Fig. 4.1
Slit lamp photograph of normal radial keratotomy incisions
Procedure
The surgeon makes a number of uniform microscopic cuts with a diamond-blade knife into the cornea to sever the collagen fibrils in the corneal stroma. These cuts are set to a specific depth (ideal depth between 85–90%) and range between 4 and 32 in number. The incisions cause mid peripheral bulging of the cornea with a resultant flattening of the central cornea inducing a hyperopic shift. The diameter of the central optical clear zone is inversely related to the amount of refractive correction; for example, a 3 mm central clear zone yields a greater myopic correction than a 4 mm central clear zone.
Structural Effects of RK on the Cornea
Keratotomy wounds are partial thickness, unsutured, and typically not treated with prolonged topical corticosteroids. There are numerous studies looking at the structure and strength of these wounds in vitro, in both histologic studies and animal models. Histologically, RK incisions produce a sharp demarcated break in Bowman’s layer and the stroma causing flattening and posterior bending of the corneal lamellae and Descemet’s membrane toward the anterior chamber [2]. Additionally, when an incision exceeds 80% of the stromal thickness, there is loss of the endothelial cells in the areas of the incision [2].
With normal corneal wound healing, an epithelial plug, a bed of corneal epithelial cells that grow into the incision, forms but then regresses within 6–14 days. After 3–6 months, fibrocytes start to migrate to the wound resulting in a scar. The resultant scar, however, never regains its original tensile strength. Contrary to normal corneal wound healing, histological studies have shown that following RK the normal epithelial plug remains in the incision up to five and a half years and active fibroblasts have been shown to persist for many years as well. As a result of these wound changes, there is a wound gape which leads to refractive fluctuations due to changes in stromal wound healing and remodeling. This can be observed by slit lamp examination by observing a gray haze adjacent to the incisions. Due to a break in the continuity of the collagen fibrils once the wound is healed, the wound integrity is never the same as a normal cornea.
The reduced integrity of an RK incision is demonstrated by a case report by Jammal [3] who described multiple RK wound ruptures 20 years after surgery secondary to blunt trauma. Additionally, Glasgow wrote about a case of a 55-year-old male who sustained a traumatic dehiscence of a 7-year-old RK incision [4]. Upon microscopic examination of this patient’s enucleation specimen, Glasgow et al. [4] observed persistent epithelial plugs in RK incisions. From this study, the authors hypothesized that the cornea may be susceptible to rupture from blunt trauma due to the presence of the epithelial plugs. Bryant et al. [5] studied human corneas 8 years after RK. They cut the cornea into strips and subjected various areas of the cornea with and without RK incisions to tensile testing by loading weights until rupture occurred. They concluded that indeed epithelial plugs cause a relative weakness in the RK wounds compared to the surrounding stroma.
The most common locations for globe rupture in the normal eye when subjected to blunt trauma are circumferential scleral arcs parallel to the limbus, just posterior to the muscle insertions, and at the equator. Luttrull et al. [6] studied enucleated porcine eyes and found that when incisional depths exceed 70%, there is an increased incidence of corneal rupture after blunt trauma; however, incisions that are 70% and less behaved more like normal globes, rupturing at the equator. Larson et al. [7] found that 98% of wound ruptures in rabbit eyes following 8-incision RK occurred in one or more of the incisions. Studies have also shown that in post-RK eyes, 54% less force was needed to cause a corneal rupture than in control eyes. Additionally, incisions traversing the limbus, microperforations during the surgery, and deeper incisions increased the incidence of a wound rupture. Fewer RK incisions, however, do not appear to be protective as the number of incisions does not appear to affect the likelihood of corneal rupture [8, 9].
Mini-radial keratomy (mini-RK) limits the extent of radial incisions from the 3 mm optical zone to the 7 mm optical zone, compared with “conventional” RK which extends to the 11 mm optical zone. Pinheiro et al. [10] showed that corneas that underwent mini-RK required significantly higher pressures to rupture than corneas that underwent regular RK. Steinmann [11], similarly, demonstrated that longer RK incisions require a lower force to rupture.
Trauma-Associated Complications with RK
Wound Rupture/Dehiscence
One of the worst trauma-related complications that occurs post-RK is a wound rupture. Even among the estimated 2–3 million eyes that have undergone RK [12], wound rupture appears to be relatively uncommon [12]. Based on animal models, the most common rupture pattern was a “cut-to-cut” rupture in which two RK incisions were connected by the rupture site (Fig. 4.2) [7]. The next most common rupture patterns by decreasing frequency are a radial corneoscleral laceration along an RK incision followed by a stellate rupture, in which 3 or more RK incisions rupture and connect to form a single laceration, followed by an “incision-opening” rupture involving a single RK incision without extension [13]. Examples of each of these rupture patterns have been described in case reports in human eyes [8].


Fig. 4.2
External photograph of an expulsive hemorrhage through a radial keratotomy “cut-to-cut” rupture. Note the black arrow indicating an intact RK incision as well as the red arrow indicating the “cut-to-cut” rupture. Photograph courtesy of Lori Stec, MD
Vinger et al. [14] studied the largest case series of 28 RK-related wound ruptures. They found that the rupture extended across the visual axis in over 70% of the eyes, which they suggested occurred due to the close proximity of the incisions to the visual axis. Another hypothesis is that a corneal dehiscence is more likely to propagate along the thinner central corneal tissue [12].
In terms of timing, half of all RK-related reported wound ruptures occur within 2 years of surgery, and about two-thirds within 3 years of surgery [14]. After 3–4 years, there appeared to be a decline in the risk for wound ruptures [14]. This is consistent with experimental models that have shown that the RK wounds are likely more susceptible to trauma earlier on due to abnormal wound healing and presence of epithelial plugs.
We know that some direct force is required to create a RK wound rupture; however, the threshold of force required to create a wound rupture is difficult to quantify. McKnight et al. [15] performed an experiment on adult cat eyes. The cat eyes underwent RK with incision depth set at 90% and were subjected to BB pellet trauma of different velocities. While control eyes, those without RK incisions, developed hyphemas without any corneal ruptures, about one-third of RK eyes that underwent impact with the highest velocity (240 ft/s) developed wound ruptures [15].
Conversely, there are examples of significant ocular trauma that did not result in a keratotomy wound rupture, because the remaining 5–10% of non-incised corneal stroma with Descemet’s membrane appears to afford a relatively strong barrier to wound rupture [16]. Authors have described wound gapes that have resulted from blunt trauma [17]. Bouchard [18] described a bilateral case of anterior dehiscence of RK wounds without a wound rupture following a gasoline tank explosion injury. One month out from the incident, the wounds were well-healed with minimal irregularity and good vision. The degree and distribution of force may play a role in whether incisions rupture or just dehisce [18].
Epithelial Downgrowth
Epithelial downgrowth after RK is rare but can occur if deep radial incisions splay open during trauma [19]. The epithelial downgrowth may result from microperforation with implantation of epithelial cells into the anterior chamber from a preexisting epithelial plug that rapidly self-sealed [19]. If suspected, confocal microscopy allows noninvasive in vivo microscopic imaging to diagnose presumed epithelial downgrowth. Argon laser photocoagulation can only be used to confirm the diagnosis in cases with iris involvement.
Incisional Changes Without Dehiscence
Even without obvious trauma to the incisions on slit lamp examination, the incisions may still be affected. Forstot [20] reported a case of moderate, direct trauma that was significant enough to cause a subconjunctival hemorrhage and traumatic iritis. Although there was no opening of the RK incisions, they measured an increased keratometric cylinder of 1.5 diopters, which regressed spontaneously.
Incisional Infectious Keratitis
While relatively rare, infectious keratitis following RK may occur in the early or late postoperative period. Trauma has been found to be a risk factor for late-onset incisional keratitis as a source of microorganisms. Incisional keratitis may present with an infiltrate along the incision, conjunctival injection, surrounding corneal edema, with or without an anterior chamber reaction or hypopyon (Fig. 4.3). Pseudocysts within keratotomy wounds may be predisposing factors as they can lead to easier access for bacteria or occasionally break down, resulting in microscopic or macroscopic erosions [21]. Incisional keratotomy infections can potentially lead to endophthalmitis.


Fig. 4.3
Slit lamp photograph of infectious infiltrate along a radial keratotomy incision. Note the black arrow indicating the location of the infiltrate
Iatrogenic RK Incision Damage
Patients with previous RK can present a challenge when other ocular surgeries are necessary. Similar to blunt trauma, secondary surgical procedures can have an effect on RK wounds. There have been reports describing rupture of RK incisions during corneal transplant trephination, keratomileusis, phacoemulsification [18, 19, 22, 23], scleral buckling, and scleral depression [16].
Astigmatic Keratotomy
Introduction
Corneal astigmatism (mainly regular astigmatism) can be addressed by several astigmatic keratotomy techniques, often performed concurrently with cataract surgery. There are both incisional and ablative methods available to reduce or correct astigmatism. Astigmatic keratotomy incisional options (Fig. 4.4a) include tangential (horizontal/linear) keratotomy, arcuate keratotomy (AK), and limbal relaxing incisions (LRI). All of these incisions are corneal relaxing incisions made parallel to the limbus that lead to flattening in the meridian of the incision and steeping in the meridian 90° away. With tangential and arcuate incisions, the incisions are placed closer to the visual axis compared to an LRI incision. Since AK incisions are closer to the visual axis, this may amplify any errors if there is misalignment between the planned and treated axes. In contrast, LRI incisions are more peripheral, mitigating the potential for error and preserving the central corneal optical clarity. There is less patient discomfort with the LRI incisions and fewer corneal irregularities leading to reduced glare. More recently, the femtosecond laser has become an additional platform that can create precise AK incisions (Fig. 4.4b).


Fig. 4.4
a Slit lamp photograph of a normal astigmatic keratotomy. b Slit lamp photograph of femtosecond laser creation of limbal relaxing incision
LRI Procedure
For axis orientation, the cornea is marked at 12 and 6 o’clock in the upright position. After the patient has been positioned supine, the treatment axis and incision lengths can be marked based on the prior reference marks. Arcuate and tangential keratotomies can be performed either manually using a keratome or a diamond knife (often front-cutting), or by means of the femtosecond laser. These incisions are made approximately 95% depth in the steep meridian of the mid-peripheral cornea at the 7 mm optical zone. There are nomograms available, based on preoperative degree of cylinder and patient age, to direct a surgeon as to the placement of incisions.
The LRI incision is commonly made using a diamond knife (often back-cutting), although disposable and reusable metal LRI knives are also available. Both types often have preset depths for surgeon convenience, and the usual depth is 600 microns (range 450–650 microns) or 50 microns less than the thinnest limbal corneal thickness.
Structural Effects of AK on the Cornea
Deg et al. [24] found that wound healing after astigmatic keratotomy appears similar to the healing process following standard radial keratotomy with the exception that AK incisions promoted faster wound healing. This was attributed to the better apposition of tangential incisions while semi-radial/radial incisions tend to gape as a result of the mechanical stress of intraocular pressure (IOP). Tangential incisions were also found to have a shallower depth which can both aid in better wound healing as well as corneal structural integrity.
Trauma-Associated Complications with AK
Wound Rupture/Dehiscence
There are reports of AK wound rupture although it has only been reported in association with RK wound rupture. Lee et al. [25] reported a case where 4 out of 8 RK and 1 out of 1 AK incisions dehisced following direct blunt trauma to the eye. The 45-degree arcuate incision had been made at a 7.00 mm optical zone with a preset blade depth of 105% of the central corneal thickness [25]. Eggleston [26] presented a similar patient where half of the RK incisions were completely or partially ruptured, and a single transverse incision (2–3 mm from the limbus) also ruptured following blunt trauma. The radial incision ruptures had intersected the transverse incision to combine both into one rupture wound.
Jammal et al. [3] reported traumatic wound dehiscence appearing only in RK scars but not in any of the four transverse incision scars. It is unclear whether the decreased likelihood of AK incision dehiscence is based on a difference in geometry, incision orientation, or location in thicker corneal tissue. Both RK and AK incisions are typically placed at a similar depth in the cornea; however, the amount of residual intact corneal tissue is larger with transverse incisions, possibly making them less vulnerable to rupture. To date, there are no reports of isolated rupture of astigmatic keratotomy incisions or LRIs.
Incisional Infectious Keratitis
Incisions from AK are at risk for late-onset infectious keratitis following trauma in a similar manner as RK incisions (See Infectious Keratitis section under RK).
Intrastromal Corneal Ring Segments (ICRS)
Introduction
The concept of an intrastromal corneal implant was introduced in 1978 by Reynolds [27]; however, it was not used until 1991 when the first complete polymethyl methacrylate (PMMA) ring was inserted into the stroma for the correction of myopia. The procedure subsequently evolved from complete ring insertion to the insertion of two C-shaped rings in order to avoid insertion-related complications. The initial indication for the ICRS procedure was low myopia (−1.00 D to −3.00 D) and emerged as a unique, potentially removable refractive surgical device that does not invade the central optical zone [28]. The procedure has since been replaced by photoablative corneal surgery as the results have been shown to be more predictable. ICRS have been adopted for the treatment of corneal ectasia following LASIK [29] as well as severe keratoconus for treatment of irregular astigmatism and corneal instability (Fig. 4.5) [30, 31].


Fig. 4.5
Slit lamp photograph of a normal intrastromal ring placed inferiorly
Procedure
The surgeon marks the geometric center of the cornea with a Sinsky hook as a reference point. This procedure may be performed mechanically or with femtosecond laser-assisted technology. To complete the surgery mechanically, a 1 mm radial incision at 70–80% of the corneal thickness is created with a diamond blade knife along the steepest meridian. During the next step of the procedure, two continuous stromal tunnels are dissected by blunt separation of corneal lamellae for the insertion of the intrastromal ring segments. The dissected tunnels are created at 80% stromal depth with inner and outer diameters of 6.50 and 8.10 mm respectively. This step may be performed using a semi-automated suction ring and semicircular dissectors or using femtosecond laser technology. When using femtosecond laser technology, a single continuous circular tunnel is created rather than two tunnels. The ring segments are then manually inserted into the stromal tunnel. The radial incision may then be closed with glue or 10-0 Nylon suture.
Structural Effects of ICRS on the Cornea
The structural goal of refractive surgery for the correction of myopia is to flatten the cornea. The insertion of ICRS achieves this goal by the “arc shortening effect” in which the segment acts as a spacer between arching bundles of corneal lamellae therefore shortening the central corneal arc length. The stroma anterior to the ring segment is elevated while the posterior cornea is steepened resulting in central cornea flattening [32]. Without invading the central optical zone, this procedure induces morphologic change to reduce corneal steepening and astigmatism. Therefore, it does not depend on the healing properties of the cornea in order to have an effect.
There are four types of intrastromal ring segments that differ in various characteristics including the shape of the ring cross-section, arc length, radius, and thickness. These include INTACs, Ferrara rings, Bisantis segments, and the Myoring. The INTACs are the only intrastromal segment rings approved by the FDA in the United States. This segment has a hexagonal cross-section and is created with either a conical or oval longitudinal section. The ring thickness and proximity to the visual axis are two parameters that most directly induce the refractive changes created by ICRS insertion. In normal human corneal tissue, there is an almost linear relationship between the thickness of the segment and the degree of corneal flattening [33, 34].
Clinical and histologic studies of human corneas following ICRS show lamellar channel deposits of intracellular lipids around the segments that currently have unknown significance. Histologic evaluation of corneal stroma in rabbits following ICRS by Twa et al. [35] demonstrated new keratocyte activation, intracellular lipid accumulation, and new collagen formation.
Trauma-Associated Complications with ICRS
There are reports of patients with ICRS and history of microtrauma who present with intermittent worsening of vision that improve with rubbing the eye. On slit lamp examination, it was noted that the intrastromal ring segments migrated, leading to superimposed distal segments. Surgical repositioning and ring segment fixation can be performed with 10-0 nylon suture to the cornea for stabilization of the intrastromal ring segments. In this case, a complete circular tunnel created by the femtosecond laser technique may have provided more opportunity for ring migration and override [36]. Other traumatic complications could include extruded segments and segments penetrating into the anterior chamber.
Incisional Refractive Surgery: Examination
Evaluation requires a complete general and ophthalmic examination. Similar to other types of eye-related trauma, when approaching a patient with a history of refractive surgery, it is important to differentiate a penetrating wound (passes into a structure) from a perforating wound (passes through a structure). Vigilant examination with a narrow slit beam can help determine the depth of the incision and/or injury. If not obvious, a Seidel test with fluorescein can confirm one’s suspicion for a perforating corneal injury (Fig. 4.6). In addition, patients with ICRS should be examined for malposition including overriding segments, extruded segments, and segments penetrating into the anterior chamber.


Fig. 4.6
Slit lamp photograph of Seidel positive wound. Note the white arrows indicating the wound. Photograph courtesy of David Heidemann, MD
Incisional Refractive Surgery: Treatment
For most wound ruptures, definitive treatment will require closure with sutures, typically 10-0 nylon (Fig. 4.7). At times, the rupture may be small and self-sealed. In these cases, a bandage contact lens (BCL) may be considered along with aqueous suppressants. A BCL may also be used to treat a partial thickness laceration/dehiscence.


Fig. 4.7
External photograph of repaired radial keratotomy incisions with 10-0 nylon following rupture. Photograph courtesy of Lori Stec, MD
As the cornea is the primary refracting component of the eye, wound repair can often induce decreased visual acuity secondary to scar formation and irregular astigmatism. Keratometry and corneal topography may help evaluate for irregular astigmatism. Rigid gas permeable (RGP) contact lenses can often provide improved visual acuity by correcting irregular astigmatism. This can be combined with a soft prosthetic contact lens for cosmesis (piggyback contact lens system).
Once the RK cornea has sustained injury and ruptured, some have advocated that suture removal should be avoided after the initial repair, especially if the sutures are not causing irregular astigmatism. Sony [37] reported a repeat dehiscence of 18-year-old RK incisions two days following suture removal 14 months after the initial wound repair.
As a broad spectrum of microorganisms have been found to cause incisional keratitis, management should include initial cultures and aggressive treatment with broad spectrum anti-microbials until the offending microorganism is identified.
Laser Refractive Surgery
Laser Refractive Surgery: Procedures
Photorefractive Keratectomy (PRK)
Introduction
PRK utilizes the excimer laser to change the shape of the cornea similar to laser-assisted in situ keratomileusis (LASIK); however, in PRK, there is no flap creation. PRK emerged after RK as a new way to change the refractive state of the cornea without the disadvantages that can accompany RK incisions, including the potential susceptibility to trauma. While the final visual acuity results of PRK surgery are comparable to LASIK outcomes, PRK visual recovery is initially slower because it takes weeks for the epithelial layer to regenerate and form a stable refractive corneal surface. The entire thickness of the underlying stroma is available for treatment because a flap including epithelium and anterior stroma is not removed. Phototherapeutic keratectomy (PTK) is practically the same technique as PRK but typically refers to using the excimer laser for treatment of other corneal diseases (instead of refractive indications).
Procedure
Following topical anesthetic, the corneal epithelium in the ablation zone is first removed to allow for accurate ablation of the stromal tissue. This is often accomplished by placing a circular well over the ablation zone and filling it with 20% absolute alcohol for 20–45 s. Once the epithelium is loosened, it can be removed with a weck-cell sponge. Other debridement techniques include using a sharp blade, blunt spatula, rotary brush, or transepithelial ablation by the excimer laser. The excimer laser can then be applied to the exposed corneal stroma. Following the treatment, a BCL is then placed over the cornea. Topical antibiotic, NSAIDs, and corticosteroid are administered postoperatively. Visual acuity slowly improves after the epithelium heals and the refractive state stabilizes 3–6 months following surgery.
Structural Effects of PRK on the Cornea
Several studies have looked at whether PRK can potentially weaken the cornea structurally and increase the likelihood of damage with trauma. Campos et al. [38] tested a 10-diopter PRK treatment in porcine eyes with lateral compression blunt trauma and found that these post-PRK eyes behaved similarly to unoperated eyes. The trauma was delivered by placing the eyes in a bench press device which applied gradually increasing lateral compression. The thinnest portion of the sclera was the site of rupture in almost all post-PRK and nonoperated eyes. In contrast, post-RK eyes demonstrated structural weakness relative to the thinnest sclera with ruptures at the RK incisions. Lower average pressure was needed to rupture post-RK eyes compared to post-PRK eyes. Interestingly, porcine eyes undergoing deep PTK ablations developed corneal ruptures when corneal thickness was reduced to 0.45 mm from an average 0.90 mm (removal of >40% stromal thickness) [38]. This greatly exceeds the required ablation for most clinical refractive scenarios.
Similarly, Burnstein et al. [39] studied human eye-bank eyes and subjected them to PRK treatment ranging between 6 and 54 diopters. They demonstrated that PRK does not weaken the corneal integrity with ablation depths commonly used in the clinical setting. In their experiment, IOP was increased gradually using nitrogen gas in human eye-bank eyes until the globes ruptured. Rupture only occurred at the ablation site with ablations greater than 37% of corneal thickness and pressures greater than 65 psi [39]. Most clinical PRK protocols do not exceed 15% of corneal thickness. Using a similar pressurizing system, Alcaraz et al. [40] looked at wound integrity following excimer laser photoablation compared to RK. There was leakage at the incision only in the RK group and burst pressures were significantly lower in the RK group. Galler [41] found that PRK in porcine eyes does not affect ocular integrity after blunt trauma when subjected to a squash ball impacting the cornea axially.
Trauma-Associated Complications with PRK
There are no reported cases of isolated globe rupture after PRK treatment to date. Artola [42] reported a case of ruptured RK incisions following blunt trauma 6 years after combined RK/PRK. Four of the eight radial incisions ruptured with extrusion of intraocular tissues. They believed that PRK carried out on a thin cornea already weakened by RK may have compromised the structural integrity of the eye.
Laser-Assisted in Situ Keratomileusis (LASIK)
Introduction
LASIK, first approved in 1999, is now one of the most commonly performed refractive procedures to correct various degrees of myopia, hyperopia, and astigmatism. LASIK has replaced many other procedures because of its relative safety, efficacy, quick visual recovery, and visual stability.
Procedure
In this procedure, a corneal suction ring is first applied to the topically anesthetized eye. Traditionally, a microkeratome was used to create a 300° flap of corneal tissue by creating an incision along the corneal stromal lamellae to separate the epithelium, Bowman’s layer, and the anterior stroma from the rest of the remaining posterior stroma. More recently, the femtosecond laser has been commonly used to create this flap (Fig. 4.8). The laser has allowed surgeons to optimize the flap dimensions and create a more precise flap. This flap is then reflected back at the hinge, the 60° that was not incised, to reveal the stroma.


Fig. 4.8
Slit lamp photograph of a normal LASIK flap created by a femtosecond laser. Note arrow indicating the flap edge
Next, the excimer laser is used to reshape the residual corneal stromal bed using precisely guided pulses to disrupt the stromal carbon–carbon bonds. This ultraviolet laser energy can be titrated to an exact depth, which makes it ideal for manipulating the surface of the stroma. Once the stroma has been ablated to achieve the intended optical shift, the corneal flap is then refloated and repositioned on the stromal bed. Healing then takes place over the next several days to weeks.
Structural Effects of LASIK on the Cornea
The LASIK flaps created by the microkeratome and femtosecond laser have been studied histologically. After the first day following LASIK, the epithelial layer starts to regenerate at the flap margin. Over the next several weeks, a collagenous scar forms at the LASIK flap edge between the severed edges of Bowman’s layer.
There are two types of reparative stromal wound healing responses associated with LASIK surgery: a hypercellular fibrotic scar at the flap-wound margin (this holds the corneal flap in place) and a weaker transparent, hypocellular primitive scar in central/paracentral areas of the lamellar wound [43]. The hypocellular primitive scar develops without reconnection of severed collagen lamellae, and the scar regains only 2.4% of normal stromal strength without evidence of remodeling even 6.5 years after LASIK surgery [44]. The LASIK flap-wound margin heals in a more complete manner, averaging 28.1% of normal corneal stromal strength [43–46].
Wound healing appears to only occur superficially (in the epithelial cell layers) as the collagen lamellae do not bond with each other in the area of the primitive scar. This can explain why the biomechanical properties of the post-LASIK cornea are primarily determined by the residual stromal bed with the LASIK flap only contributing minimally to the tectonic corneal strength and stability [47, 48]. Peacock et al. [49] studied the force needed to cause ocular rupture in RK, LASIK, automated lamellar keratoplasty (ALK), and PRK cadaver eyes. All operated eyes required less energy to rupture as compared with that of control eyes; however, unlike RK eyes, the level of energy required to rupture PRK and LASIK eyes were much greater [49].
Due to this wound healing, the interface between the flap and underlying stromal bed interface can be detected several years after its creation, with a clear absence of reconnection between adjacent severed lamellae despite close approximation of the flap-stromal interface. This lack of reconnection between the flap-stromal interface is what contributes to traumatic dislocations of the LASIK flap even many years following surgery. Two rabbit model studies have compared the force required to dislodge LASIK flaps at 1, 3 months, and at 75 days [50]. In both studies, femtosecond flaps were significantly stronger than their microkeratome counterparts. That being said, the flaps do take a substantial amount of force to create a dehiscence. This has been demonstrated by numerous authors; for example, the force from a cockpit ejection seat simulator was insufficient to dislodge microkeratome LASIK flaps 1 month after surgery in a rabbit model [51]. Additionally, subjecting rabbit eyes to high-speed wind trauma as early as 24 h after LASIK found microkeratome flaps to be stable, probably as a combined result of epithelial bridging across the flap edge and the osmotic gradient across the interface [52].
Mousavi et al. [53] simulated the effect of a blunt foreign body in PRK and LASIK eye models using finite element analysis. They showed that rupture occurs at higher velocities in the corneal stromal bed of the LASIK model than in the cornea of the PRK model [53]. In the clinical range of application of the excimer laser for PRK and LASIK (corneal thickness 500–350 μm), the required velocity for corneal rupture decreases nonlinearly with reduced corneal thickness. This reflects the reduction in impact force that occurs when the force is transferred from the upper separated corneal flap to the lower stromal bed in the LASIK model.
Trauma-Associated Complications with LASIK
Flap Dislocation/Displacement
While it is most vulnerable for spontaneous dislocation during the period immediately following surgery, the corneal flap created in LASIK is at a risk of dislocation at any time following ocular trauma secondary to the limited healing at the flap-stromal interface (Fig. 4.9). Following trauma with a substantial force, patients may present with either a dislocated corneal flap or a completely detached flap. Traumatic flap dislocation may occur in up to 1% of patients (and can predispose patients to a host of other subsequent complications, such as epithelial ingrowth, diffuse lamellar keratitis, infectious keratitis, and interface foreign bodies [54].


Fig. 4.9
Slit lamp photograph of a dislocated LASIK flap. Note the arrow indicating the dislocated flap edge. Photograph courtesy of Aparna Shah, MD
Dislocation or subluxation of the flap is possible, even many years after the procedure is performed. Cases of dislocation up to 14 years after the initial LASIK procedure have been reported. Because of the absence of reconnection between adjacent severed lamellae at the flap-stromal interface, a flap dislocation, theoretically, will always be a risk [55].
Flap Avulsion and Tears
Compared to flap dislocations, a flap avulsion is much less common [56]. Trauma to completely detach a flap likely requires substantial shearing force at a particular angle to sever the hinge. Because of the resultant irregular astigmatism, RGP lens fitting may be necessary to optimize visual acuity. Loss of the flap may induce a refractive error, reported up to 6 diopters of a myopic shift. PRK with Mitomycin C can be a potential option to treat this refractive error [57].
If not completely avulsed, a flap tear can result in the flap being flipped either anteriorly or posteriorly relative to the remaining intact portion of the flap [58]. This has been associated with epithelial ingrowth at the areas of exposed stroma (Fig. 4.10). The exposed stromal bed may also develop opacities that are visually significant. Even with slit lamp examination, there may be difficulty identifying the proper orientation of a flipped flap. Anterior segment optical coherence tomography (OCT) can be valuable in these situations. Its high magnification and resolution can identify the orientation of the flap and can reveal the location of epithelial ingrowth.


Fig. 4.10
Slit lamp photograph of a partially amputated LASIK flap with re-epithelialization over the bare stroma. Note the black arrow indicating the edge of the remaining flap. Note also the white arrow indicating the ridge of epithelium growing over the bare stroma. Photograph courtesy of Marius Miron
Corneal Penetrating/Perforating Injuries
When corneal injuries occur in patients with a history of LASIK, one must evaluate how both the flap and the stromal bed have been affected. Careful slit lamp examination can help determine partial and full-thickness injuries. The Seidel test may be falsely negative in a corneal perforation if the flap is slightly offset from the stromal bed because the aqueous fluid may accumulate in the interface and may not be apparent on the epithelial surface (see next section: Interface Fluid Syndrome).
Interface Fluid Syndrome (or Pressure-Induced Stromal Keratopathy [PISK])
Lamellar interface fluid accumulation is a well-described LASIK complication described by Lyle and Jin that is typically seen following corticosteroid-related IOP elevation [59]. Here, fluid collects in the interface potential space as aqueous humor diffuses into the corneal stroma in the setting of compromised endothelium. Interface fluid syndrome has also been reported following blunt, penetrating/perforating, and barotrauma [60–62]. The time from ocular trauma to development of interface fluid syndrome is much shorter (~2–3 days) than that associated with corticosteroids (typically after 10–21 days of use). Anterior segment OCT may prove valuable as the interface fluid may masquerade as interface hyperreflection (haze)/keratitis on slit lamp examination. Bushley et al. [62] reported a case of a paracentral full-thickness corneal laceration in an eye with prior LASIK that developed microcystic edema confined to the flap and fluid in the interface following initial laceration repair with 10-0 nylon. An additional 10-0 nylon suture was placed in the area of presumed posterior wound gape, and there was resolution of the microcystic edema and interface fluid within 24 h. In blunt trauma, as there is no direct pathway for fluid to sequester in the interface, it has been hypothesized that direct traumatic force to the flap and anterior chamber inflammation contribute to the formation of interface fluid (Fig. 4.11). For interface fluid syndrome associated with blunt trauma, treatment with anti-glaucoma medications and corticosteroids often leads to resolution.


Fig. 4.11
Slit lamp photograph of an intact LASIK flap with an underlying stromal perforation. The red arrow indicates a flat anterior chamber. The black arrow indicates the intact LASIK flap. The white arrow indicates the perforation site. Between the black and white arrows demonstrates fluid within the interface. Photograph courtesy of David Heidemann, MD
Diffuse Lamellar Keratitis
Diffuse lamellar keratitis (DLK) is a well-known complication of LASIK, with overall incidence in most published series reported as less than 3% [63]. It was first described by Robert Maddox as “Sands of Sahara Syndrome,” [64] and it was later termed “DLK” by Smith and Maloney [65]. DLK classically develops 2–6 days following LASIK (Fig. 4.12a, b) and typically resolves 5–8 days after the initiation of appropriate therapy (corticosteroids with or without lifting the flap) [65]. Although more rare, there have also been reported cases of late-onset DLK ranging from months to years after surgery with trauma being one of the more common triggering etiologies. This demonstrates the persistence of the potential space made upon flap creation.


Fig. 4.12
Slit lamp photograph of a diffuse lamellar keratitis (DLK). There LASIK flap demonstrating a ground glass appearance consistent with inflammation confined to the flap. a Diffuse illumination redemonstrating the ground glass appearance. b Slit beam illumination inflammation confined to LASIK
Traumatic epithelial defects, blunt trauma [66], flap dislocation [67], metallic foreign body [68], and surgical trauma (including conductive keratoplasty [69], epithelial debridement [70], and cross-linking) [71] have all been associated with late-onset DLK. The etiology of late-onset DLK is not well understood. While early-onset DLK has been thought to be an inflammatory reaction to certain exogenous exposures, late-onset cases may illustrate how the interface continues to remain unhealed many years after lamellar surgery, resulting in a potential space for endogenous inflammatory debris/cells to collect in this space [72].
Epithelial Ingrowth
Epithelial ingrowth is a complication in which epithelial cells in the peripheral flap interface advance inwards along the lamellar interface (Fig. 4.13). It can be an early postoperative complication or can accompany trauma involving full or partial dehiscence of the corneal flap [73]. Epithelial ingrowth typically advances from the periphery where the flap was disrupted; however, any corneal penetrating/perforating injury along the flap can be associated with epithelial ingrowth in that area.


Fig. 4.13
Slit lamp photograph with direct illumination shows epithelial ingrowth at the edge of the LASIK flap between 9 and 10 o’clock growing towards the central cornea. Note the black area is denoting the area of epithelial ingrowth
Flap Folds
Flap folds, otherwise known as striae, can appear within the first 24 h, but most occur within the first week following LASIK. They are classified as either macrostriae or microstriae. Macrostriae represent full-thickness folds and microstriae represent partial thickness folds. There have been cases of striae reported without corneal flap dislocation following trauma years after the initial photorefractive surgery [74]. It is important to consider the formation of striae when examining LASIK patients with ocular trauma even in the absence of flap slipping, subluxation, or dislocation. Flap striae tend to occur more frequently in cases of delayed presentations following trauma.
Histological studies with rabbit corneas that have undergone LASIK and developed corneal flap folds have demonstrated that the formation of macrostriae leads to neutrophil infiltration around the incisional area, as well as irregular epithelial growth due to the disruption in the epithelial basement membrane [75]. This inflammatory reaction and asymmetrical hyperplasia fixes the folds into place, which leads to persistent visual deficits.
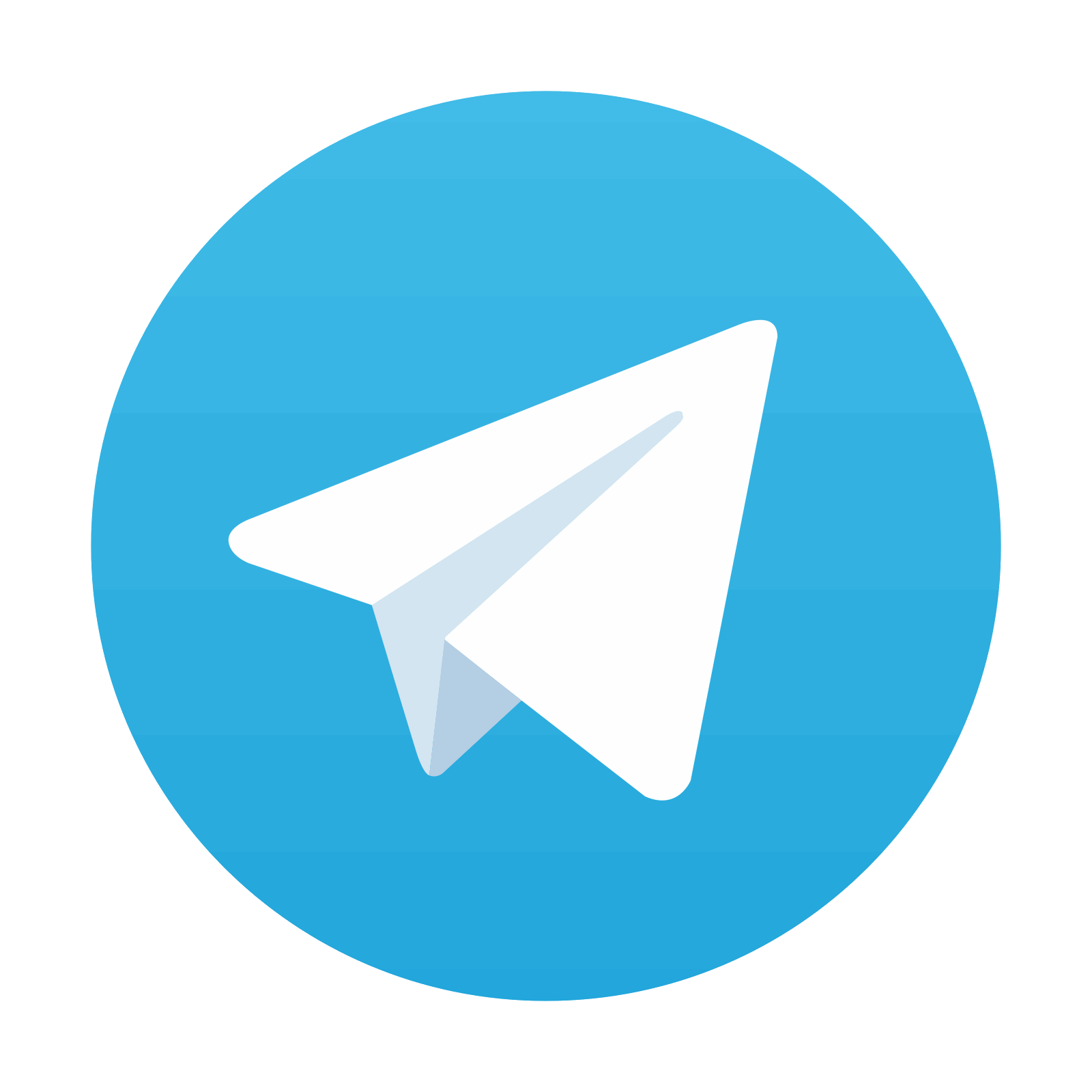
Stay updated, free articles. Join our Telegram channel

Full access? Get Clinical Tree
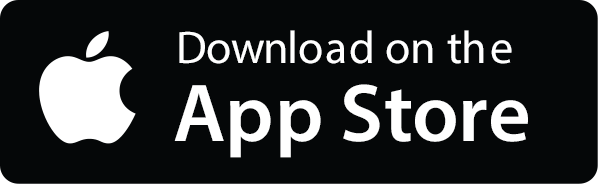
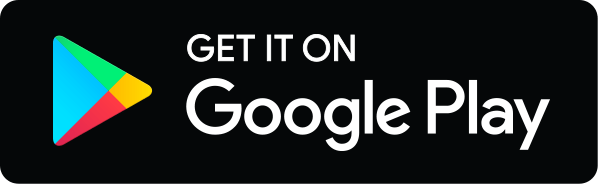
