferation and transdifferentiation. Cell proliferation is referred to as the growth and division of cells, during which a supporting cell reenters the cell cycle giving rise to two daughter cells that differentiate into one supporting cell and one hair cell. When cochlear hair cells are destroyed, they send a signal to neighboring supporting cells to activate proliferation.18,25 This signal prompts supporting cells to migrate through the sensory epithelium, reenter the cell cycle, and generate a daughter hair cell and a supporting cell.15 Alternatively, hair cells may be generated via supporting cell transdifferentiation, during which a differentiated cell is transformed into another cell type without cell cycle reentry.26 In this approach, neighboring supporting cells are converted to hair cells via nonmitotic mechanisms with consequent depletion of the supporting cell population.
As applied to the mammalian inner ear, it is not clear how these mechanisms of regeneration might affect the organization, structure, and functional integrity of the organ of Corti.27,28 If supporting cells don’t replace themselves as they transdifferentiate into hair cells, the cytoarchitecture and function of the organ of Corti might be compromised. As such, a method to regenerate hair cells in the mammalian inner ear that does not lead to depletion of the cohort of endogenous supporting cells would seem logically preferable to one that relies on transdifferentiation alone. It is possible, however, that the mammalian inner ear has some tolerance to a level of depletion of the supporting cell population if it provides a healthy, functional cohort of hair cells. Ongoing research in this area through the approaches described here will likely provide insight into these unanswered questions in the years to come.
Regenerative Approaches for the Treatment of Hearing Loss
There are several approaches being taken in the pursuit of novel, regenerative treatments for hearing loss, each having unique potential benefits and challenges. These approaches can generally be grouped into four categories:
1. Gene transfer
2. Pharmacotherapies
3. Exogenous delivery of stem cells
4. Promotion of endogenous stem cells
Gene Transfer
Gene transfer has become an attractive avenue for regenerating hair cells by introducing a gene of interest to cells. To date, several studies expressing various genes of interest have produced promising results, which are discussed next.
Atoh1
The expression of the basic helix-loop-helix transcription factor Atoh1 is one of the first indicators of hair cell differentiation in the cochlea.29–32 In developing mammals, Atoh1 is expressed in prosensory patches that give rise to the auditory sensory epithelia and is both necessary and sufficient for hair cell development and formation.31–33 Mice without the Atoh1 gene lack sensory hair cells in the auditory and vestibular portions of the inner ear.29,31,32 In contrast, when Atoh1 is overexpressed in cultured cochlear explants, supernumerary hair cells are generated.33 Moreover, Atoh1 has been found to be upregulated during hair cell fate specification in the adult chicken during hair cell regeneration.34 Collectively, these findings speak to the critical role that Atoh1 plays in determining hair cell fate specification within the inner ear.
Investigators have introduced Atoh1-expressing viral vectors into the organs of Corti of a variety of different rodent species. Kawamoto and colleagues showed that delivery of Atoh1-expressing adenoviral vectors to the organ of Corti of mature, normal-hearing guinea pigs results in expression of the gene product in the organ of Corti and in some nonsensory locations (ectopic expression in cells outside of the organ of Corti) within the cochleae.35 Cells expressing the exogenously delivered Atoh1 also expressed the hair cell marker myosin VIIa and displayed immature stereociliary bundles at the apex of the cell. In addition, these newly formed hair cells appeared to attract axons extended from the auditory nerve on some level. From this, the authors of this study concluded that cells in the normal-hearing adult mammalian inner ear are capable of generating new hair cells upon Atoh1 misexpression.
Taking this a step further, Izumikawa and colleagues delivered an Atoh1-expressing adenovirus to the organs of Corti of deafened mature guinea pigs.36 Animals transfected with the virus showed new hair cell formation in the organ of Corti and in some ectopic locations in the cochlea. The authors also reported a significant improvement in auditory brainstem response thresholds in the ears of animals transfected with Atoh1. Cross-section analysis revealed that some of the cells displayed a mixed phenotype, having both hair cell and supporting cell features. As a whole, the source of the newly generated hair cells was unclear; however, it was hypothesized they arose from transdifferentiated and proliferated cells within the damaged regions that had been transfected with the Atoh1-expressing adenovirus.
In 2008, Gubbels and colleagues established a method to conduct gain-of-function studies in the developing inner ear using an in utero gene transfer technique.37 Plasmids encoding Atoh1 and green fluorescent protein (GFP) were microinjected into the otic vesicle of mice on embryonic day 11.5 and examined on embryonic day 18.5 and later time points. Ears that received Atoh1 demonstrated supernumerary hair cell formation throughout the cochlea. Cells that formed secondary to the delivery of exogenous Atoh1 expressed myosin VIIa and displayed stereociliary bundles. Moreover, they attracted neuronal processes and expressed the ribbon synapse marker carboxy-terminal binding protein 2. Postnatal electrophysiological analysis of the cells generated from in utero transfer of Atoh1 revealed age-appropriate basolateral conductances and mechanoelectrical transduction properties. These results demonstrate that it is possible to generate cochlear hair cells by Atoh1 misexpression after in utero gene transfer. Moreover, this study showed that the generated hair cells are functional on a cellular level and establish connections with the central auditory network.
Collectively, these studies suggest that a gene transfer approach using transcription factors known to be critical for hair cell development can generate hair cells in normal and deafened cochleae of both adult and developing rodents. Furthermore, these studies demonstrate that the newly generated hair cells are able to associate with the nearby auditory nerve and are functional on a cellular and possibly even an organ system level. It remains unclear if this type of approach leads to depletion of the supporting cell population and, if so, its implications. In addition, the long-term viability of hair cells generated through Atoh1 gene transfer is similarly unclear. Regardless, Atoh1 gene transfer–based approaches represent a promising and active area of investigation in the pursuit of novel, regenerative therapies for hearing loss.
Cell Cycle Modulators
Modulating genes that have a role in cell cycle regulation is another molecular approach being pursued to achieve hair cell regeneration. Although mammalian supporting cells are generally quiescent in vivo, several studies have reported that these cells have the capacity to reenter the cell cycle and generate hair cell–like cells in vitro.38–43 The concept of this approach is that by altering the cell cycle of residual supporting cells following hair cell loss, proliferation may ensue, with subsequent differentiation of the progeny into hair and supporting cells.
It is well established that during development, cell cycle exit continues progressively along the cochlear duct from the apex to the base, starting on embryonic day 12 and completing by embryonic day 14 in mice.29,44–46 While this is occurring, cochlear cells begin to express hair cell markers, including Atoh1, myosin VI, and myosin VIIa.29,31,32 At a similar developmental time, supporting cells begin to express the cyclin-dependent kinase inhibitor p27kip1.44,46 The expression of p27kip1 has been shown to coincide with the cell cycle exit of hair cell and supporting cell progenitors.29,44,47 P27kip1 is continually expressed in supporting cells, which may be responsible, to some degree, for maintaining the quiescent state of supporting cells.46 Alternatively, hair cells rapidly downregulate p27kip1 during differentiation, expressing the cyclin-dependent kinase inhibitor p19Ink4d instead,46,48 which is thought to maintain them in a quiescent state (see later discussion).
Modulation of the expression of cell cycle inhibitors, such as p27kip1, may hold promise as a potential means for promoting some level of regeneration of hair cells in the mammalian inner ear. P27kip1 appears to play a significant role in the inability of mammalian hair cells to regenerate after damage.47,49 In the cochlea, P27kip1 is regulated at both transcriptional and posttranscriptional levels.44 Mice deficient for p27kip1 have supernumerary hair cells and supporting cells, most of which are located in the apical region of the cochlea.47 In addition, auditory brainstem response thresholds obtained from these mice were significantly elevated compared with controls, suggesting severe to profound hearing loss. The significant elevation in auditory brainstem response thresholds is thought to occur from excess hair cells and supporting cells disrupting the spatial organization and mechanical properties of the basilar membrane.47,49 In another study, White and colleagues examined the regenerative capacity of supporting cells isolated from the postnatal mouse.41 The authors found that postmitotic supporting cells are capable of transdifferentiating into new hair cells in vitro. In the first experiment, the ability of postmitotic supporting cells to reenter the cell cycle was examined by isolating supporting cells expressing green fluorescent protein under a p27kip1 promoter and culturing them in vitro. After 2 days, 60% of green fluorescent protein (p27kip1) positive cells downregulated expression of p27kip1, whereas 38% of these cells incorporated BrdU, signifying that these cells had reentered the cell cycle. Additional analysis determined that these cells were then able to differentiate into hair cells. A small number of these cells expressed the hair cell marker myosin VI. These results demonstrate that postnatal supporting cells from mice have the ability to divide and differentiate into hair cells via mitotic and nonmitotic means. Collectively, these studies suggest that modulation of p27kip1 in supporting cells may establish a method, or part of a method, to regenerate hair cells following their loss.
Although p27kip1 expression coincides with cell cycle exit, it is not essential for this occurrence.50 This suggests there are other genes that play a more central function in regulating cell cycle exit. Retinoblastoma (RB) 1 is another type of cell cycle regulator, which plays a role in holding inner ear hair cells in a state of quiescence and may represent a potential target for enabling regeneration to occur in the mammalian organ of Corti. The RB1 gene is a tumor suppressor involved in regulating cell cycle exit, differentiation, and survival. Although hair cells in the vestibular and auditory portions of the inner ear generally undergo similar processes during development and differentiation, RB1 appears to play different roles in these regions.51,52 In 2005, Sage and colleagues found that targeted deletion of RB1 in the developing mouse utricle leads to proliferation of vestibular hair cells,53 suggesting a potential role for alteration of RB1 as a means to achieve regeneration of hair cells in the future. More recently, inactivation of RB protein in postmitotic supporting cells resulted in cell cycle reentry, with a subsequent increase in the number of supporting cells in the neonatal mouse cochlea.54 Moreover, some of the nuclei of proliferating supporting cells were intermittently observed in the hair cell layer above their normal position, similar to supporting cells undergoing regeneration in the avian auditory epithelium. There was no evidence of newly generated hair cells from supporting cells, suggesting that there may be a potential role involving other signaling factors to facilitate the continued differentiation of the newly generated cells into hair cells. One concern in the application of this type of strategy lies in the risk of tumor formation with manipulation of tumor suppressor genes such as RB1. As such, additional investigation will be needed to determine if efforts aimed at targeted alteration in the expression or function of genes, such as RB1, could be pursued safely as a potential therapeutic strategy for hair cell regeneration in the future.
Following hair cell formation, a mechanism to maintain the postmitotic status and continued viability of the cells is necessary to prevent their degeneration. The cyclin-dependent kinase inhibitor p19Ink4d is a factor that appears to sustain the postmitotic status of hair cells.48 During development, mice deficient for p19Ink4d develop in a normal manner; however, hair cell loss is observed beginning on postnatal day 17.48 In these mice, it appears that hair cells attempt to reenter the cell cycle, causing them to die by programmed cell death. As already discussed, hair cells rapidly downregulate p27kip1 during differentiation,46 suggesting that p19Ink4d alone is responsible for maintaining the postmitotic state of the hair cells.48 For the purpose of newly generated hair cells, continued maintenance is a constant process, and failure to regulate this accurately can have adverse effects on hearing. Accordingly, future efforts aimed at regenerating hair cells in the deafened cochlea will need to take into account the ongoing need for maintenance of the newly generated hair cells to ensure their permanence.
Collectively, studies evaluating gene transfer–based approaches for the treatment of hearing loss have produced promising results. Additional investigation is necessary to characterize key genes and cell cycle modulators that have been found to be critical for hair cell regeneration in animal and cell culture models. Furthermore, it is essential to determine if direct transdifferentiation of supporting cells into hair cells leads to a depletion of supporting cells, which might disrupt the cytoarchitecture of the organ of Corti. Moreover, the long-term viability of hair cells generated through gene transfer is similarly unclear. In addition, determining a safe and effective method to deliver genetic material into the inner ear remains a critical challenge. It is plausible that genes of interest could be introduced to the inner ear through transtympanic delivery to the middle ear with subsequent transport or diffusion through the round window membrane to the cochlea. Alternatively, direct injection of a gene of interest to the scalar fluids of the inner ear might ultimately be required for meaningful delivery within the cochlea. The ability of the gene to penetrate all areas of the cochlea would need to be determined in addition to ensuring that no further damage results from these delivery approaches to the inner ear. Beyond the challenges of gene delivery on an organ basis, genetic material needs to be transported across the cell membrane for subsequent transcription to take place. Methods to accomplish this include packaging the gene in a viral vector that has tropism for the supporting cells of the organ of Corti, usage of electrical pulsations (electroporation) to drive the genetic material through the cell membrane or potentially packaging the genetic material in a lipid-based carrier that fuses with the cell membrane to release the gene of interest into the cytoplasm. Although these cellular delivery mechanisms are plausible approaches for gene delivery to the inner ear, a viral-based method for gene delivery appears to be the most logical candidate and the approach used in scientific studies on cochlear gene transfer to date.35–37 Although many questions remain to be answered, gene transfer–based approaches for the treatment of hearing loss remain a promising and attractive area of investigation.
Pharmacotherapy
Pharmacotherapeutics focuses on the use of drugs to modulate signaling pathways or gene expression in a cell. In regard to hearing loss, these drugs may target specific cellular pathways that signal supporting cells to divide, or target the regulation of specific genes such as Atoh1 in attempts to generate new hair cells. In concept, these synthetic molecules with biological activities could potentially be given systemically, transtympanically, or by intracochlear administration to effect the generation of hair cells after loss, potentially avoiding some of the challenges associated with other approaches for hair cell regeneration.
The Notch signaling pathway is one potential target for pharmacotherapeutically mediated efforts toward hair cell regeneration. This signaling pathway is responsible for establishing, at least in part, the hair cell–supporting cell mosaic of the organ of Corti during inner ear development.55–60 During development, Notch activation in supporting cells upregulates Hes and Hey transcription factors, which inhibit Atoh1 expression.60
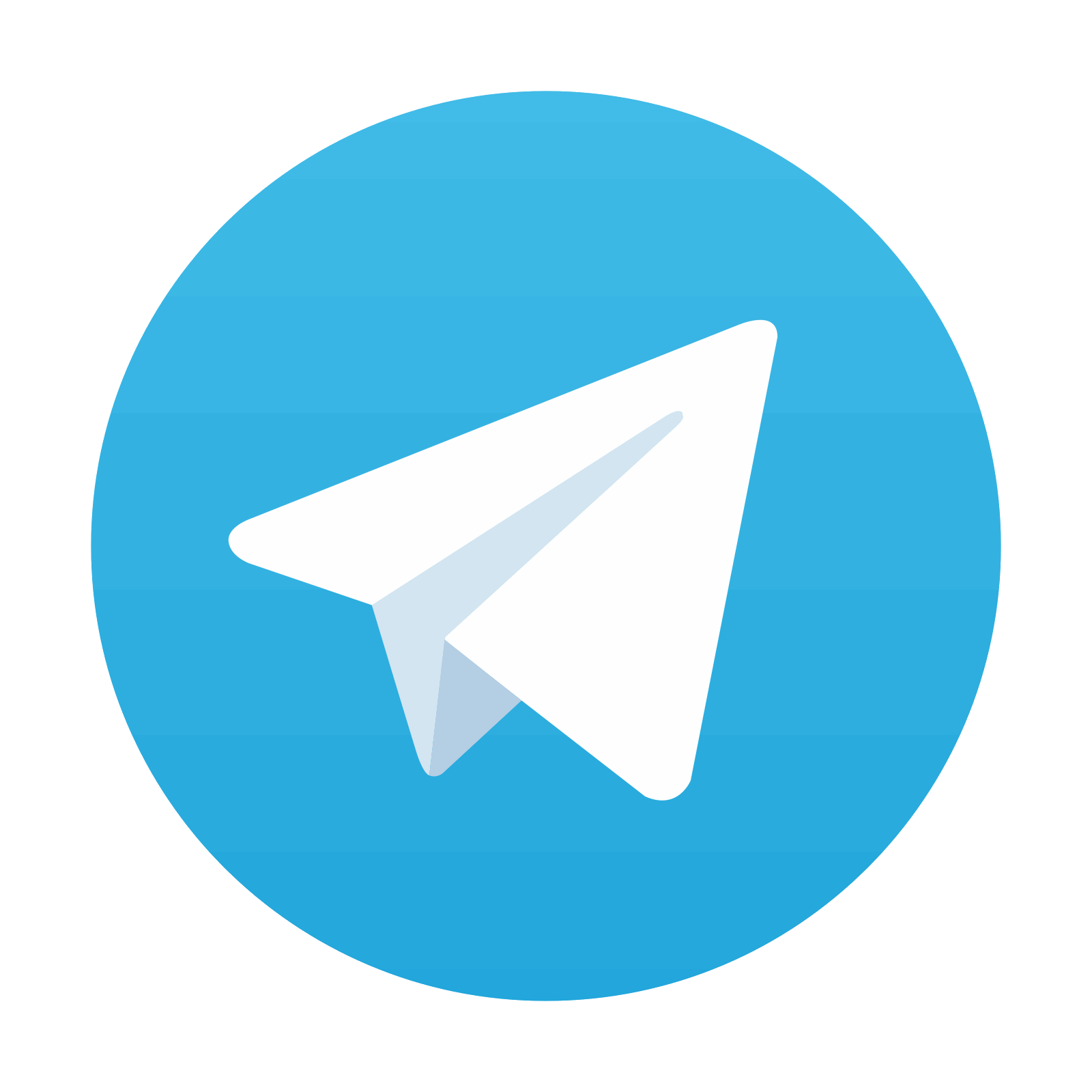
Stay updated, free articles. Join our Telegram channel

Full access? Get Clinical Tree
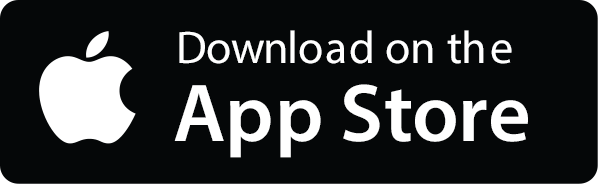
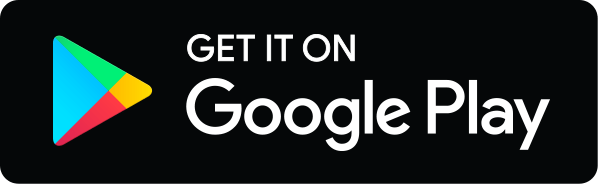