Fig. 15.1
Three elements of tissue engineering. Cells: stem cells (such as ES cells and mesenchymal stem cells) and progenitor cells. Scaffold: natural polymers such as collagen used as artificial extracellular matrix, synthetic polymers such as polyglycolic acid, and inorganic compounds such as hydroxyapatite. Regulatory factors: hepatocyte growth factor (HGF), basic fibroblast growth factor (b-FGF), nerve growth factor (NGF), various interleukins, and interferons
In the original method of tissue engineering, these three elements were placed in a Petri dish to prepare the target tissue and the resulting tissue was implanted into a living body [1]. Absolute requirements for successfully engineered tissue are the establishment and maintenance of a blood supply to the implanted tissue and the survival of the tissue. However, if the tissue is larger than a certain size, a good blood supply is difficult to maintain in the implanted tissue.
A concept called “in situ tissue engineering” (in vivo regeneration) was developed by the Institute for Frontier Medical Sciences at Kyoto University. This concept involved direct placement of these three elements into the living body for regeneration. According to in situ tissue engineering, if cells can be supplied by the surrounding tissue, only addition of scaffold and regulatory factors is needed for cells and blood vessels to migrate from the surrounding tissue into the scaffold. Subsequently, tissue regeneration proceeds as the cells grow in the shape of the scaffold. This method is currently used for clinical application of tracheal regeneration.
15.2 Development of Tracheal Prosthesis
In the 1970s, Professor Grillo showed that tracheal reconstruction can be performed with end-to-end anastomosis if the tracheal defect was 6 cm or less [2]. However, this surgical procedure places a large burden on patients, and the patients must maintain their necks in a flexed position for approximately 1 month after surgery. Various tracheal prostheses were developed to enable tracheal reconstruction without a significant burden on patients with cervical flexion, but many of these efforts were unsuccessful.
In Japan, research on tracheal prosthesis began to progress in 1980. Tracheal prostheses currently in clinical application are prostheses developed using tissue regenerated from autologous cells and subsequently further improved. Figure 15.2 shows the construction of such prosthesis. Its supporting structure is made from a fine Marlex mesh cylinder (BARD mesh), which is reinforced with polypropylene monofilament spiral. The cylinder is then covered with a sponge made of atelocollagen extracted from porcine skin. This atelocollagen sponge has a thin film, multilocular microstructure.
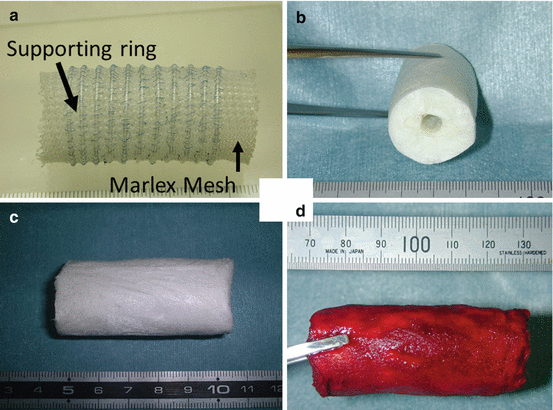
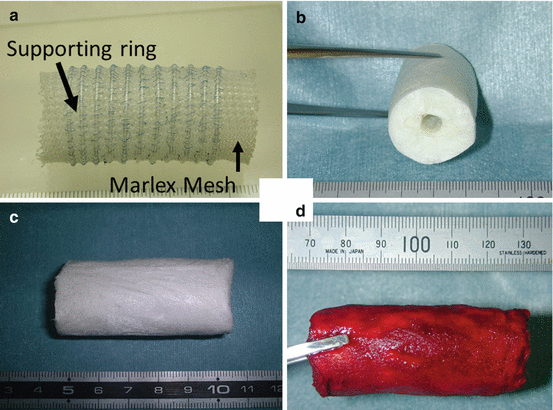
Fig. 15.2
Tracheal prosthesis. (a) Framework of tracheal prosthesis. (b, c) Tracheal prosthesis was composed of a polypropylene frame and a collagen sponge scaffold. The prosthesis can be sterilized as is and stored. (d) The prosthesis was soaked in blood. As a result, the collagen sponge became compressed and the lumen enlarged, resulting in an airtight condition
The trachea has a hollow interior and is supported by cartilage in its walls. The trachea can maintain its shape because cartilage rings give resistance to external compression and negative pressure caused by coughing. Therefore, tracheal regeneration requires scaffold materials with certain strength. Marlex mesh alone does not provide sufficient strength to maintain a patent tracheal lumen. Therefore, polypropylene was used for reinforcement. In our studies, Marlex mesh and polypropylene reinforcement are fusion-bonded by heat and secured together using 7-0 surgical sutures at a 2 mm interval.
Shimizu and Nakamura et al. developed a tracheal prosthesis whose polypropylene monofilament surface was molecularly modified to increase its tissue compatibility. Specifically, they used plasma surface treatment on the prosthesis to create chemically reactive groups to which collagen molecules could covalently bond [3].
The tracheal prosthesis was soaked in blood. Subsequently the remaining tracheal stumps were inserted into the prosthesis and secured using an absorbable suture (Fig. 15.3). In the implanted tracheal prosthesis, fibroblasts migrate from the surrounding tissue into the atelocollagen scaffold. Fibroblasts secrete collagen which replaces atelocollagen, and regeneration of autologous tissue occurs. Regeneration is complete within 1–6 months depending on the length of the defect. Experiments using beagles have shown that tracheal mucosal epithelium and cilia were regenerated along the lumen of the tracheal prosthesis (Figs. 15.3 and 15.4). Tracheas were resected and complete regeneration of the epithelium and cilia was observed even at the tracheal bifurcation (Fig. 15.4). The longest segment of trachea replaced by prosthesis was 9 tracheal rings in length [4, 5].
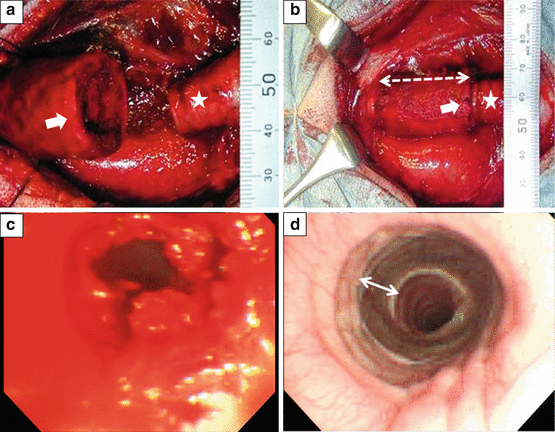
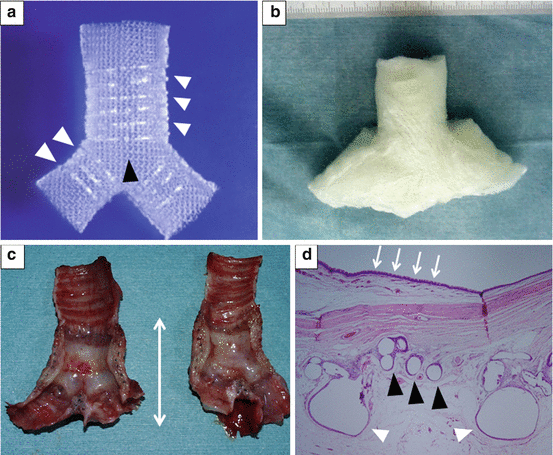
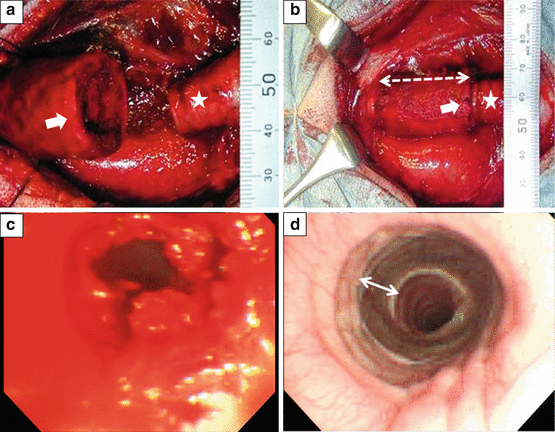
Fig. 15.3
Suture fixation of tracheal prosthesis (dog). Star: Caudal trachea, solid single-headed arrow: end of the tracheal prosthesis, dotted double-headed arrow: tracheal prosthesis, solid double-headed arrow: recipient site of tracheal prosthesis after regeneration. (a) Caudal tracheal prosthesis before suture fixation. (b) The trachea was inserted into the ends of the tracheal prosthesis, and suture fixation was performed. (c) Endoscopic findings of tracheal lumen soon after implantation of tracheal prosthesis. (d) Patency of the tracheal lumen was maintained and epithelialization was complete at 10 months after implantation
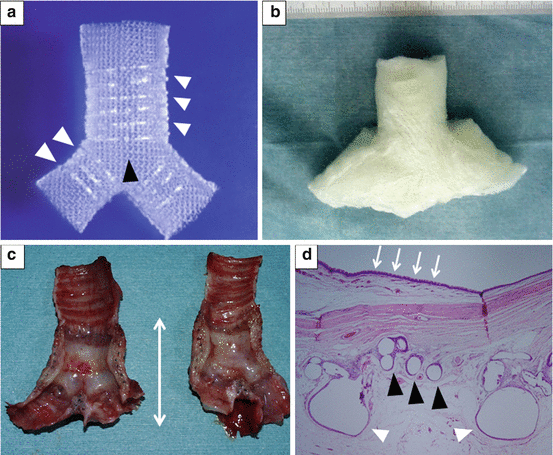
Fig. 15.4
Regeneration at the tracheal bifurcation (dog). White arrowhead: polypropylene reinforced ring, black arrowhead: Marlex mesh, solid double-headed arrow: recipient site of tracheal prosthesis after regeneration, solid single-headed arrow: tracheal epithelium and cilia in the area of regeneration. (a) Framework at the tracheal bifurcation of the prosthesis. (b) Tracheal bifurcation of the prosthesis composed of a polypropylene frame (a) and a collagen sponge scaffold. (c) Cross-section specimen showing the tracheal prosthesis lumen 1 year after implantation. (d) Tissue sample of recipient site of the tracheal prosthesis in another dog (HE staining)
15.3 Clinical Application of the Tracheal Regeneration
A 5-year study on dogs has demonstrated the long-term safety of the tracheal prosthesis made using autologous tissue replacement [6]. Clinical application of this tracheal prosthesis began in 2002 after the review and approval by the ethics committees of the Department of Otolaryngology, Head and Neck Surgery, at Kyoto University Hospital, the Department of Otolaryngology at Fukushima Medical University Hospital, and the Department of Otolaryngology at Kitano Hospital in Osaka. This tracheal prosthesis is presently used for reconstruction of cervical tracheal defects due to tracheal invasion of thyroid cancer and for repair of tracheal stenosis due to various causes [7, 8
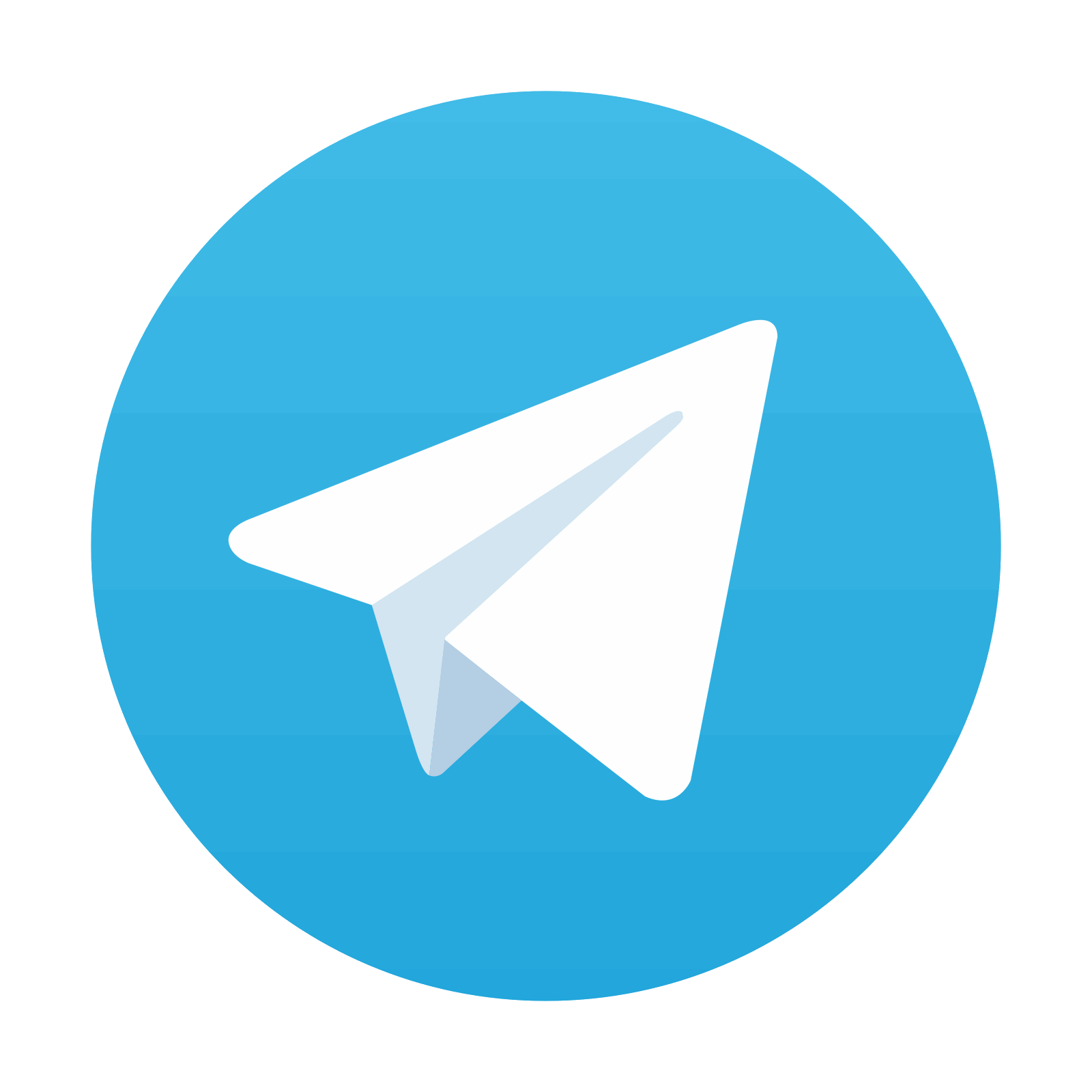
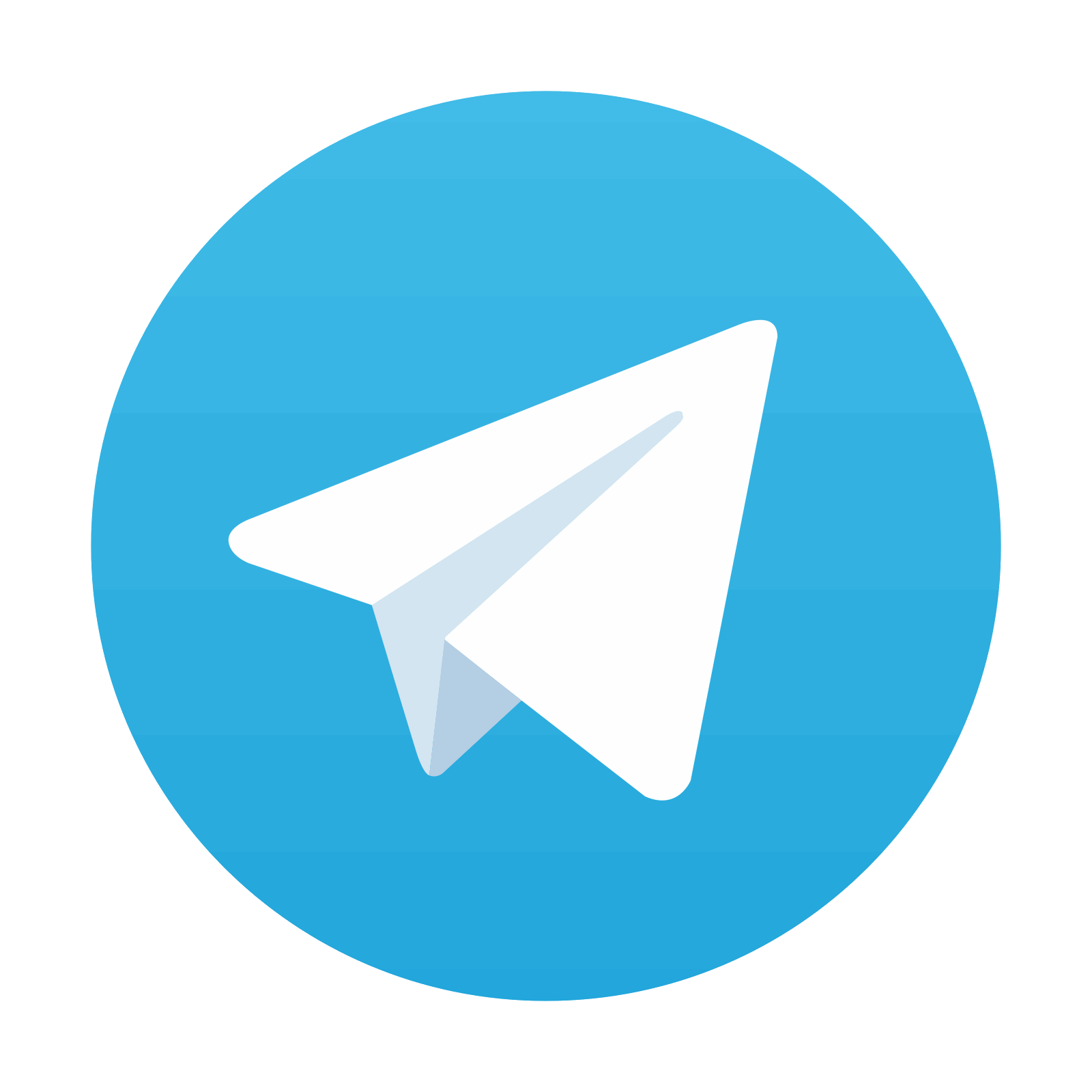
Stay updated, free articles. Join our Telegram channel

Full access? Get Clinical Tree
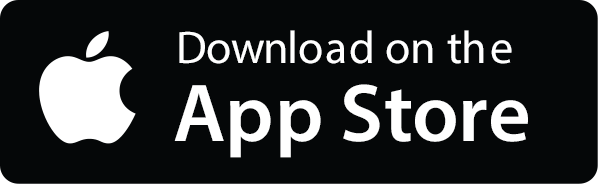
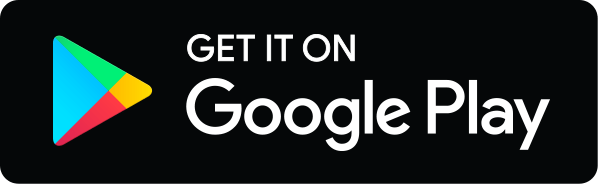