Radiation Therapy
Jerome S. Schwartz
Gady Har-El
Hassan Aziz
Radiation therapy is based on directed ionizing radiation used to manage malignant and, occasionally, benign tumors. Radiation therapy can be used as a curative modality, as combination therapy with chemotherapy or surgical excision, as postoperative salvage, as palliative therapy, or as prophylactic treatment to suppress cancer proliferation. Delivery of ionizing radiation is accomplished with an external source (external beam radiation therapy) or within the target tissue by means of implantation of radioactive sources (brachytherapy).
Ionizing radiation causes three types of injury—immediate cellular death, temporary mitotic arrest, and loss of reproductive capacity—in biologic tissue through the formation of free radicals, which cause direct and indirect cellular damage. The first type of damage is immediate cellular death of highly radiosensitive tissue such as lymphocytes. With the second type, temporary arrest of mitosis, the length of time the cell is arrested depends on the duration of radiation exposure and the location of the cell in the cell cycle during radiation exposure (increased length when cells are in the G2 phase). The third type of injury is loss of reproductive integrity. The direct interaction of ionized particles with DNA changes the structure of the DNA, leading to ineffective repair of sublethal or lethal damage.
Radiation therapy seems primarily to cause its damage indirectly by producing free radicals that interact with intracellular substrates. These macromolecules are broken apart by the free radicals, causing an increase in osmotic pressure (intracellular) and lysis of the cells after a critical pressure is reached. Not all cells in the radiation field die, because free radical-induced sublethal DNA damage is repaired by both the surrounding noncancerous normal cells and by tumor cells. Repair of DNA damage by normal cells is superior, therefore providing an opportunity for the normal cells to recover and the tumor cell to die.
Radiosensitivity is the vulnerability of cells to lethal injury from ionizing radiation, and is unique to each type of tumor. Radiosensitivity does not necessarily correlate with curability. A radiosensitive tumor adjacent to vital organs might require a dose of radiation that will cause intolerable tissue damage to the surrounding normal cells. Similarly, a radiosensitive tumor that has metastasized to vital organs might not be curable. Therapeutic ratio is defined as the radiation tolerance dose of normal tissue divided by the lethal dose of radiation of the tumor mass. The higher the ratio, the better is the chance of complete response. Factors that increase the numerator and decrease the denominator are helpful, because local normal tissue is spared while the tumor is eradicated. A positive therapeutic ratio is a valuable tool for radiation oncologists.
The first factor to alter the therapeutic ratio is oxygen. Although oxygen is necessary for the formation of free radicals,
the effect of oxygen on radiosensitivity comes to a plateau at a PO2 of about 30 mm Hg. Above this level, no more benefit is derived from adding more oxygen. Conversely, low oxygen tension has been shown to contribute to radioresistance. Large tumors are difficult to manage because there are areas within the tumor where the cells have outgrown their blood supply. These are areas of relative hypoxia, which decreases free radical formation.
the effect of oxygen on radiosensitivity comes to a plateau at a PO2 of about 30 mm Hg. Above this level, no more benefit is derived from adding more oxygen. Conversely, low oxygen tension has been shown to contribute to radioresistance. Large tumors are difficult to manage because there are areas within the tumor where the cells have outgrown their blood supply. These are areas of relative hypoxia, which decreases free radical formation.
The second factor is hyperthermia. It is well established that heat alone can kill tumor cells. The cell survival curves for heat and radiation therapy can be superimposed. Hyperthermia and radiation therapy act synergistically. Hyperthermia can be delivered through hot water baths, soft-wave diathermy, radio frequency-induced currents, microwaves, and ultrasound. Cumbersome methods of heating and the inability to achieve a uniform temperature seem to be the greatest problems with this method.
The third factor to alter radiosensitivity and curability is drugs that increase the sensitivity of tissues to radiation, as well as a newer class of drugs that protect normal well-oxygenated cells from the effects of the radiation therapy. Chemicals with electron affinity, such as misonidazole, seem to increase the therapeutic ratio for hypoxic cells, whereas some sulfhydryl compounds can act as chemoprotectors in normal cells with normal oxygen tension. The workhorse agents used are 5-fluorouracil and cisplatin, which act synergistically to prime the cells for radiation therapy.
Once radiation therapy is chosen as an option in the multidisciplinary treatment plan, implementation of the plan begins with evaluation of the patient. Radiographic localization of the tumor is undertaken to guide port selection. A firm understanding of the natural routes of tumor spread in the head and neck is a prerequisite to proper selection of the radiation field. With a head and neck restraint as a phantom, the proposed radiation field is marked, and port size is determined. Port size should be large enough to include the entire tumor and possible zones of spread with a cuff of normal tissue, while sparing most of the unaffected surrounding structures.
The source of ionizing radiation is based on the location and size of the tumor, amount and depth of tissue penetration desired, and availability of sources. The radiation oncologist chooses between external beam radiation and brachytherapy and chooses the ionizing particles to be used—electrons, photons, α particles, protons, or neutrons. Brachytherapy is the placement of isotope into the tumor mass itself as an interstitial implant, either permanently or temporarily. This allows the tumor to receive a higher radiation dose with relative sparing of the surrounding tissues.
The depth of radiation penetration depends on the voltage used (increased voltage equals increased depth penetration), as well as the type of radiation administered. Superficial lesions such as carcinoma of the skin require a source that provides shallow penetration, such as x-ray or electron beam radiation. Deeper lesions require megavoltage x-rays or cobalt-derived gamma rays, which spare superficial structures and penetrate deeply. Neutron beams deliver the most energy to tissues per
unit of distance than any other source (high linear energy transfer) but are very expensive, inaccessible, and still considered experimental. Neutron beams eliminate the hypoxic protection of cells, spare the skin, and fail to penetrate bone, which in turn reduces the incidence of osteoradionecrosis.
unit of distance than any other source (high linear energy transfer) but are very expensive, inaccessible, and still considered experimental. Neutron beams eliminate the hypoxic protection of cells, spare the skin, and fail to penetrate bone, which in turn reduces the incidence of osteoradionecrosis.
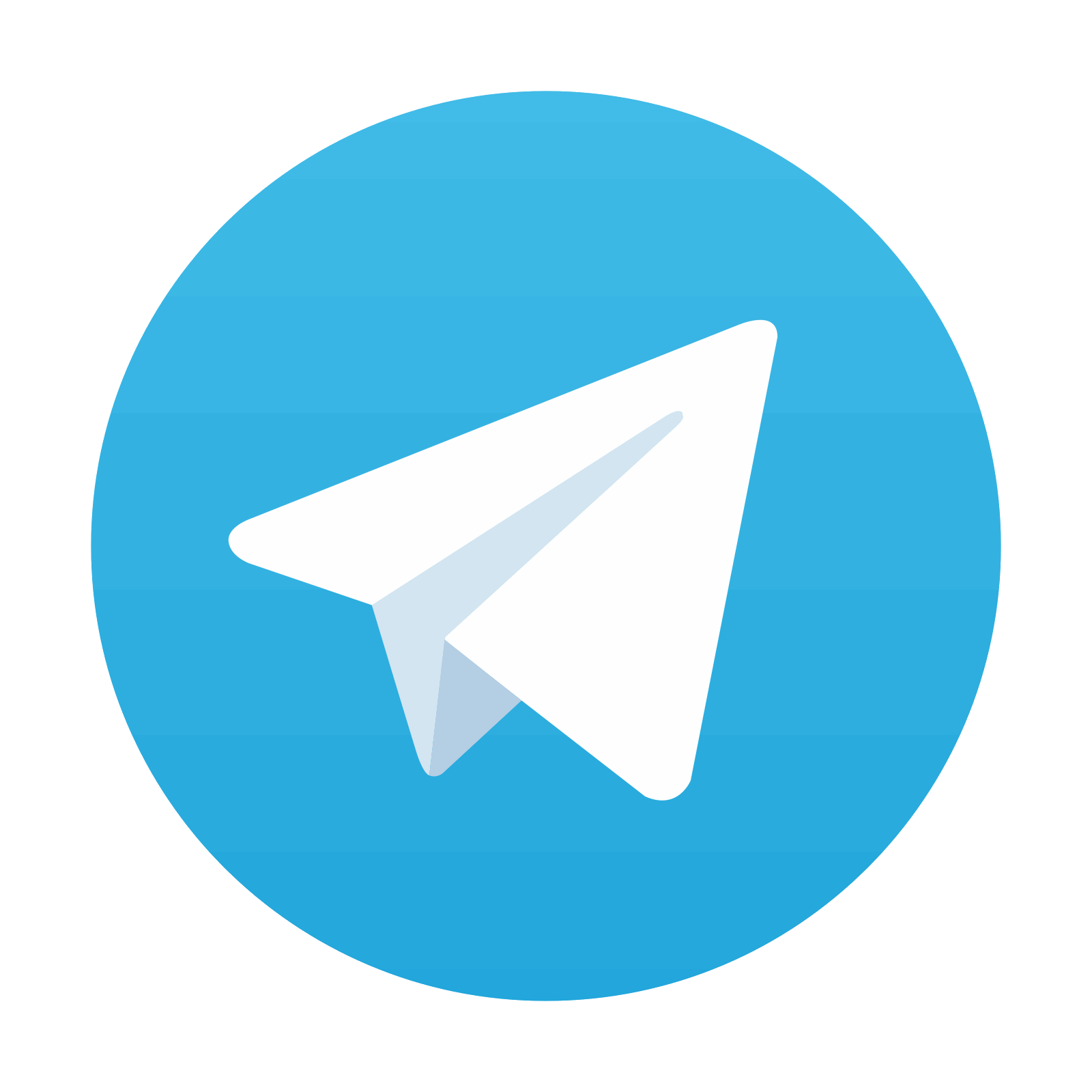
Stay updated, free articles. Join our Telegram channel

Full access? Get Clinical Tree
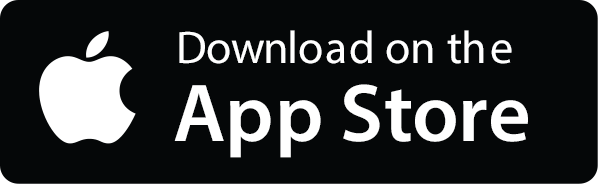
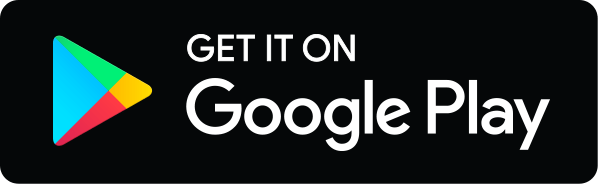