, Maneli Mozaffarieh1 and Hans Bebie2
(1)
Department of Ophthalmology, University of Basel, Basel, Switzerland
(2)
Institute for Theoretical Physics, University of Bern, Bern, Switzerland
Abstract
The term “oxidation” refers to the loss of electrons and “reduction” refers to the gain of electrons, as shown in Fig. 13.1.
13.1 Redox Chemistry and Terminology
The term “oxidation” refers to the loss of electrons and “reduction” refers to the gain of electrons, as shown in Fig. 13.1.
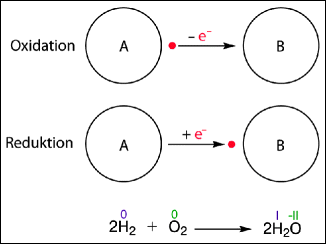
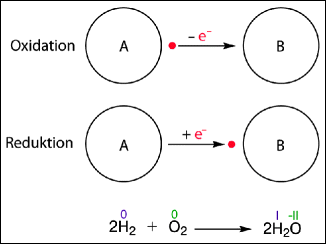
Fig. 13.1
Redox reactions. Oxidation is the loss of electrons by a molecule, atom, or ion. Reduction is the gain of electrons by a molecule, atom, or ion. Substances that have the ability to oxidize other substances are known as oxidizing agents. Substances that have the ability to reduce other substances are known as reducing agents
The oxidation of iron is an example of a redox reaction. When iron is oxidized by oxygen, iron oxide results, which is known as “rust.” An example is the formation of rust in the cornea when a foreign body sticks in the cornea (Fig. 13.2).
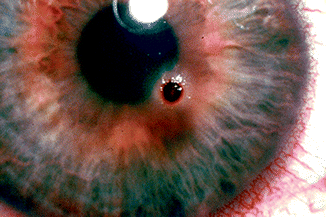
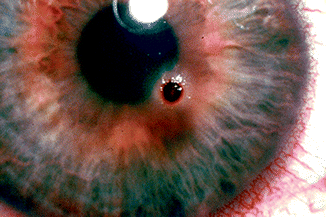
Fig. 13.2
Corneal foreign body. Iron in the cornea forms a rust ring quite quickly. This rust ring, in turn, damages the cornea by facilitating redox reactions
Besides the formation of iron oxide, iron can also be oxidized by transferring electrons to hydrogen peroxide in the so-called Fenton reaction, thereby creating reactive oxygen species (ROS) (Fig. 13.3).


Fig. 13.3
Fenton reaction, which occurs between iron II and hydrogen peroxide in aqueous solutions. Hydrogen peroxide oxidizes iron II (Fe2+) and, in the process, generates the highly reactive hydroxyl radical (OH)
Pro-oxidants are molecules that can oxidize other molecules; in other words, they have an oxidizing potential that is stronger than the oxidizing potential of the molecule they react with. A small but pathophysiologically important subgroup of prooxidants is reactive oxygen species (ROS). ROS shall subsequently be discussed in more detail.
13.2 Production of ROS
ROS are oxygen-containing molecules that are highly reactive. ROS can occur in the form of free radicals (e.g., superoxide anion) or non-radical species (e.g., singlet oxygen). Free radicals are molecules that contain one or more unpaired electrons. The presence of an unpaired electron makes free radicals highly reactive because of the need to pair up the unpaired electron.
The normally occurring oxygen molecule in the atmosphere is relatively inert and called “ground-state oxygen” (Fig. 13.4) due to the fact that the unpaired electrons in the oxygen molecule have the same spin (refer to Chap. 9). Thus, for the oxygen molecule to react, it would need another molecule or atom with two unpaired electrons with a parallel spin opposite to that of the oxygen molecule. This rarely occurs, which explains why oxygen in the ground state is only minimally reactive.
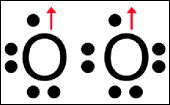
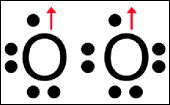
Fig. 13.4
Ground-state oxygen, in which the last two electrons of the oxygen molecule are located in a different π* antibonding orbital. These two unpaired electrons have the same quantum spin number (they have parallel spins) and qualify ground-state oxygen as a diradical
The activation of ground-state oxygen may occur by two different mechanisms: either through the absorption of sufficient energy to reverse the spin on one of the unpaired electrons or through monovalent reduction. If ground-state oxygen absorbs sufficient energy to reverse the spin of one of its unpaired electrons, the two unpaired electrons now have opposite spins (see Sect. 9.5). Due to a change in spin direction, this oxygen molecule, called singlet oxygen (1O2), is much more reactive than ground-state oxygen and reacts destructively, particularly with molecules with double bonds.
The second mechanism of activation is by the stepwise monovalent reduction of oxygen, which gives rise to the superoxide anion radical (O2−) (Fig. 13.5), hydrogen peroxide (H2O2), and, finally, to water (H2O).
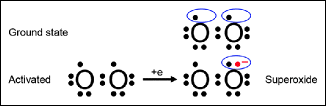
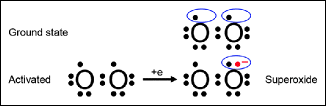
Fig. 13.5
Activation of ground-state oxygen, which can occur by the monovalent reduction of ground-state oxygen to form the more reactive superoxide anion
Both of these mechanisms also occur in our bodies. The production of singlet oxygen is of particular importance in organs exposed to light, such as the eye and the skin, whereas the production of superoxide anion occurs essentially in any tissue. In aerobic cells, energy is gained by oxidizing molecules such as sugar. During this energy metabolism, a certain amount of ROS is produced physiologically as a byproduct. This occurs mainly during electron transfer in the respiratory chain. The tetravalent reduction of molecular oxygen (together with hydrogen) by the mitochondrial electron–transport chain produces water. This system, however, does not always work perfectly and, as a result of electron leakage, the univalent reduction of oxygen molecule forms superoxide anions (O2−). ROS can also be built as intermediates in enzymatic reactions. Certain cell types such as macrophages can produce high amounts of ROS as a part of their defense mechanism (Fig. 13.6).
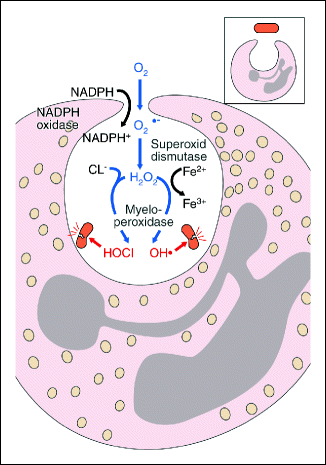
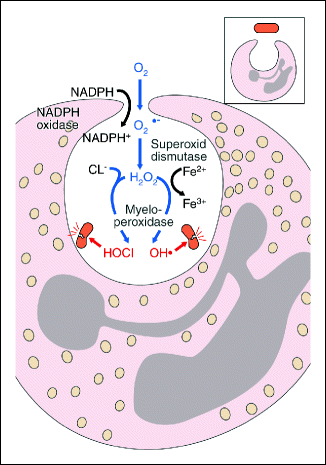
Fig. 13.6
Respiratory burst. The rapid release of reactive oxidative species (ROS) is referred to as “respiratory burst.” Neutrophils have oxygen-dependent mechanisms (myeloperoxidase system) for killing bacteria. After phagocytosis, NADPH oxidase, located in the leukocyte membrane, converts ground-state oxygen into ROS, which, in turn, attacks bacteria
ROS production is obviously not only detrimental but can also be beneficial. It is, for example, a prerequisite to achieve the optimal physical training effect. However, an unwanted increase in ROS occurs in a number of pathologic conditions such as those resulting from an unstable oxygen supply. This is particularly relevant in elderly people, as the capacity to eliminate free radicals decreases with age (Fig. 13.7). Under optimal conditions, the magnitude of ROS formation is balanced by the rate of ROS elimination through the available antioxidants. If the production of ROS exceeds the capacity of its elimination, the amount of ROS increases to a level that leads to oxidative stress. This condition is described in greater detail below.
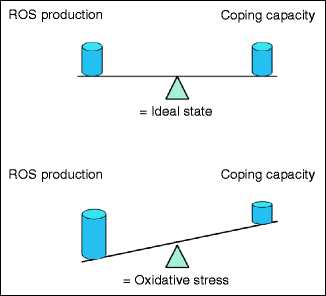
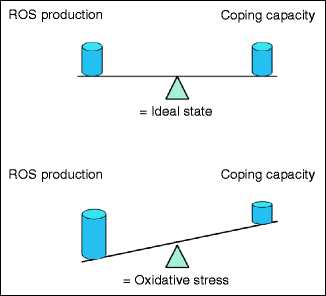
Fig. 13.7
Coping with free radicals. Under optimal conditions, the rate and magnitude of oxidant formation is balanced by the rate of oxidant elimination through the action of antioxidants. If ROS production, however, exceeds this capacity, oxidative stress damages different molecules
13.3 Oxidative Stress
Nature has provided us with mechanisms to cope with ROS with the help of antioxidants. An antioxidant, by definition, is any substance that reduces oxidative damage, such as that caused by free radicals. The antioxidant defense system is comprised of a variety of molecules. These include proteins such as enzymes (e.g., superoxide dismutase and catalase) and low-molecular mass molecules (e.g., some vitamins).
Oxidative stress can damage molecules. One example is lipid degradation initiated by lipid peroxidation (Fig. 13.8). This is a process by which molecular oxygen (O2) is inserted into an unsaturated fatty acid (called a lipid peroxide). This process is initiated by free radicals. The formation of peroxide again leads to the formation of free radicals, inducing a chain reaction.
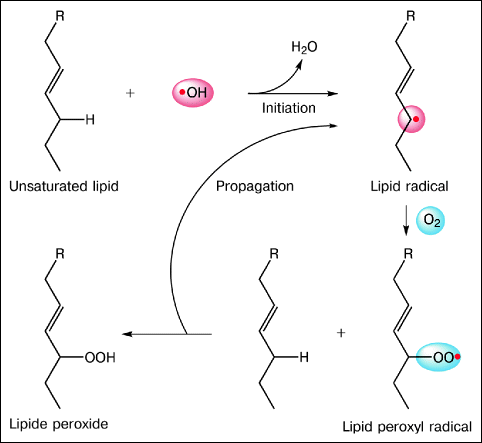
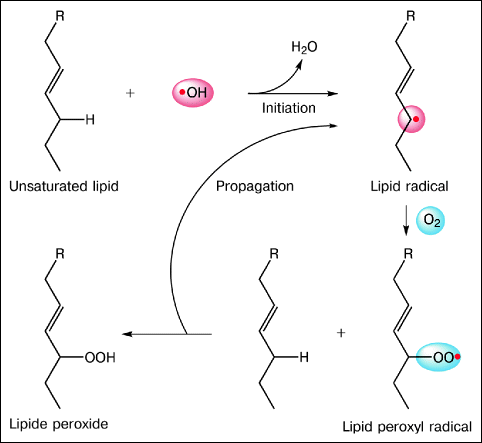
Fig. 13.8
Lipid peroxidation, the oxidative degradation of lipids. It is the process through which free radicals “steal” electrons from the lipids, forming a lipid radical. This lipid radical can then further react with molecular oxygen to form a lipid peroxyl radical, which, in turn, can oxidize other lipid molecules
As long as nature is capable of repairing damaged molecules (e.g., DNA) or eliminating them (e.g., proteins via proteosomes), no major structural damage will occur. However, if oxidative stress exceeds the capacity of antioxidants and the molecular damage exceeds the capacity of repair or elimination mechanisms, structural damage will occur. This results in damage that is ultimately clinically relevant as a basis of diseases (Fig. 13.9). This can occur in any organ, including the eye. In this context, we will focus on eye diseases.
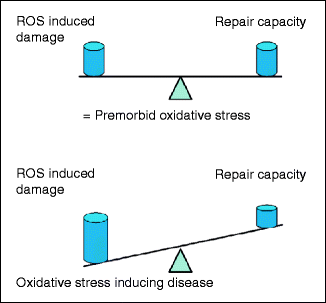
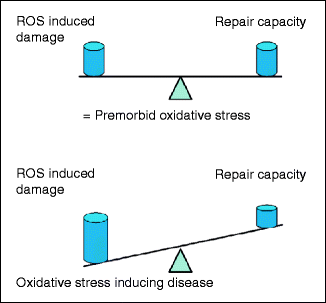
Fig. 13.9
Repair of molecular damage. Top: balance between induced damage and repair capacity. Bottom: imbalance between induced damage and repair capacity
13.4 Oxidative Stress in the Eye
The cornea and the conjunctiva are in direct contact with the ambient air. This has a positive effect on the cornea, as it can receive its oxygen supply from the air, but it also has a negative effect, as the cornea and the conjunctiva are exposed to toxic substances such as ozone in the air. Likewise, the retina requires light for vision but light can also contribute to the production of ROS via photodynamic procedures. We will now discuss some of the consequences of oxidative stress in the eye.
Oxidative stress plays a role in the pathogenesis of cataract formation (Fig. 13.10).
< div class='tao-gold-member'>
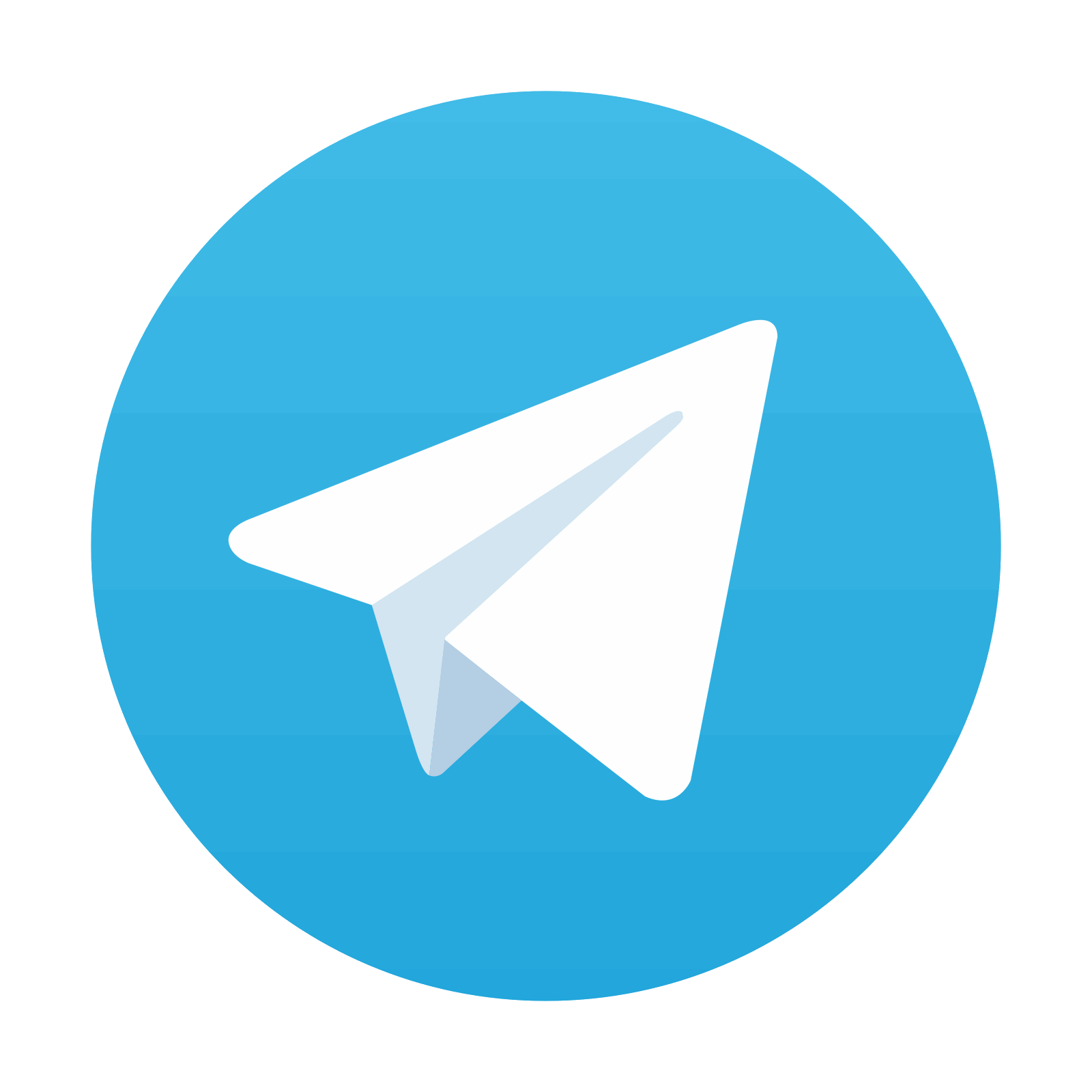
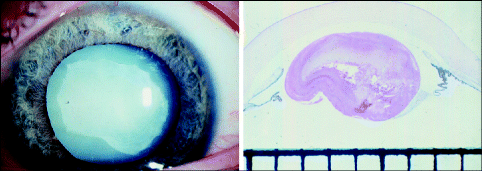
Only gold members can continue reading. Log In or Register a > to continue
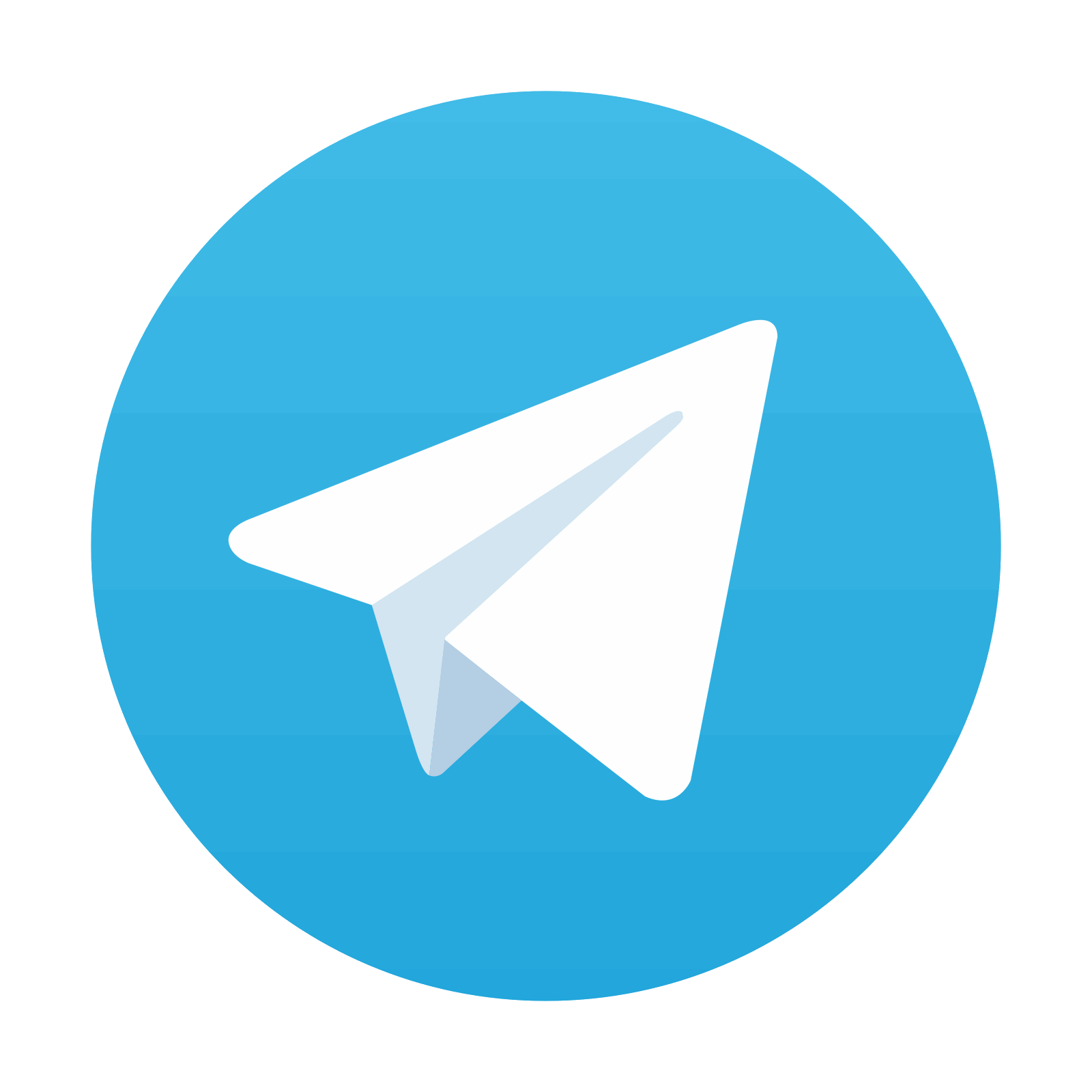
Stay updated, free articles. Join our Telegram channel

Full access? Get Clinical Tree
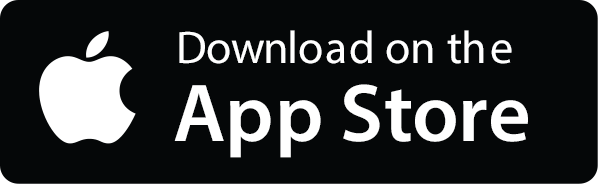
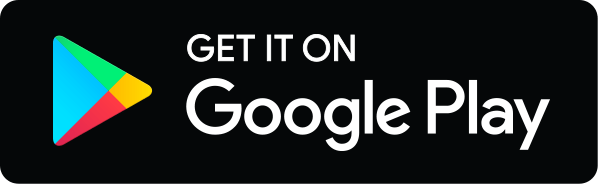