Refractive Error
David E. FitzGerald
The development of the human refractive status from birth to the school-aged child has been the topic of theoretic models and research, particularly over the past 30 years. The resulting literature is voluminous and at times complex; consequently, it can be difficult for one unfamiliar with it to apply the knowledge to actual patient care. This chapter provides an overview of what is known about this dynamic aspect of human development in the interest of optometric patient care.
The concept of an emmetropization process has become the key model in understanding the early development of the human refractive status. In recognition of its importance, this chapter begins with a discussion of the process. This is followed by summarizing reports of the effects of optical interventions on emmetropization. The next section discusses in some detail the refractive status of premature infants, newborns, and provides an overview of refraction from infancy through the preschool years, and finally during the elementary school years. The last section focuses on developmental aspects of myopia, hyperopia, anisometropia, and astigmatism.
Emmetropization
Emmetropization is frequently discussed and infrequently defined by authors, which results in multiple meanings. The following definition is a reasonable standard, however:
“A process presumed to be operative in producing a greater frequency of occurrence of emmetropia and near emmetropia than would be expected in terms of chance distribution, as may be explained by postulating that a mechanism coordinates the formation and development of the various components of the human eye which contribute to the total refractive power” (1).
Thus, it is postulated that the desired result of the process is emmetropia: where parallel rays of light, emanating from an object at optical infinity, come to a point focus or near point on the retina of an eye, which is in a relaxed state of accommodation (2). In clinical terms, emmetropization is a process by which the refractive condition of the eye, regardless of whether it is initially hyperopia, myopia, or astigmatism, centers on a refractive range around low hyperopia to emmetropia (3, 4). Sorsby et al. (3) defined these limits as +0.50 to +1.00 diopter (D) of hyperopia with a standard deviation (SD) of ± 1.00 D.
Some have proposed that emmetropization is a passive process, where nature and genetics are the guiding factors; others have viewed it as an active process, where nurture and the environment are the major influences. Further, evidence indicates that effectiveness of the process depends on four main elements: a healthy eye, a healthy environment, an operational refractive range, and an intact emmetropization mechanism (5,6,7,8,9,10).
Emmetropization as a Passive Process
Emmetropization as a passive process is a school of thought that suggests that nature and genetics are the key factors. Thus, the natural growth and development of most eyes are relatively predictable. Larsen (11,12,13,14), using ultrasound, tracked the changes in ocular growth over the first 13 years of life. Axial length, which was approximately 16.5 mm at birth, increased by 3.8 mm during the first 2 years of life. From 2 to 5 years of age the average increase was 1.2 mm. Most of this growth occurred in the vitreal chamber. Larsen noted that males tended, on average, to have a greater axial length, although at birth there appears to be little difference from females. But by 2 years of age, a differential of 0.3 mm existed, which tended to continue until puberty at which time the differential increased to 0.5 mm (11,12,13,14). During this same period, the crystalline lens flattens, the anterior chamber deepens, and the cornea flattens. All of these changes can contribute to the overall refraction of the eye (11,12,13,14,15,16,17).
The predictable programmed growth gives rise to the passive theory. In addition, genetic tendencies are cited as evidence supporting the passive theory. The likelihood of being myopic is directly correlated to the number of myopic parents. According to Gwiazda et al. (18), a 42% chance of being a myopic offspring exists if both parents are myopic, a 22.5% if one is myopic, and an 8% chance if neither is myopic. Ong and Ciuffreda (19) found a 60% chance of myopia if both parents had myopia, whereas Goss and Jackson (20) note a 57% chance. On the contrary, Zadnik et al. (21) found only a 12% prevalence. In addition, they noted that regardless of the number of parents with myopia, corneal curves were essentially 44.00 D (21). A number of other studies, including those of twins, have supported the hereditary aspects of myopia(22,23,24,25,26).
Emmetropization as an Active Process
Emmetropization as an active process is a theory that prepossesses that emmetropization is mediated by retinal blur (27, 28). The visual system is able to compute the existence of a blur and then respond appropriately to compensate for it. A number of animal studies have been cited in support of the active process theory. Form deprivation and image degradation have been shown to have an intimate positive correlation to eye growth and subsequent refractive status (29, 30).
Animal Studies
Convex and concave lenses, various levels of occlusion, and duration and timing of form deprivation have been methods used to create optical defocus in young primates. The lenses simulate a hyperopic or a myopic refractive condition. This causes the chick or monkey’s eye to alter its anterior-posterior length to maintain image conjugacy. If the causative agent is removed within a specified period of time, the compensating adaptation will respond appropriately to meet the new demand for conjugacy (5,6,8,31,32). Smith et al. (33) note that relatively long periods of form deprivation can be offset by relatively short periods of normal viewing. In another monkey study, Smith and Hung (34) noted a positive correlation between varying degrees of form deprivation and the resulting axial myopia. This “graded phenomenon” can be initiated with lesser amounts of chronic defocus (34).
Human Studies
In humans, form deprivation can be caused by physical insult and substantial defocus. Eyes, which are compromised at birth or before being fully developed, are at risk for physical alteration and reduced function. Pathologic conditions (e.g., ptosis, lid alterations, corneal opacifications, glaucoma, cataracts, aphakia, vitreal abnormalities, retinal anomalies, and retinopathy of prematurity) have been cited in the literature (35,36,37,38,39,40,41,42,43,44,45,46). These compromising conditions frequently result in axial elongation and a relatively more myopic eye. Studies of identical twins with one of their four eyes having cataracts have documented this axial change (32,47,48).
These two seemingly competing theories may not be mutually independent and the emmetropization process may result as a summation and interaction of both. Saunders et al. (49) echo this by stating that the process is a result of the combination of the two theories.
Elements of Emmetropization
For emmetropization to be successfully completed, several prerequisite elements need to be present. Essential are healthy eyes, which are exposed to a visually healthy and stimulating environment. Further, the emmetropization mechanism must be intact, and lastly, the refractive status at particular ages of childhood must be within the limits of the process.
Healthy Eyes and Healthy Environment
Ocular pathologies and anomalies can compromise the eye and its refractive state. In animal studies, the greatest myopic shift occurred with early onset, essentially at birth, lid closure (6,50,51). The duration of occlusion appears to contribute to the gravity of the insult (52). Similarly, the alterations of the visual environment, whether it is done by restrictive stimuli, form deprivation, or optical defocus has a profound impact on the process. In form deprivation (myopia), which is a constant state of defocus, the eye seems to lack the ability to make appropriate ocular adjustments. This results in uncontrolled growth and an increase in refractive abnormalities (9). This process has both a local (eye level) control (39,53,54,55,56,57) and, to a degree, a higher level (brain), for the process is less accurate if the optic nerves are severed or accommodation is blocked (5,31,58). The former seems to have the greater influence on emmetropization, but the latter has been shown in chicks to have some regulating control.
Intact Emmetropization Mechanism
It is uncertain why some patients do not emmetropize or have an inhibitory response to the process. A proposed reason is that the emmetropization mechanism is compromised. In this regard, some predisposing conditions have been suggested.
Patients with amblyopia or strabismus have an altered emmetropization process (59,60,61). Abrahamsson et al. (59) and Lepard (60) found the deviating strabismic eye increased in hyperopia after the onset of the strabismus. An echobiometric (ultrasound) study of patients with strabismic amblyopia revealed ocular growth toward emmetropia in the fixing eye of those with hyperopia and ocular elongation in the nonfixing eye of those with myopia (62). Almeder et al. (63), in a longitudinal study of 686 children ages 3 months to 9 years, then proposed that anisometropia developed slowly and was the result of a microstrabismus. The concept of the existence of some higher level control of emmetropization can also be argued from the fact that patients with compromising mental (not including mental retardation) or neuromuscular conditions tend to have a higher incidence of higher refractive errors than the general population (64,65,66).
Evans et al. (38) suggest that an association exists between defective emmetropization and a faulty visual input or neural transmission. Patients with congenital achromatopsia tend to have higher refractive errors, which do not conform to the concept of emmetropization. In the patient who is achromatopsic, the hyperopia reduces, but the astigmatism noticeably increases. This emmetropization nonconformity would imply the existence of a faulty emmetropization mechanism. Evans (38) proceeds to make a correlation between myopia and early onset image degradation and a delay in retinal function, whereas hyperopia results in the failure of the normal retinal neural processing, such as in achromatopsia, Leber’s amaurosis, albinism, and aniridia (38,67,68). Similar issues can be made about patients with other compromising conditions (e.g., Down syndrome and fragile X syndrome (69,70,71).
Limits of Refraction Status
Aurell and Norrsell (72), in a longitudinal study of children from 6 months to 4 years of age, found that, if a hyperopic refractive error of 4.00 D or more was evident at 6 months of age and strabismus resulted, emmetropization was compromised. Those children in the sample who did not develop a strabismus, however, did emmetropize.
Children whose refraction at 1 year of age was myopic in either meridian by 2.50 D or more tended not to emmetropize, whereas those with a lesser amount did (73). Smith et al. (9) note that the emmetropization process can offset
moderate amounts of defocus; however, they proceed to speculate that a more moderate operational defocus range for emmetropization exists in the monkey than does in the chick. In the Ingram et al. and Atkinson et al. studies (73,74,75,76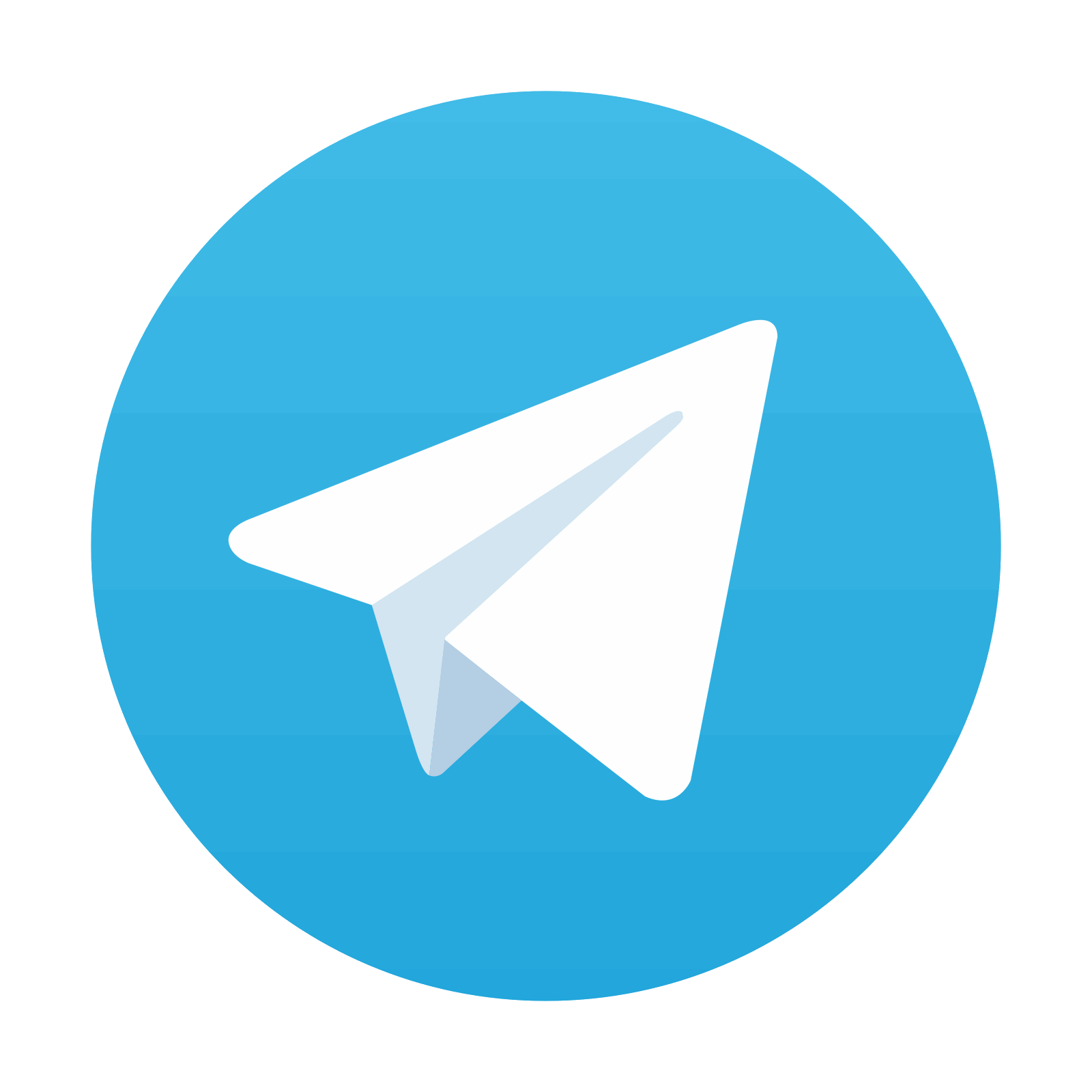
moderate amounts of defocus; however, they proceed to speculate that a more moderate operational defocus range for emmetropization exists in the monkey than does in the chick. In the Ingram et al. and Atkinson et al. studies (73,74,75,76
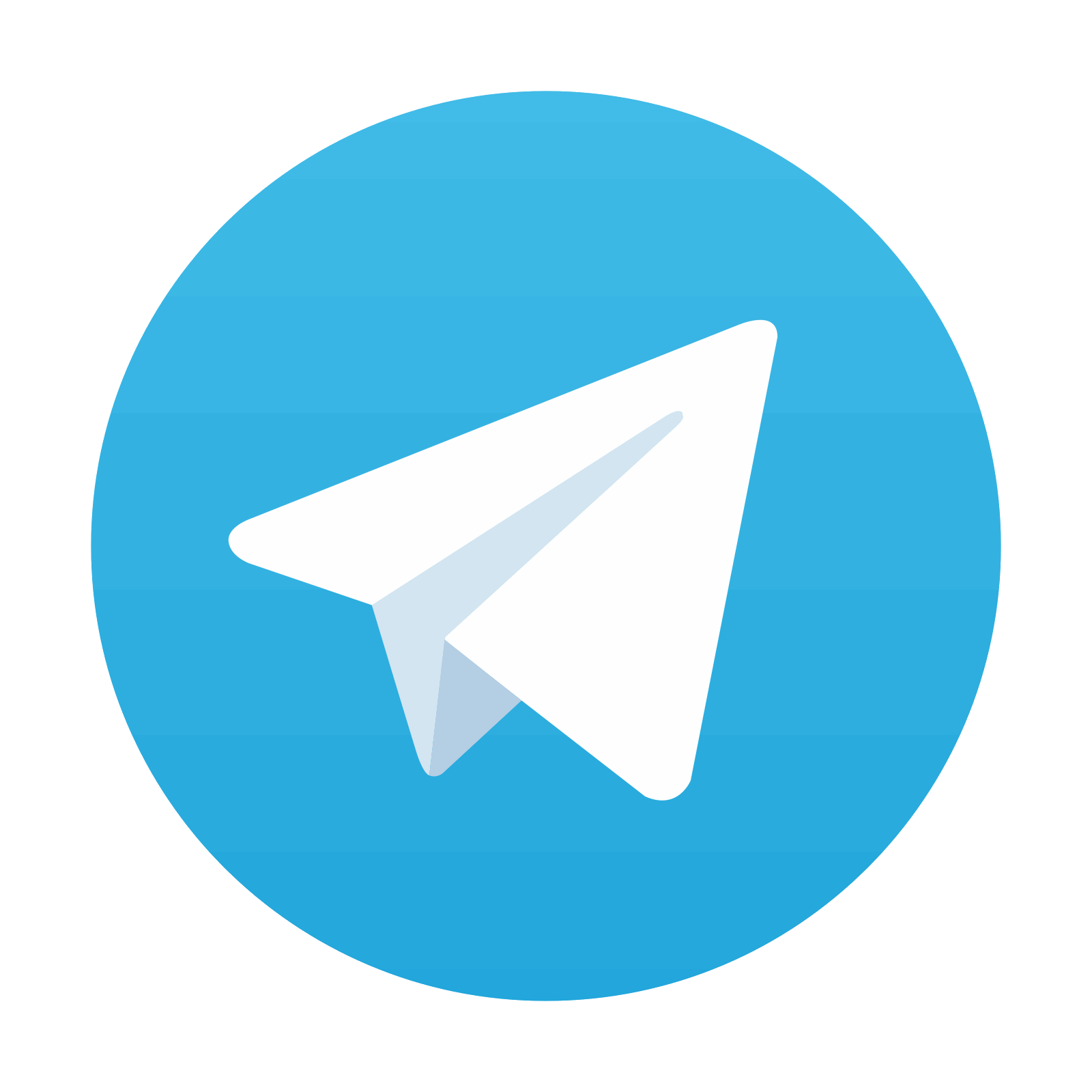
Stay updated, free articles. Join our Telegram channel

Full access? Get Clinical Tree
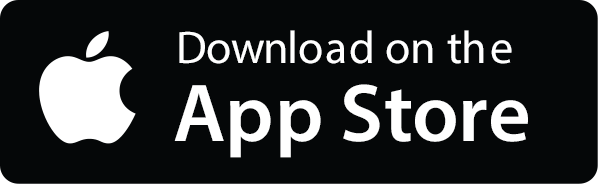
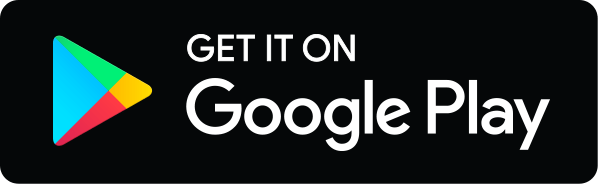
