Principles of Orbital Reconstruction
Both congenital and acquired orbital deformities can necessitate reconstructive treatment of the orbit. However, this does not mean that functional impairments, such as diplopia, are always clinically evident. Due to posttraumatic primary treatments of large bony orbital wall defects with bioresorbable materials, for example, a slow volume change of the orbital content can occur although the orbital content itself remains constant. As a consequence, hypoglobus and enophthalmos can occur without apperception of diplopia. This is a typical example of a shift of orbital content developing slowly (over months) that nevertheless, due to the plasticity of the brain, will not necessarily result in functional impairment. On the other hand, when these intraorbital volume shifts take place very rapidly, the consequence is usually an impairment of vision—typically accompanied by diplopia.
From the outset of a chapter on reconstructive orbital surgery it seems reasonable to assume that right and left orbits in the same patient are basically homologous and virtually symmetrical (with the exception of congenital malformations with disturbance of orbital growth and microphthalmos). However, particular pathology in a patient could be a reason not only to try to reestablish this symmetry as an operative aim when contemplating orbital reconstruction procedures, but also to apply corrections according to the volume requirement of the orbit—for example, an extension of the bony orbit (e.g., in cases of Graves’s disease) or reduction of orbital volume (e.g., in posttraumatic enophthalmos) ( ▶ Fig. 19.1).
Fig. 19.1 Sagittal oblique plane image before secondary orbital reconstruction. The primary reconstruction of the orbital floor using absorbable implants had been performed some years before.
The authors of this chapter are not aware of the existence of any considerable orbital deformities that do not have a correlate in a voxel-based dataset. That means that CT scans, MRI scans, and digital volume tomography scans usually show the morphologic correlate of a visible orbital wall deformity, such as hypoglobus, hyperglobus, enophthalmos, or exophthalmos. This is especially evident in posttraumatic or tumor-associated orbital deformities. Orbital volume–associated aspects, such as posttraumatic atrophy of orbital fat tissue, are only mentioned in passing here, with the intention to indicate that they are considered subordinate to the more important issues already mentioned. The authors regard orbital volume–associated aspects not as a primary issue of a volume difference but as a side aspect that should, nevertheless, be included in consideration.
19.3 Summary History of the Development Reconstructive Orbital Surgery
The history of modern orbital surgery is inalienably connected with Paul Tessier (1917–2008), who developed surgical approaches to the orbit along with the understanding necessary for the complex and stable reconstruction of the orbit. Tessier advocated and achieved detailed three-dimensional reconstruction. These ideas were transposed to the present time by various surgeons—Joseph Gruss (Seattle, WA, U.S.A.), and Paul Manson (Baltimore, MA, U.S.A.). Beat Hammer (Aarau, Switzerland) has the reputation of being currently the foremost European pioneer of the concept of modern three-dimensional orbital reconstruction. Hammer took the so called “key areas” of the orbit into consideration for reconstruction and optimized the use of form-stable materials, particularly autogenous bone. 1 Hammer recognized the necessity for and importance of complex reconstruction of the three-dimensionality of the orbit and periorbital structures even before computer-assisted planning was available. His reconstruction concepts have been shown to be feasible and effective even under most difficult circumstances (e.g., post trauma, post radiation therapy, and in congenital malformations), wherein he perfected the reconstruction the bony orbit and periorbital structures. This is demonstrated, for example, by the particular reconstruction of the medial ligament, which he inaugurated. Transnasal medial ligament repositioning and fixation was long the standard procedure for reducing the intercanthal distance. Nowadays this technique has been shown ineffective in cases of complex medial ligament malpositioning, and has been replaced by the so called “Hammer technique,” which allows not only a transversal reduction but also a vertical, and—more importantly—a sagittal repositioning of the medial ligament region, with results that are predictable preoperatively. Hammer’s turn around technique, with particular consideration of structural positioning of the medial ligament, is described in detail later in this chapter.
It was Paul Manson in his important paper “Deep orbital reconstruction for correction of post-traumatic enophthalmos” 2, who pointed out the influence of posttraumatic problems in cases with shape and volume changes of the orbit, and the consequences for the long-term result of enophthalmos, which was then hard to correct ( ▶ Fig. 19.2). His surgical concept involved an extended dissection of the orbit and the reconstruction of the bony orbital walls using bone transplants (calvaria split bone grafts), which were precisely positioned and adapted to the individual patient. In 1989 Joe Gruss published a critical analysis on the results of primary treatment of complex posttraumatic orbital defects using bone transplants. He pointed out that enophthalmos was the most frequent orbital deformity, which in his opinion was essentially attributable to non–form-stable biomaterials used for reconstruction; he related the volume problem of the orbit due to lack of form-stable materials to other problems, such false positioning of intraconal or extraconal adipose tissue, scar formation, or incarceration of the supporting ligament system of the orbit. 3
Fig. 19.2 Cranial view before secondary reconstruction of a right-sided enophthalmos following primary posttraumatic reconstruction using an absorbable implant.
In 2004 Edward Ellis III and E. Messo investigated the usage of nonresorbable alloplastic implant materials for reconstruction of the bony orbital walls. 4 In their validation of solid, nonpermeable alloplastic materials—silicone and Teflon, but also biomaterials—they focused on titanium mesh–based reconstruction materials as the material of first choice for reconstructing the inner orbit. Teflon and Vitallium mesh were reported in the literature as reconstruction material before the currently accepted first choice of titanium grade IV.
One of the first titan mesh–based orbital reconstruction was described by Adrian Sugar (Swansea, Wales, U.K.), 5 who as early as 1991 published the use of titanium mesh for form-stable orbital reconstruction. However, the form specificity of the metallic and other form-stable mesh materials was missing from these early reports. In most cases a non-patient-specific preformed mesh was used, which was adapted to the patient intraoperatively according to the surgeon’s experience. Later Sugar and colleagues developed the idea of patient-specific individualized orbital implants, which were first processed out of cold deformed titanium sheets. Porous polyethylene was also described as a form-stable material. However, like other biomaterials (bioabsorbable materials, fascia, etc.) it has one disadvantage: they all have only minor radiolucency so that these materials are not directly visible in postoperative control CT scans or CBCT datasets, but only indirectly after a time interval by constrasting the paranasal sinuses. 6, 7
19.4 Shape and Volume Reconstruction of the Orbit
The purpose of primary (especially posttraumatic) reconstruction of the orbit is the exact anatomical restoration of shape and volume of the orbit. Any reconstruction procedure, particularly for dimension-relevant orbital wall defects, must have the aim of long-term form stability. Otherwise secondary deformities might occur, which—although sometimes not functionally relevant—are morphologically adverse, and which cannot preserve the stability of the primary reconstruction result. In addition to this, reconstructions in orbital surgery must be visualized, which means that the materials used must have radiopacity. Accordingly it is the authors’ opinion that neither polyethylene-based materials nor polyether ether ketone (PEEK) implants are materials of first choice. 8
▶ Fig. 19.3 shows a typical manifestation of an orbital deformity that occurred several months following primary posttraumatic reconstruction. The material used initially (in this case PDS foil) did not maintain the form stability and revision surgery with secondary orbital reconstruction was required.
Fig. 19.3 Defect of the left orbit before secondary reconstruction. The primary surgical procedure had been performed using a bioabsorbable implant. (a) Sagittal view and (b) multiplanar view of the intraoperative navigation; (c, d) postoperative control visualization of the final secondary reconstruction.
The necessity of a successful primary reconstruction with the aim of restoration of the natural anatomy using form-stable materials emphasizes the importance of a competent primary reconstruction as summarized by Paul Manson: “You never get a second chance.”
The need for reconstruction of the complex three-dimensional shape and volume of the orbit is to provide the ability for quantification of both planning and the intraoperative transformations implemented, and also for postoperative evaluation. Thus three-dimensional imaging with thin slices (CT scans are ideal; axial planes, minimum slice thickness 1 mm, dataset in soft tissue kernel, and saved in DICOM format) is required for preoperative analysis and planning, as well as for postoperative three-dimensional image analysis. This allows evaluation of the morphologic reconstruction of the inner orbital structures.
There are basically two anatomical structures that are important with respect to the evaluation of a deformity and also for evaluation of a reconstruction result: (1) the transition zone between medial orbital wall and orbital floor, and (2) the oblique sagittal plane of the orbit, 8 which is the view in the course of the optic nerve or from the optic nerve canal entrance to the midline of the infraorbital ridge. These structures represent the most important elements and key areas that, according to Hammer, are critical for a successful orbital reconstruction ( ▶ Fig. 19.4, ▶ Fig. 19.5, ▶ Fig. 19.6).
Fig. 19.4 Visualization of the intraoperative navigation of a CAD model (pink) during secondary correction of a malpositioned primary reconstruction of the right-sided orbital floor and median orbital wall region. The initial reconstruction typically differs more from the ideal model the more posteriorly the reconstruction extends into the orbit. (a) Coronal. (b) Sagittal. (c) Axial. (d) 3D volume reconstruction.
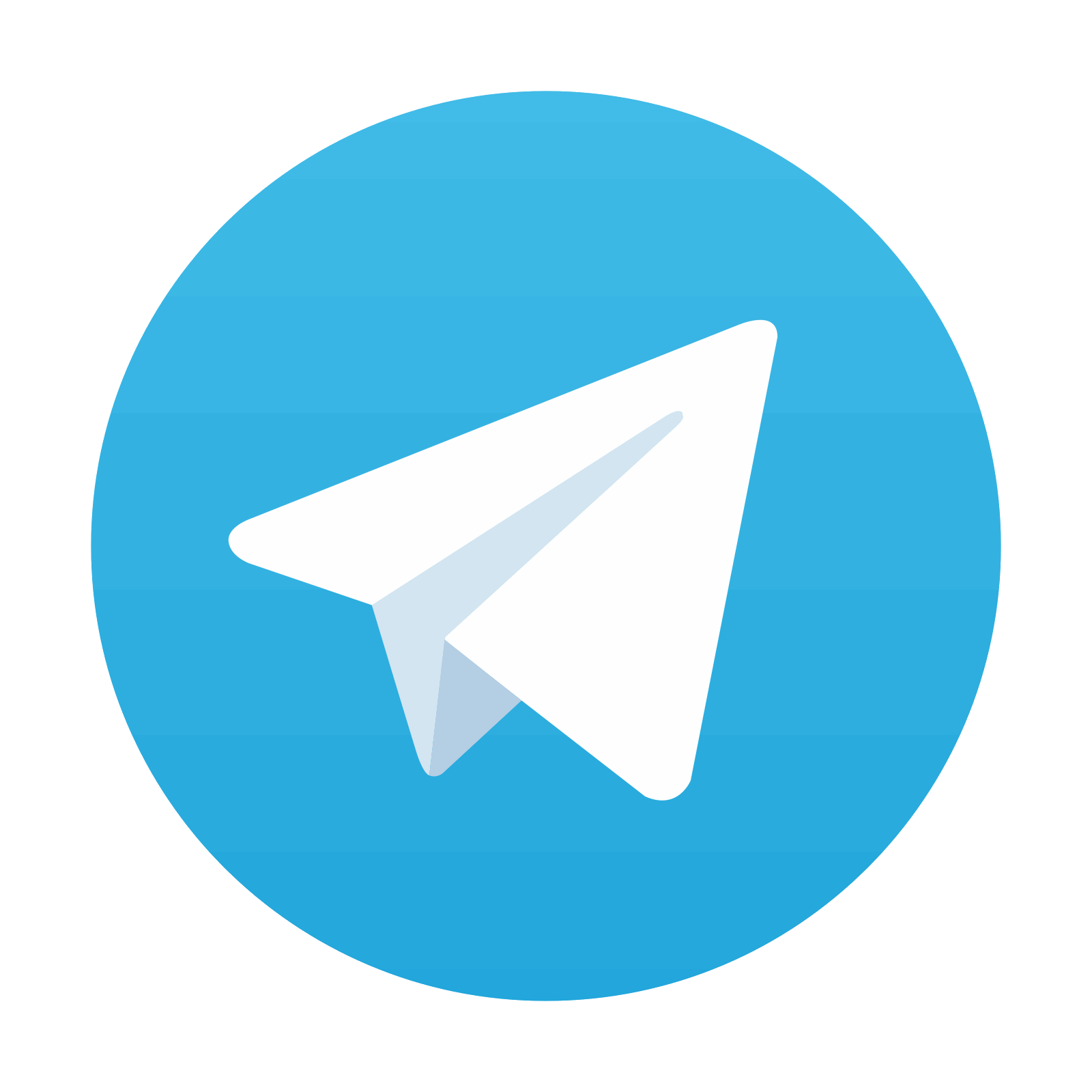
Stay updated, free articles. Join our Telegram channel

Full access? Get Clinical Tree
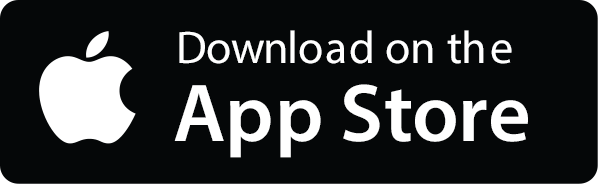
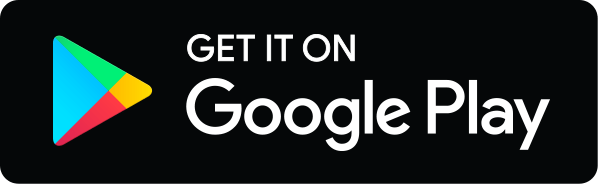