Purpose
To evaluate rates of change measured with scanning laser polarimetry with enhanced corneal compensation (GDx ECC) and compare them to those measured using the variable corneal compensation (GDx VCC) method in a cohort of glaucoma patients and individuals suspected of having the disease followed over time.
Design
Observational cohort study.
Methods
The study included 213 eyes of 213 patients with an average follow-up time of 4.5 years. Images were obtained annually with the GDx ECC and VCC, along with optic disc stereophotographs and standard automated perimetry (SAP) visual fields. Progression was determined by the Guided Progression Analysis software for SAP and by masked assessment of stereophotographs by expert graders. Joint linear mixed-effects models were used to evaluate rates of change in GDx measurements and their relationship with disease progression.
Results
Thirty-three patients (15%) showed progression over time on visual fields and/or stereophotographs. Mean rates of average retinal nerve fiber layer (RNFL) thickness change measured by the GDx ECC were significantly different in progressors versus nonprogressors (−1.24 μm/year vs −0.34 μm/year; P < .001). The area under the ROC curve for discriminating progressors from nonprogressors was significantly higher for rates of change measured by ECC compared to VCC (0.89 vs 0.65; P < .001).
Conclusion
Rates of RNFL change detected by the GDx ECC were significantly greater in eyes with progressive glaucoma compared to eyes with stable disease. Also, the ECC performed significantly better than the VCC for detection of change, suggesting that it could improve longitudinal evaluation of the RNFL with scanning laser polarimetry.
Detection of longitudinal change plays a central role in the diagnosis and management of glaucoma. Most glaucoma patients will show some evidence of progression if followed long enough; however, the rate of structural and functional deterioration can be highly variable among them. While some patients progress slowly over the course of many years or decades with minimal impact on the quality of vision, others have aggressive disease with rapid rates of change that can eventually result in blindness or substantial impairment unless appropriate interventions take place. The evaluation of rates of change is therefore a fundamental aspect in the management of this disease, so that resources can be directed towards the patients who are most likely to develop substantial impairment.
Optic disc stereophotographs have been considered the gold standard for evaluation of structural damage in glaucoma. However, assessment of rates of structural change using stereophotographs is difficult because of the qualitative and subjective nature of this assessment. The use of objective and quantitative structural measurements derived from imaging technologies has facilitated the assessment of rates of structural damage in the disease. One of these technologies, scanning laser polarimetry (SLP), provides quantitative estimates of the thickness of the retinal nerve fiber layer (RNFL) with potential use for diagnosis and follow-up of glaucoma patients. The GDx with variable corneal compensation (GDx VCC; Carl-Zeiss Meditec, Inc, Dublin, California, USA) has been successfully used to evaluate rates of progressive RNFL loss in glaucoma. However, the presence of an artifact known as atypical retardation patterns (ARP) on GDx VCC scans has been shown to adversely affect its ability to monitor RNFL loss over time. Medeiros and associates showed that eyes with large amounts of atypical patterns and/or great fluctuations on these patterns over time may show changes in GDx VCC measurements that can falsely appear as glaucomatous progression or mask true changes in the RNFL. ARPs show a prevalence of up to 51% in glaucomatous eyes and seem to result from poor signal-to-noise ratio as a consequence of light scattering in the eye.
In order to improve neutralization of ARPs, a new software-based method of corneal compensation called enhanced corneal compensation (ECC) has been developed. This method can be incorporated into the GDx VCC instrument and does not require changes to the hardware. In cross-sectional studies, the GDx ECC performed significantly better than the VCC for diagnosing disease in eyes with ARPs. However, no longitudinal study has yet compared the performance of these 2 imaging techniques for assessment of progressive glaucomatous damage and rates of structural change in the disease.
The purpose of the current study was to evaluate rates of change measured with GDx ECC and compare them to those measured using the VCC method in a cohort of glaucoma patients and individuals suspected of having the disease followed over time.
Methods
This was an observational cohort study. Participants from this study were selected from a prospective longitudinal study designed to evaluate optic nerve structure and visual function in glaucoma (DIGS—Diagnostic Innovations in Glaucoma Study) conducted at the Hamilton Glaucoma Center, University of California, San Diego. Participants in the DIGS were longitudinally evaluated according to a pre-established protocol that included regular follow-up visits in which patients underwent clinical examination and several other imaging and functional tests. All the data were entered in a computer database. All participants from the DIGS study who met the inclusion criteria described below were enrolled in the current study. Informed consent was obtained from all participants. The University of California San Diego Human Subjects Committee approved all protocols and the methods described adhered to the tenets of the Declaration of Helsinki.
At each visit during follow-up, subjects underwent a comprehensive ophthalmologic examination including review of medical history, best-corrected visual acuity, slit-lamp biomicroscopy, intraocular pressure (IOP) measurement, gonioscopy, dilated funduscopic examination, stereoscopic optic disc photography, and automated perimetry using Swedish interactive threshold algorithm (SITA). Only subjects with open angles on gonioscopy were included. Subjects were excluded if they presented best-corrected visual acuity less than 20/40, spherical refraction outside ±5.0 diopters and/or cylinder correction outside 3.0 diopters, or any other ocular or systemic disease that could affect the optic nerve or the visual field.
The study included patients diagnosed with glaucoma as well as patients suspected of having the disease, as determined on the baseline visit. Eyes were classified as glaucomatous if they had repeatable (2 consecutive) abnormal visual field test results on the baseline visits, defined as a pattern standard deviation outside of the 95% normal confidence limits, or a glaucoma hemifield test result outside normal limits, regardless of the appearance of the optic disc. Eyes were classified as glaucoma suspects if they had a history of elevated intraocular pressure (>21 mm Hg) and/or suspicious or glaucomatous appearance of the optic nerve but normal and reliable visual field results on the baseline visits.
Images were obtained annually with GDx VCC and ECC. Eligible subjects were required to have had a visual field examination and optic disc stereophotographs taken close in time (within 6 months) to the baseline and last GDx scans. Baseline was set at the first occurrence of this matching and the GDx date was used as the baseline date. During follow-up time, each patient was treated at the discretion of the attending ophthalmologist.
Standard Automated Perimetry and Stereophotograph Grading
Only reliable tests (≤33% fixation losses and false negatives, and <15% false positives) were included. Glaucomatous visual field progression was assessed using the HFA Guided Progression Analysis (GPA) software (Carl-Zeiss Meditec, Inc). Progression by standard automated perimetry (SAP) GPA was defined as a statistically significant decrease in the pattern deviation values from the average of 2 baseline examinations. Change in 3 or more of the same test points on 3 consecutive follow-up tests were required for confirmed progression.
Simultaneous stereoscopic optic disc photographs (TRC-SS; Topcon Instrument Corp of America, Paramus, New Jersey, USA) were reviewed using a stereoscopic viewer (Asahi Pentax Stereo Viewer II; Asahi Optical Co, Tokyo, Japan). For progression assessment, each patient’s most recent stereophotograph was compared to the baseline one. Definition of change was based on focal or diffuse thinning of the neuroretinal rim, increased excavation, or appearance or enlargement of RNFL defects. Evidence of progression was based on masked (patient name, diagnosis, temporal order of photographs) comparison between the baseline and most recent photograph, by 2 observers. If these observers disagreed, a third observer served as an adjudicator.
When both eyes of the same individual were eligible for the study, the eye that showed progression on SAP and/or optic disc stereophotographs was selected for analysis. If none of the eyes had evidence of progression on these methods, 1 eye was randomly selected for analysis.
Scanning Laser Polarimetry
Patients were imaged using a commercially available scanning laser polarimeter with variable corneal compensation (GDx VCC, software version 5.5.1; Carl-Zeiss Meditec, Inc) and enhanced corneal compensation (GDx ECC; Carl-Zeiss Meditec, Inc). The general principles of scanning laser polarimetry and the algorithms used for variable and enhanced corneal compensation have been described in detail elsewhere. Assessment of GDx image quality was performed by an experienced examiner masked to the subject’s identity and results of the other tests. The same criteria were applied to ECC and VCC scans. To be classified as good quality, an image required a focused and evenly illuminated reflectance image with a centered optic disc. The image quality score had to be greater than or equal to 7.
RNFL retardation measurements were obtained on a 3.2-mm-diameter calculation circle around the optic nerve head. Several parameters were calculated from RNFL measures obtained within this calculation circle and used in this study: TSNIT average (average of RNFL measurements obtained on the 360 degrees around the optic nerve; T stands for temporal, S for superior, N for nasal, and I for inferior), inferior average, superior average, nasal average, and temporal average. The GDx provides measurements of RNFL retardation, which are converted to estimated RNFL thickness using a fixed conversion factor.
To quantify the presence of ARPs on GDx VCC scans, we used the software-provided parameter Typical Scan Score (TSS). The TSS is a continuous variable ranging from 0 to 100 and is the result of a support vector machine analysis of SLP data labeled for training based on the subjective appearance of each scan (typical vs atypical). TSS is based on the slope, standard deviation, and average magnitude of RNFL thickness measurements from the edge of the optic disc extending outwards to 20 degrees. Low TSS scores indicate atypical scans and high TSS scores indicate typical ones.
Statistical Analysis
Joint linear mixed-effects models with random intercepts and random slopes were used to evaluate rates of change in GDx VCC and ECC measurements and their relationship with disease progression, as evaluated by SAP and optic disc stereophotographs. We have previously applied these models for evaluation of change in imaging instruments and to model multivariate longitudinal processes.
Linear mixed models were used to evaluate the evolution of each response (ie, longitudinal ECC and VCC data) over time. In these models, the average evolution of a specific response is described using some function of time, and subject-specific deviations from this average evolution are introduced by random intercepts and random slopes, allowing for different baseline values and different rates of change for each patient. In a joint-modeling approach using mixed models, random effects are assumed for each response process and the different processes are associated by imposing a joint multivariate distribution on the random effects.
Each response process is described using a linear mixed-effects model:
Y 1 i ( t ) = μ 1 ( t ) + a 1 i + b 1 i t + ε 1 i ( t )
Y 2 i ( t ) = μ 2 ( t ) + a 2 i + b 2 i t + ε 2 i ( t )
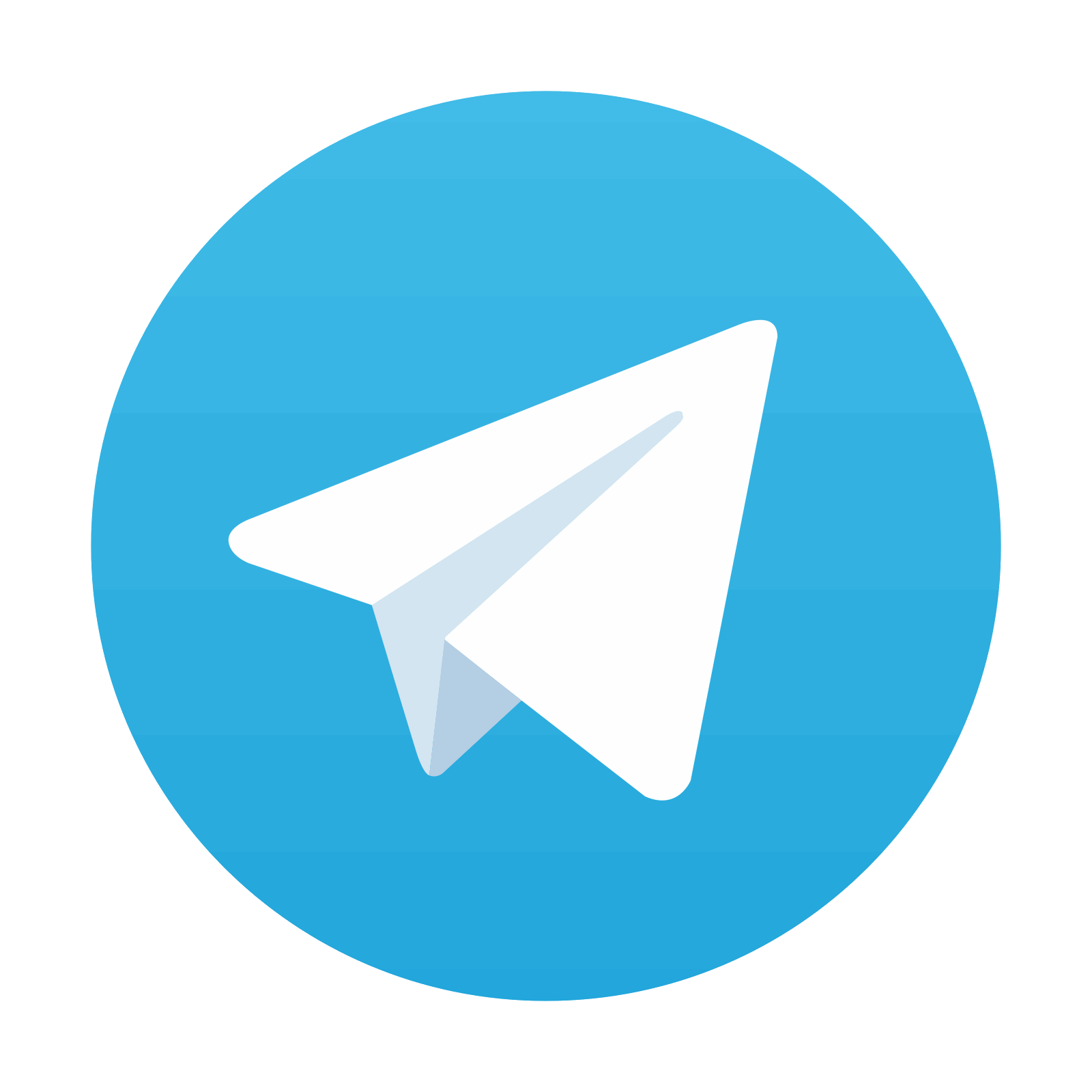
Stay updated, free articles. Join our Telegram channel

Full access? Get Clinical Tree
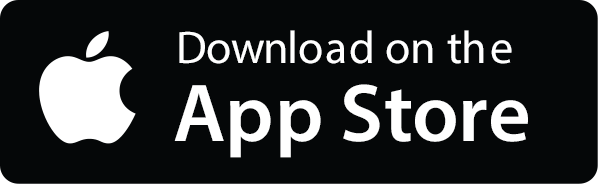
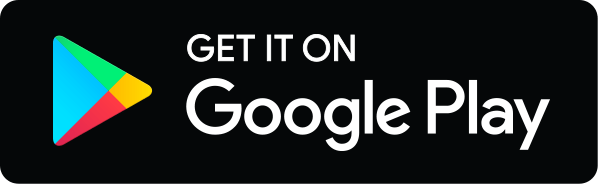