Yali Jia, PhD; Acner Camino, PhD; Miao Zhang, PhD; Simon S. Gao, PhD; and David Huang, MD, PhD
Optical coherence tomography angiography (OCTA) produces en face angiograms that allow for the clear visualization of retinal and choroidal vasculature.1 Changes such as reduced flow, loss of vasculature, and/or abnormal vessel formation as a result of pathology are readily apparent. Significant progress has been made with regards to quantifying such changes and demonstrating OCTA’s ability to provide objective information. In this chapter, we will discuss the nature and utility of quantification parameters derived from OCTA in the diagnosis and assessment of diseases affecting the posterior eye including glaucoma, diabetic retinopathy (DR), age-related macular degeneration (AMD), and choroideremia.
BUILDING BLOCKS TO QUANTIFICATION
Flow Signal
It is important to first understand a few peculiarities about the flow signal in OCTA. OCTA relies on assessing the blood flow-induced variation in the optical coherence tomography (OCT) signal over time to distinguish vasculature from static tissue. Different OCTA implementations assess this variation differently, but the resulting flow signals have similar characteristics. Calibration experiments from flow phantoms show that this flow signal, in the split-spectrum amplitude-decorrelation angiography (SSADA) implementation and likely other OCTA implementations, is positively correlated to flow velocity.2 However, there exists both a sensitivity and saturation limit. The sensitivity limit is the lowest detectable flow, while the saturation limit is the fastest flow beyond which the flow signal does not increase further. The 2 limits or dynamic range are inversely related to the sampling time, meaning increasing the time between a pair of B-scans used for flow calculation decreases both limits.
The flow signal in microvasculature can be further modified depending on the size of the vessel relative to the lateral resolution of the OCT system. When beyond the saturation speed, differences in flow signal are a result of differences in vessel diameter. The corollary is that flow signal is thus also dependent on scan size when sampling is fixed. Therefore, quantitative comparison of results makes sense only when performed between scans of equivalent size and sampling.
Region of Interest
While most of the inner retina is supported by a continuous vascular network, no retinal vessels exist within the foveal avascular zone (FAZ). In healthy eyes, the FAZ area extends to a diameter of approximately 0.6 mm and is surrounded by 2 important regions, the parafovea and the perifovea (Figure 6-1). The parafovea is an annular region of 0.95 mm in width extending beyond the FAZ. The perifovea is an annular region of 1.5 mm in width circumscribing the parafovea. Quantification of vessel density and flow index in the parafoveal and perifoveal region is a common way of assessing macular perfusion.
Figure 6-1. A normal eye. (A) Color fundus photograph. (B) A 6 × 6 mm en face macular retinal OCT angiogram with concentric circles shows the foveal avascular zone (FAZ, 0.6 mm diameter), parafoveal, and perifoveal regions. (C) Disc fundus photo 3 × 3 mm OCTA of the optic disc and peripapillary region. (D) En face OCTA of the disc and the peripapillar retina. Flow parameters in the peripapillar retina can be measured in the annular region between the 2 dashed green circles.
Other diseases such as glaucoma manifest in the surrounding areas of the optic nerve head. In such cases, the flow index and vessel density has been computed for a 0.7 mm wide elliptical annulus that extends outward from the optic disc, known as the peripapillary retina (see Figure 6-1). There is no strict definition of the peripapillary region and larger annuli may be used for wider OCTA scans.
Quantification Metrics: Vessel Density and Flow Index
To assess the flow signal in a region of interest on the en face angiogram, 2 common metrics have been described. Vessel density describes the percentage area occupied by vessels within the region of interest and can be calculated as:
Flow index is a dimensionless quantity between 0 and 1 that is the average flow signal within the region of interest:
Flow index is proportional to the density of blood vessels (fractional area occupied by vessels) and related to flow velocity.3 To determine whether V is 1 or 0, a threshold based on the flow signal in a known avascular region such as the FAZ can be used.4,5 It is usually set as 2.3 standard deviations above the mean decorrelation signal for the FAZ for normal controls.
Quantitative Metric: Nonperfusion Area
When the perfusion loss is more concentrated, an alternative metric called the nonperfusion area can be used to highlight the regions of capillary dropout. A nonperfusion or capillary dropout area is constructed by first identifying pixels with flow signal lower than a certain threshold on en face OCTA. Same as vessel density and flow index, this threshold can be based on flow signal from the FAZ. In addition, identified regions of nonperfusion smaller than the normal gap between vessels should not be considered. The total area of nonperfusion is then computed by adding the remaining identified regions. When reporting total nonperfusion area, the FAZ is not counted. In cases for which the distinction between FAZ and microvascular loss is not obvious, the total avascular area can be reported instead; this includes the FAZ and all identified nonperfusion areas.
Figure 6-2. Results from a normal eye (top row) and a perimetric glaucoma eye (bottom row). En face OCTA of the peripapillary retina shows generalized reduction and (D) an infratemporal area of focal capillary dropout (yellow arrow) in the glaucoma eye.7 (Reprinted with permission from Liu L, Jia Y, Takusagawa HL, et al. Optical coherence tomography angiography of the peripapillary retina in glaucoma. JAMA Ophthalmol. 2015;133(9):1045-1052.)
Quantitative Metric: Neovascularization Area
On the opposite side of capillary loss is neovascularization, where abnormal vessel growth occurs in regions typically devoid of vasculature, such as vitreous and outer retina. Neovascularization area is the sum of pixel areas on en face OCTA, usually presented in units of mm2, corresponding to abnormal vessels. As with the previously described methods, a threshold is used to classify pixels.
DEMONSTRATION IN DISEASE
Quantification of Peripapillary Retina Perfusion in Glaucoma
In addition to elevated intraocular pressure, vascular insufficiency may play important roles in the pathophysiology of glaucoma. This suggests another possible imaging target for early diagnosis and monitoring. Jia et al recently quantified optic disc perfusion using the SSADA algorithm on a custom swept source OCT and showed decreased disc perfusion in glaucoma.6 On the commercial 70 kHz spectral OCT, Liu et al found the optic nerve head flow index to be less reliable because of the absence of OCT signal in segments of major blood vessels.7 The loss of signal in rapidly moving fluids, known as “flow voids,” occurs because of washout of interferometric fringes within the OCT signal integration time.8 Fringe washout occurs at a lower velocity range in spectral OCT compared to swept source OCT.8 Thus it would interfere with the accurate measurement of optic nerve head flow index more in spectral OCT than swept source OCT. Therefore, more studies have concentrated on the peripapillary microvasculature, which could be visualized with excellent capillary detail using spectral OCTA. The peripapillary retina also offers a larger area over which focal perfusion defects can be seen more easily (Figure 6-2). Using OCTA, Liu et al found reduction in peripapillary retinal perfusion in eyes with glaucoma could be visualized as focal defects and quantified as flow index and vessel density with high repeatability and reproducibility.7
Quantification of Macular Perfusion in Diabetic Retinopathy
DR affects retinal vessels by causing the loss of cellular components such as perycites and endothelial cells. These processes lead to the onset of pathologies like vessel occlusion, hyperpermeability, and sometimes neovascularization. One of the early physiologic manifestations of the disease is that microcapillaries are left with no flow, a phenomenon known as capillary dropout. As this progresses, visible regions of no perfusion start to be visualized on angiograms. An important mechanism in the loss of vision due to DR is capillary dropout in the region of the parafoveal belt, an important nourishing source of the avascular fovea. OCTA is capable of visualizing capillary dropout as areas of no flow where in healthy eyes there should be vasculature (Figure 6-3).
Figure 6-3. Quantification of inner retinal blood flows in normal control (upper panels, A1 to C1) and nonproliferative diabetic retinopathy (NPDR) with macular edema (lower panels, A2 to C2). OCTA was acquired using a 70 kHz spectral OCT system with a center wavelength of 840 nm (AngioVue [Optovue Inc]). (A1, A2) First column: (A1) fundus photo and (A2) fluorescein angiography. (B1, B2) Second column: en face 6 × 6 mm OCTA. (B2) Enlargement of the FAZ is present in the parafoveal region for NPDR. (C1, C2) Six nonperfusion areas (blue) are shown in the right-most panels of 6 × 6 mm OCTA. The normal individual had a FAZ of 0.30 mm2, while the NPDR case showed an enlarged FAZ and scattered areas of macular nonperfusion totaling 7.07 mm2.
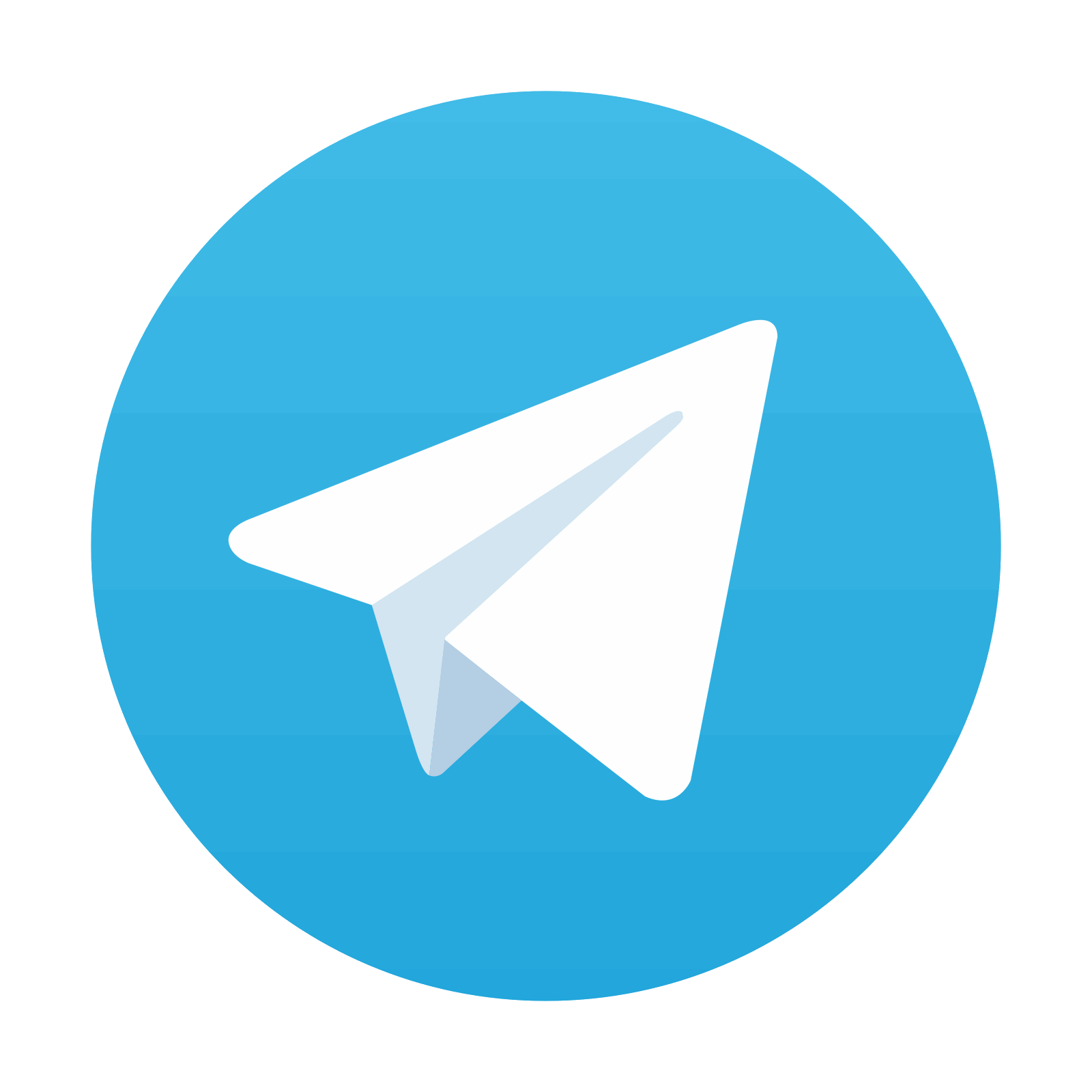
Stay updated, free articles. Join our Telegram channel

Full access? Get Clinical Tree
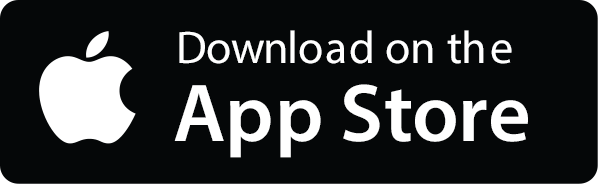
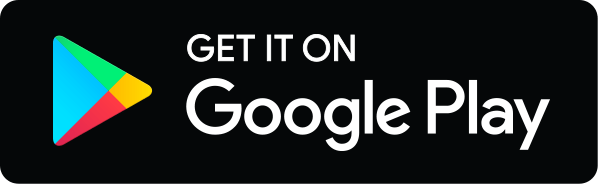