Development of Eye Movements in Infants
Jordan R. Pola
Over the past 40 years, many studies have investigated both the characteristics and development of oculomotor behavior in infants. These studies have used recording techniques ranging from simple observation of the direction of gaze to objective recording of eye movements in carefully controlled and executed experimental situations. A general finding coming from this work is that, at the time of birth or shortly thereafter, infants make what are typically regarded as immature eye movements, although in some important respects these eye movements are similar to adult movements. Within as little as a few weeks to several months, depending on the particular oculomotor system, the eye movements change to become virtually identical to adult movements.
Concurrent with the study of infant eye movements, the neural mechanisms underlying the generation of oculomotor behavior have been vigorously investigated in both humans and animals. This work has uncovered basic aspects of neurophysiologic activity associated with the generation of eye movements, and the functional significance of this activity as represented, in particular, by models of the oculomotor system. Especially well understood are the features of motoneuron signals and the manner in which they produce efficient eye movements in the face of the resistive mechanics of the extraocular muscles and surrounding orbital tissue (i.e., the oculomotor plant). An important general finding is that along with brainstem motor areas, a broad range of cortical and subcortical areas discharge in association with eye movements, whether fast or slow.
A primary purpose of this chapter is to examine some of the main features of infant eye movements and their development as well as to attempt to relate these features, at least in a first-order manner, to some of the known functional properties of the oculomotor system. The review of the each type of eye movement is divided into three parts: basic features of the eye movement in adults along with a simple explanatory model of the movement; characteristics and development of the eye movement in infants; and mechanisms and processes that may underlie the infant eye movement. An outline of experimental procedures is often provided to explain how the resulting oculomotor data and conclusions were obtained.
Saccadic Eye Movements
Basic Features of Saccadic Movements in Adults
Saccades are high-velocity eye movements that shift the direction of gaze from one spatial location to another. These movements enable us to quickly bring the fovea to a new object so that the object can be seen with maximal clarity. Saccades are utilized in a wide variety of circumstances. We make voluntary saccades to look at an object (or event) that seems to be of
immediate interest or importance. Saccades also occur reflexively in the direction of an event that occurs suddenly and without warning. We typically make a repeated sequence of saccades when we enter a room, are engaged in social interaction, view some panoramic scene, or simply examine a picture. This type of behavior, sometimes called a saccadic scan-path, allows us to rapidly acquire information about the characteristics of the physical or social circumstance. Saccades are often of relatively large magnitude, ranging from 2° to as much as 40°, in everyday circumstances. Small amplitude saccades, however, play an important role in a number of situations. Reading involves a sequence of small saccades as the eyes move along a line of text. When we look at a stationary target, we make microsaccades as part of the process of holding our direction of gaze on the target.
immediate interest or importance. Saccades also occur reflexively in the direction of an event that occurs suddenly and without warning. We typically make a repeated sequence of saccades when we enter a room, are engaged in social interaction, view some panoramic scene, or simply examine a picture. This type of behavior, sometimes called a saccadic scan-path, allows us to rapidly acquire information about the characteristics of the physical or social circumstance. Saccades are often of relatively large magnitude, ranging from 2° to as much as 40°, in everyday circumstances. Small amplitude saccades, however, play an important role in a number of situations. Reading involves a sequence of small saccades as the eyes move along a line of text. When we look at a stationary target, we make microsaccades as part of the process of holding our direction of gaze on the target.
The latency for a saccade is traditionally given as 200 milliseconds (ms). This reflects the time it takes for the following series of events to occur: (a) the passage of visual signals to oculomotor areas of the brain; (b) the processing of oculomotor signals; and (c) the flow of oculomotor signals to the extraocular muscles. Nevertheless, substantial variation exists in the latency from one person to another, especially depending on the specifics of the stimulus situation. Consider, for example, an experiment where a subject initially views a fixation target, and then, on the appearance of a peripheral target, makes a saccade as quickly as possible to look at the target. Typically, the latency of the saccade is shorter if the fixation target disappears before the peripheral target appears (the “gap” condition), than if the fixation target disappears after the peripheral target appears (the “overlap” condition). One interpretation of this difference is that, in the gap condition, an attention disengagement process occurs automatically and quickly with the disappearance of the fixation target, whereas in the overlap condition, the attention disengagement process is slowed by the continued presence of the fixation target.
Saccadic eye movements are among the fastest of human motor behaviors, in some cases reaching a peak velocity of more than 800°/second (s). An important feature of these movements is that, in most situations, they have rather invariant response characteristics: Their duration increases linearly and their peak velocity increases exponentially as a function of amplitude. This stereotypic response shows that, although we often have a choice whether to make a saccade or not, once that choice is made, the mechanism for generating the movement is automatic and deterministic. For this reason, the saccadic system has traditionally been regarded as being ballistic, in the sense that once it is activated, it typically runs until its task—creating a saccade—is completed.
The saccadic system, for our purposes, can be represented as consisting of a cortical component and a brainstem component (Fig. 5.1). The cortical component might be viewed as being responsible for perceptual information processing (perception of and attention to a target), decision-making about where and when to execute a saccade, and initiating the sequence of events leading up to the occurrence of the saccade. In contrast, the brainstem component consists of those structures that produce and shape the actual motor signals that go to the extraocular muscles.
It is worthwhile, at this point, to consider some main features of the brainstem component, because they are relevant both to the discussion of saccades and to the other types of eye movements that are reviewed below. The brainstem component can be regarded as consisting of a pulse generator and a neural integrator (Fig. 5.1). The pulse-generator creates a pulse of neural activity. The pulse goes to the integrator to produce a step of neural activity, and also travels around the integrator to sum with the step. The resulting pulse-step goes to the extraocular muscles where, filtered through the mechanics of the oculomotor plant, it generates a saccadic eye movement (i.e., the pulse overcomes viscous resistance of the plant to produce the saccade, whereas the step deals with elastic resistance to hold the eye in position after the saccade).
Saccadic Movements in Infants
Saccades to a Peripheral Target
Aslin and Salapatek (1) conducted one of the first systematic studies on saccades in infants. The infants, 1 and 2 months of age, were placed on their backs in a reclining seat and looked upward to view targets reflected in a mirror. Eye
movements were recorded using the electro-oculograph (EOG) technique. The study involved two types of trials, replacement trials and addition trials. In the replacement trials, a fixation target was turned off at the same time as a peripheral target appeared, whereas in the addition trials, the fixation target remained on when the peripheral target became visible. An interesting finding was that the infants often made an initial saccade in the wrong direction (i.e., the saccade occurred away from the location of the peripheral target) in both types of trials, but the probability of a saccade in the wrong direction occurred much less in the replacement trials than in the addition trials (Fig. 5.2). The likelihood of a saccade in the wrong direction increased as the distance of the peripheral target increased.
movements were recorded using the electro-oculograph (EOG) technique. The study involved two types of trials, replacement trials and addition trials. In the replacement trials, a fixation target was turned off at the same time as a peripheral target appeared, whereas in the addition trials, the fixation target remained on when the peripheral target became visible. An interesting finding was that the infants often made an initial saccade in the wrong direction (i.e., the saccade occurred away from the location of the peripheral target) in both types of trials, but the probability of a saccade in the wrong direction occurred much less in the replacement trials than in the addition trials (Fig. 5.2). The likelihood of a saccade in the wrong direction increased as the distance of the peripheral target increased.
Perhaps the most striking result of this study is that when infants turned their eyes toward the location of the peripheral target, their eye movements often consisted of a succession of saccades (as opposed to a single saccade as made by an adult). The number of saccades ranged from one to five, with the likelihood of multiple saccades increasing as the eccentricity of the peripheral target increased (Fig. 5.3). All the successive saccades tended to be of about the same amplitude, where the size of each saccade increased as the peripheral target eccentricity increased. The latency of the first saccade was much longer than the usual 200 ms. The latency for 1-month-old infants was 800, 1320, and 1480 ms when the peripheral target was located at 10°, 20°, and 30°, respectively. Furthermore, the intersaccadic latency varied from about 500 to 900 ms.
As would be expected, the percentage of successive saccades decreased over time, changing from 86% in infants 1 month of age to 75% in those 2 months of age (1), and from 55% in those 3 months of age to about 32% in infants 6 months of age (2). An important feature of these saccades is that their velocity–amplitude relation was similar to that for adults (2).
Saccades for Scanning Shapes and Patterns
Looking at a simple peripheral target is a far cry from viewing the complex stimuli that are present in the everyday visual environment. In experiments by Hainline et al. (3), infants
visually scanned either geometrical shapes or simple patterns, a task that might be considered a bit closer to normal visual circumstances. The experimenter held the infants, ranging in age from 14 to 151 days, so that they could view the stimuli over the experimenter’s shoulder. Eye movements were recorded using an infrared corneal reflection technique. In contrast to studies with a peripheral target, infants did not make a succession of saccades from one location to another when viewing either geometrical shapes or simple patterns. Instead, as with adults, they shifted their gaze from place to place using single saccades with intersaccadic intervals of 250 ms or less. Another difference was that instead of saccades of the same size, saccadic amplitude ranged from about 2° to 20°. The dynamics of the eye movements, however, were not the same with the patterns and the shapes. In particular, the saccadic peak velocity was similar for infants and adults with the patterns, but was much lower for infants with the shapes (Fig. 5.4).
visually scanned either geometrical shapes or simple patterns, a task that might be considered a bit closer to normal visual circumstances. The experimenter held the infants, ranging in age from 14 to 151 days, so that they could view the stimuli over the experimenter’s shoulder. Eye movements were recorded using an infrared corneal reflection technique. In contrast to studies with a peripheral target, infants did not make a succession of saccades from one location to another when viewing either geometrical shapes or simple patterns. Instead, as with adults, they shifted their gaze from place to place using single saccades with intersaccadic intervals of 250 ms or less. Another difference was that instead of saccades of the same size, saccadic amplitude ranged from about 2° to 20°. The dynamics of the eye movements, however, were not the same with the patterns and the shapes. In particular, the saccadic peak velocity was similar for infants and adults with the patterns, but was much lower for infants with the shapes (Fig. 5.4).
Saccadic scanning in infants matures rather quickly. Bronson (4,5) showed that when infants viewed simple patterns, the range of saccadic amplitudes changed dramatically between 6 and 13 weeks of age (Fig. 5.5A). The amplitudes varied from about 1° to 5° in 6-week-old infants. They increased substantially, however, by 13 weeks of age, ranging from 1° to 15°. The fixation dwell times between the saccades also changed (Fig. 5.5B). The dwell times varied from 0.1 to 4.0 s in 6-week-old infants, but decreased to no more than about 1.0 s by 13 weeks.
Mechanisms
Infant saccadic responses to a single peripheral target have at least three notable features: the saccadic latency is exceptionally long, an initial
saccade often occurs in the wrong direction, and a succession of saccades occurs when the eyes turn in the correct direction (1). What is responsible for these unusual behaviors? One possibility is that the long saccadic latency is a consequence of an immature attention disengagement mechanism (6). The misdirected saccades and succession of saccades may also be a product of this mechanism. Of course, in addition to, or instead of, undeveloped attention capabilities, infants may not be interested in a small peripheral target situated in an otherwise relatively featureless background. Another possibility is that visual mechanisms for perception of spatial location are not yet developed and, as such, are not easily activated by a simple peripheral target.
saccade often occurs in the wrong direction, and a succession of saccades occurs when the eyes turn in the correct direction (1). What is responsible for these unusual behaviors? One possibility is that the long saccadic latency is a consequence of an immature attention disengagement mechanism (6). The misdirected saccades and succession of saccades may also be a product of this mechanism. Of course, in addition to, or instead of, undeveloped attention capabilities, infants may not be interested in a small peripheral target situated in an otherwise relatively featureless background. Another possibility is that visual mechanisms for perception of spatial location are not yet developed and, as such, are not easily activated by a simple peripheral target.
Infant scanning saccades also have a number of distinctive features. Some of these may arise from the same mechanisms responsible for saccades to a peripheral target. For instance, the long dwell time between saccades when infants scan a simple pattern (4,5) could be the consequence of an immature attention disengagement process. Also, the fact that infants make lower velocity saccades when scanning shapes than when scanning patterns (3) could indicate that infants find the shapes less interesting than the patterns, or it could be a result of poor spatial localization.
The processes underlying attention, perception of location, and volition are primarily located in cortical areas of the brain (Fig. 5.1). This suggests that many of the characteristics of saccades in infants and the ways in which they differ from adult saccades are a result of immature cortical mechanisms. Direct support for a relation between cortical development and the execution of saccades comes from recordings of event-related potentials.
As an example, adults show a positive cortical spike potential preceding the onset of a saccade by about 8 to 12 ms (7,8,9). This potential is thought to arise from the parietal cortex in association with the planning of a saccade. Infants 6 months of age do not show the potential. By 12 months, however,
a positive potential occurs that is smaller but similar in timing to the adult potential (10).
a positive potential occurs that is smaller but similar in timing to the adult potential (10).
In contrast to most features of saccadic eye movements in infants, the velocity of infant saccades is similar to that of adult saccades, both for saccades made to a peripheral target (2) and for saccades made in scanning patterns (3). An implication of this is that the brainstem mechanisms responsible for generating the actual motor behavior (Fig. 5.1) are relatively mature at birth, as opposed to cortical function. That is, the pulse generator, responsible for the high velocity of the saccadic eye movement, and the neural integrator, responsible for holding the eye in its postsaccadic position, appear to be more or less fully functional at birth.
Smooth Pursuit Eye Movements
Basic Features of Smooth Pursuit Movements in Adults
We make smooth pursuit eye movements to track visually a target moving at low to moderate velocity. The primary stimulus for smooth pursuit is generally thought to be target velocity with respect to the retina (i.e., retinal-slip velocity). The pursuit system, however, seems to be able to respond to a variety of stimuli. These include, along with target velocity, target offset from the fovea, target acceleration, perceived target velocity, and possibly relative target-background motion.
![]() Figure 5.6 A model of the smooth pursuit eye movement system. The upper box shows cortical processes for pursuit, and the lower box shows a neural integrator. |
Smooth pursuit movements can occur in response to unidirectional target motion, periodic target motion, or to complex quasirandom or random target motion. Smooth pursuit velocity is typically less than target velocity (i.e., smooth pursuit gain—eye velocity or target velocity is less than 1.0). Furthermore, pursuit gain decreases as target velocity increases or as the target motion becomes more complex. As pursuit gain decreases, saccades tend to occur to reduce excessive error that accumulates between the eye and target (i.e., to keep the fovea close to the target for clear viewing).
The latency of smooth pursuit is about 100 ms, notably shorter than the latency of a saccade. This latency represents the time it takes for visual signals to pass through the visual system to the smooth pursuit motor mechanisms. As in the case of the saccadic system, the smooth pursuit system can be represented as consisting of a cortical component resting atop a brainstem component (Fig. 5.6). The cortical component is involved in perception of target velocity,
attention to the target, initiation of smooth pursuit movements, and maintenance of the pursuit. According to the model, the cortex sends a velocity signal (a step of neural activity) to the brainstem neural integrator to produce an eye position signal (a ramp of neural activity). The velocity and position signals add and are delivered to the oculomotor plant to generate a smooth pursuit movement. As with the saccadic system, the velocity signal is necessary to deal with the viscous resistance of the plant, whereas the position signal is required for the elastic resistance.
attention to the target, initiation of smooth pursuit movements, and maintenance of the pursuit. According to the model, the cortex sends a velocity signal (a step of neural activity) to the brainstem neural integrator to produce an eye position signal (a ramp of neural activity). The velocity and position signals add and are delivered to the oculomotor plant to generate a smooth pursuit movement. As with the saccadic system, the velocity signal is necessary to deal with the viscous resistance of the plant, whereas the position signal is required for the elastic resistance.
Smooth Pursuit Movements in Infants
Smooth Pursuit with Binocular Viewing of a Stimulus
Kremenitzer et al. (11) conducted one of the first quantitative experiments on smooth pursuit eye movements in infants. Newborns (1 to 3 days old) observed a large (12°) black circle moving horizontally at velocities of 9° to 40° -2.6pt/-2pt s. Eye movements were recorded using the EOG. The newborns made occasional “smooth pursuit movement” in the direction of the target motion. The smooth movement eye velocity, however, was low overall and showed only a small increase as target velocity increased (Fig. 5.7). In addition, the duration of smooth movement “epochs” was never more than several hundred milliseconds. One possible problem with this study is that the motion of the large black circle may have activated both a smooth pursuit response, dependent of the fovea, and a component optokinetic response, driven by the peripheral retina (see below: Optokinetic Nystagmus). Aslin (12) attempted to study smooth pursuit without the intrusion of optokinetic movements. He used a smaller target (2° wide, 8° high) that moved in a sinusoidal manner. The results show saccadic tracking of the target with no smooth pursuit in infants 5 to 6 weeks of age, and that smooth pursuit first appeared only after 8 weeks of age.
Both of these studies suggest that smooth pursuit movements are nonexistent or, at best weak, in infants up to several weeks of age. Recent experiments challenge this finding. Perhaps the most detailed investigation of smooth pursuit in infants was conducted by Phillips et al. (13). In this study, infants 1 to 4 months of age, observed a small (1.7°) target moving at a constant velocity. Eye movements were recorded using the EOG. An important finding was that infants as young as 1 month of age were able to make smooth pursuit movements in response to the target motion. The pursuit gain decreased as a function of target velocity (Fig. 5.8A). At higher target velocities, however, the gain increased as the infants became older. As an example, at a target velocity of 24°s, pursuit gain was 0.48 in infants 1 month of age, but increased to 0.77 by 4 months of age. In the case of adults, the gain was approximately 1.0. The duration of smooth pursuit epochs also increased with age (Fig. 5.8B). For example, at a target velocity of 8°s, the duration was about 200 ms in infants 1 month of age, increasing to about 450 ms in those 4 months of age. For adults, the duration was in excess of 1 second. As might be expected, the frequency of catch-up saccades was inversely related to the duration of pursuit epochs. Thus, the number of catch-up saccades rapidly decreased with age, approaching that for adults (Fig. 5.8C). Pursuit latency in infants was remarkably long, varying from 300 to 500 ms, whereas the latency for adults was about 150 ms.
Lengyel et al. (14) conducted a similar study, except that it involved infants less than 1 month of age. The stimulus was a square (9.4°) target moving at 7.50°/s. Smooth pursuit movements occurred in infants as young as 1 week of age. Pursuit gain for these infants was about 0.75 and it remained close to this value up to 16 weeks of age. Pursuit epochs had a duration of between 2 and 3 s in the youngest as well as the oldest infants. This duration, it should be noted, is much longer than that found in the above studies.
Smooth Pursuit with Monocular Viewing
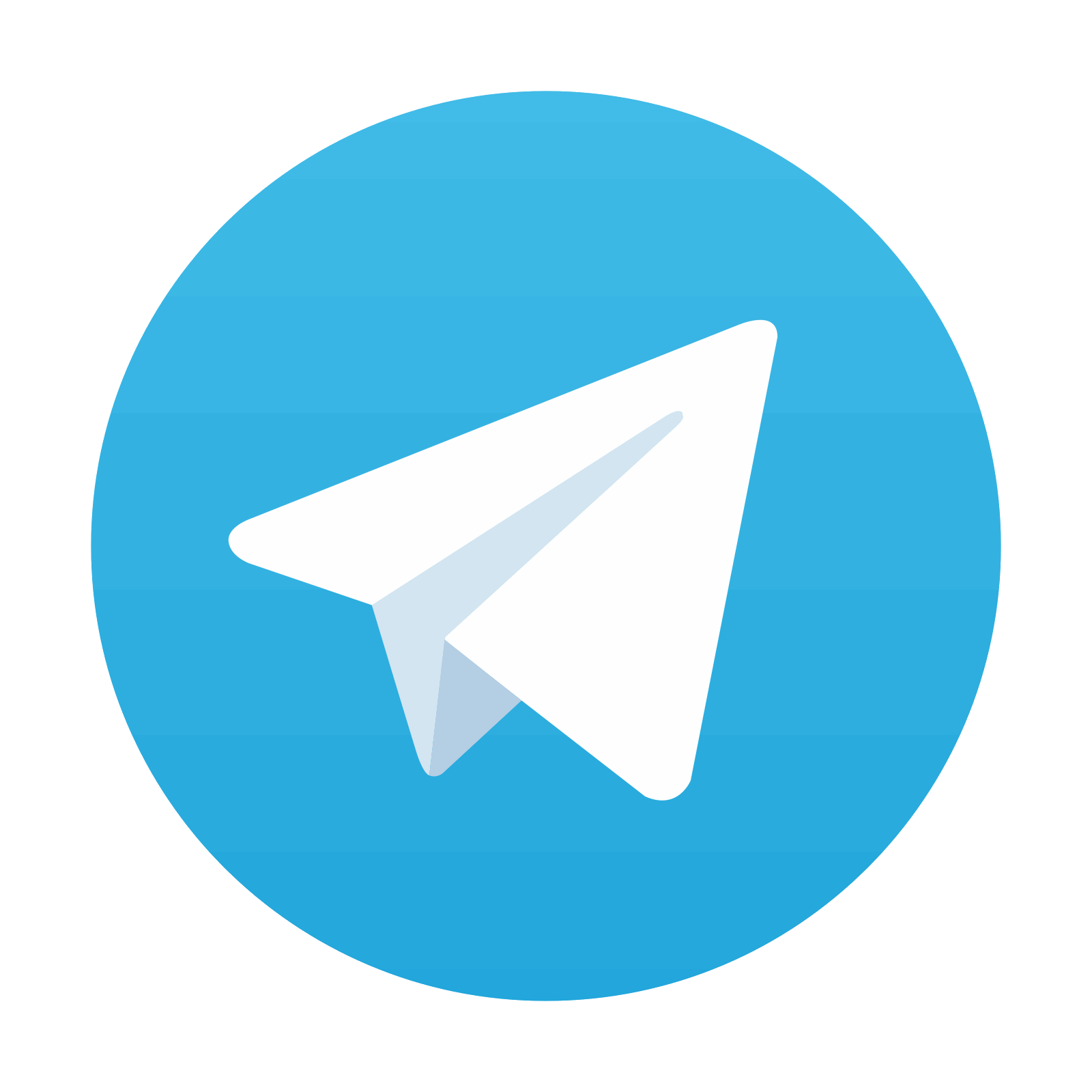
Stay updated, free articles. Join our Telegram channel

Full access? Get Clinical Tree
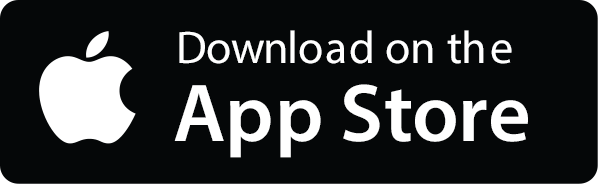
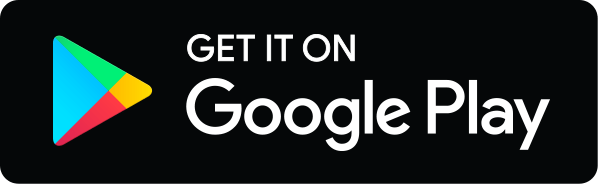
