Fig. 35.1
Echo-acoustic sensitivity to target range. Colour bars represent performances of individual subjects
To solve this task, subjects produced relatively short vocalisations (tongue clicks) with durations between 3 and 12 ms. The click SPLs, measured at the headset microphone, were more variable, ranging from about 60–105 dB. Also the number of clicks produced to evaluate the reflection properties in a 5 s interval of the 2AFC task varied across the subjects with individual averages between only 6 and up to 23 clicks. Most subjects produced relatively high-frequency clicks with peak frequencies around 6–7 kHz and −15 dB bandwidths from 3 to 10 kHz or 15 kHz.
3 Echolocation vs. Echo Suppression in Humans
The precedence effect predicts a conflict of echolocation and echo suppression: when localising sound sources, the human auditory system suppresses spatial information about echoes, but just this information underlies effective echolocation. A common approach to investigate the precedence effect is the arrangement of two sound sources that present a direct sound (lead) and a delayed copy (lag). Several experiments on lag-discrimination suppression have quantified the deterioration of spatial information about the lag produced by the lead. Here, minimum audible angles (MAAs) were measured in VEAS. In the ‘listening’ version, the subjects had to discriminate between positions of a single sound source, the leading or the lagging of two sources. In the ‘echolocation’ version, the sound sources were replaced by sound reflectors. Here, the subjects evaluated the echoes generated in real time from self-produced tongue clicks and thereby discriminated between positions of a single reflector, the leading or the lagging of two reflectors.
3.1 Stimuli
Individual HRIRs and VIRs, measured at 10° azimuthal resolution, were interpolated to 0.2° to construct the stimuli. In the ‘listening’ version, the stimuli were acoustic impulses at a repetition rate of 2.5 Hz, convolved with the required HRIRs. The lead-lag delay was 2 ms. In the ‘echolocation’ version, stimuli were generated by the listeners (typically tongue clicks), and, in addition to the direct path described above, they were convolved in real time with first the VIR and then the HRIR and played back with a delay of 10 ms (lead) or 12 ms (lag). Thus the lead-lag delay was fixed at 2 ms, the distance to the leading virtual reflector was 1.7 m, and the distance to the lagging reflector was 2.04 m. Note that in the echolocation version, the perception of the echoes from the leading and lagging reflections was always preceded by the percept of the outgoing sound.
3.2 Procedure
In an adaptive 2AFC paradigm with audio feedback, MAAs were measured following a three-down, one-up rule. The beginning and end of each 5 s interval were marked by 50 ms tone pips. Intervals were long enough to allow listeners to explore the spatial layout of the reflectors in the ‘echolocation’ version with several tongue clicks. Listeners were extensively trained until they reached stable performance.
3.3 Results
MAA measurements for single objects presented in virtual space are shown in the top row of Fig. 35.2. Objects are either a sound source emitting impulses (listening) or a surface reflecting the subjects’ tongue clicks (echolocation).
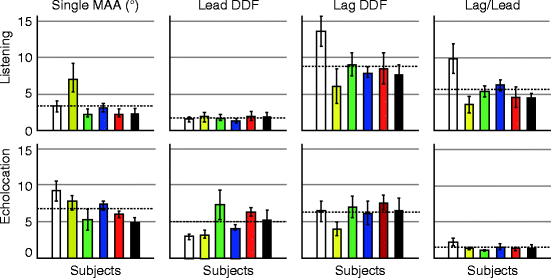
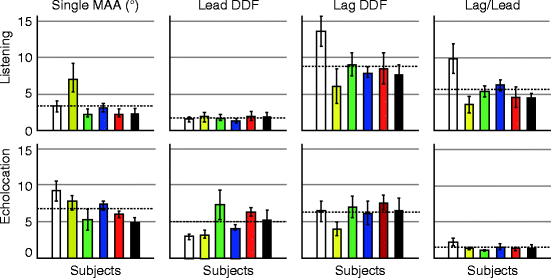
Fig. 35.2
Precedence in a listening and an echolocation task. Colour bars represent individual data
The data show that sighted subjects can be trained to discriminate reflective surfaces by echolocation with an accuracy comparable to sound-source localisation. Data from six subjects reveal a mean MAA of 3.4° in the listening version and 6.7° in the echolocation version; individual MAAs are shown in the first row of Fig. 35.2.
An appropriate method for comparing results across versions is the calculation of the discrimination deterioration factor (DDF), defined as ratio of lead/single or lag/single thresholds (Litovsky 1997; Tollin and Henning 1998). Individual DDFs for lead and lag discrimination are shown in the second and third rows of Fig. 35.2, respectively.
In the listening version, the presence of a lagging source impaired lead discrimination only slightly by a factor of 1.6 (second row, left), while a leading source impaired lag discrimination considerably by a factor of 8.8 (third row, left). This asymmetry between lead and lag discrimination is consistent with previous studies on discrimination suppression (Litovsky et al. 1999).
In the echolocation version, however, this asymmetry was significantly weaker: lead and lag discrimination deteriorated by factors of 4.8 and 6.2, respectively. To quantify the asymmetry between lead and lag discrimination as the defining measure of the influence of the precedence effect, the ratio of lag/lead thresholds was calculated and depicted in the bottom row of Fig. 35.2. The strength of precedence was significantly higher in the listening experiment.
These data indicate that the precedence effect is weakened in an echolocation context.
4 Human Sonar Sensitivity to Room Size
Echolocation does not only allow determination of the distance and position of objects in space but also assessment of the dimensions of an enclosed space itself. From architectural acoustics, it is known that the reverberant qualities of an enclosure can be quantified in terms of a binaural room impulse response (BRIR): the BRIR incorporates the spatial and temporal distribution of reflections which a sound undergoes on its way from a specific source to a human, binaural receiver. In the current experiment, we aimed to formally quantify the ability of sighted human subjects to detect changes in the size of an enclosed space by evaluating echoes of the subjects’ own vocalisations.
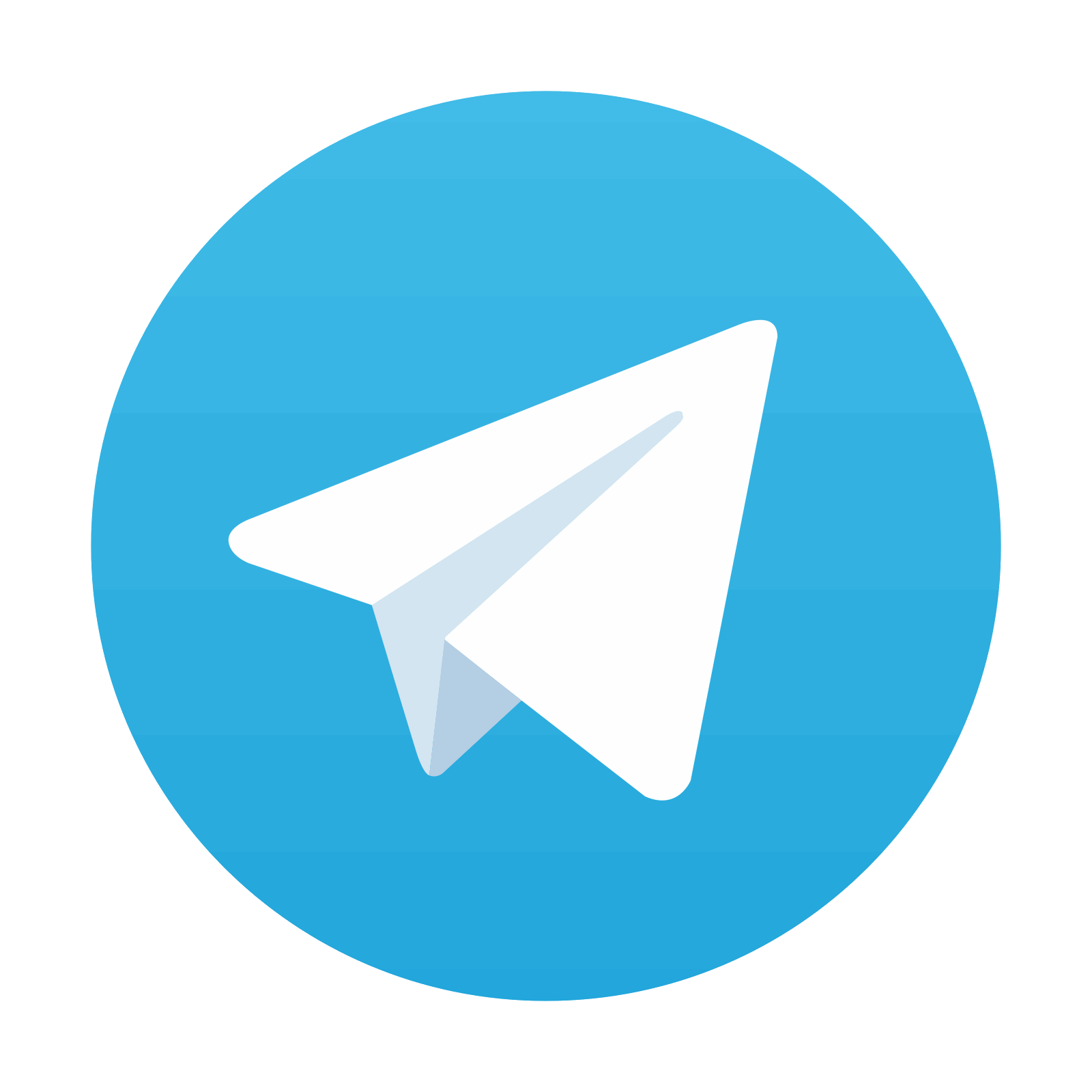
Stay updated, free articles. Join our Telegram channel

Full access? Get Clinical Tree
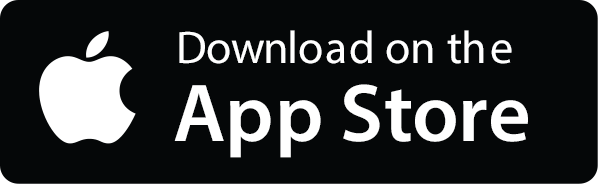
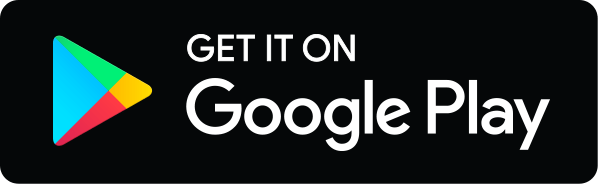