Photodynamic Therapy
Lucienne Collet
Gema Ramírez
Rafael Cortez
Virgil D. Alfaro III
John B. Kerrison
Monica Rodriguez-Fontal
INTRODUCTION
Age-related macular degeneration (AMD) is the leading cause of legal blindness or severe vision loss in patients older than 60 years in developed countries (1). The management of AMD differs depending on whether it is exudative or nonexudative. The only proven treatment for the nonexudative type, comprising 85% of cases, is an antioxidant/mineral supplement which can slow the progression of the disease by 25% over 5 years (2). For the remaining 15% of the cases, laser treatment, thermal laser photocoagulation, submacular surgery, photodynamic therapy (PDT), and pharmacologic therapy are considered as treatment options.
In 1982, the Macular Photocoagulation Study (MPS) Group demonstrated the effectiveness of laser treatment for choroidal neovascularization (CNV), showing decreased risk of vision loss in these patients (3). Treatment failure occurred in at least 50% of cases resulting from the development of recurrent or CNV on the foveal edge of the laser scar (4). In 1995, the MPS reported that treatment of subfoveal CNV composed of both classic and occult neovascularization was not beneficial with respect to visual acuity and recurrent neovascularization (5).
PDT was first used in ophthalmology in 1994 (6). In 1996, several multicenter randomized clinical trials of PDT with verteporfin were initiated to evaluate its safety and efficacy in treating subfoveal CNV secondary to AMD. Today, the management of exudative AMD is constantly changing with new antiangiogenic therapies.
MECHANISM OF ACTION AND PHARMACOLOGY OF PHOTODYNAMIC THERAPY
PDT uses an intravenously injected photosensitizing drug that reaches the target tissue through the bloodstream. The photosensitizer is activated by low-intensity laser light and causes damage to choroidal neovascular tissue through a photochemical reaction. The maximum absorption spectrum of the photosensitizer determines the wavelength of the radiation. The light-dye interaction elevates the photosensitizer from its electronic ground state to a higher-level excited triplet state. The photosensitizer quickly returns to the ground state and transfers energy to other molecules, such as oxygen. Singlet oxygen and free radicals are formed and react with proteins, nucleic acids, and lipid membranes. The effective penetration depth of PDT is dependent upon the wavelength of the light. At 630 nm the effective penetration depth is 2 to 3 mm and increases to 5 to 7 mm at 700 to 800 nm (7).
The reactions that follow the formation of intravascular free radicals are classified as cellular, vascular, and immunologic (8). At the cellular level, the free radicals develop a cytotoxic effect by interacting with mitochondria, lysosomes, and other intracellular organelles (9), leading to apoptosis. At the vascular level, which is believed to be the main mechanism, the free radicals lead to destruction of the vascular endothelium. When the vascular endothelium is destroyed, the basal membrane is exposed and it interacts with blood and its components, triggering a platelet aggregation (9). After platelet activation, diverse mediators of inflammation such as thromboxane and histamine are released, thus leading to thrombosis of the vascular lumen and a closing of the vessels limited to the CNV with slight effect on the surrounding microcirculation (10, 11 and 12). The effectiveness and selectivity of PDT depends on the photosensitizer and the laser used. The ideal wavelength must coincide with the peak light absorption of the photosensitizer used (13). The photosensitizer diffuses into the bloodstream and adheres to the plasmatic low-density lipoproteins. These lipoproteins have abundant receptors in the cytoplasmic membranes of tumoral cells and vascular endothelium. After the photosensitizer associates with LDL, this complex is incorporated into the cell by endocytosis, and then it can be activated by a laser with the appropriate wavelength (14).
VERTEPORFIN
Verteporfin (Visudyne) is a tetrapyrrole derived from the benzoporphyrin, also called benzoporphyrin derivative monoacid ring A (BPD-MA). It is a potent second-generation light-activated drug. It is a chlorin-type molecule and exists as an equal mixture of two regioisomers, each of which consists of an enantiomeric pair that demonstrate similar pharmacologic activity in vitro and in vivo (15,16). Verteporfin has a molecular formula of C41H42N4O8 and a relative molecular weight of 718.81. Nowadays, it is the only photosensitizer approved by the U.S. Food and Drug Administration (FDA) for the treatment of CNV secondary to AMD and subfoveal CNV secondary to pathologic myopia.
Verteporfin has a long absorption wavelength with a strong absorption peak at 680 to 695 nm (15). It absorbs light efficiently at a wavelength of 689 nm (red light), which can penetrate a thin layer of blood, melanin, or fibrotic tissue. Light at this wavelength is not absorbed strongly by naturally present substances (17). The strongest absorption peak of verteporfin is at approximately 400 nm (blue light), but this wavelength is not clinically useful for treatment of CNV because it is the same as the absorption peak of oxyhemoglobin (17). The most suitable light source for use in Visudyne therapy is a nonthermal diode laser, operating at a wavelength of 689 ± 3 nm (17).
The regimen of Visudyne therapy established by the phase I/II studies and investigated in phase III placebo-controlled studies is as follows:
Visudyne dose: 6 mg/m2 body surface area (BSA)
Infusion rate: 3 mL/min
Duration of infusion: 10 min
Light application interval after start of Visudyne infusion: 15 min
Wavelength: 689 nm
Dose: 50 J/cm2
Intensity: 600 mW/cm2
Resulting duration of light application: 83 seconds
Closure of abnormal, leaking blood vessels occurs for approximately 6 to 12 weeks in most patients. Reperfusion is common and multiple treatments are often required.
INFLUENCE OF TREATMENT PARAMETERS ON SELECTIVITY OF VERTEPORFIN
Studies have been conducted to test the effect of changing dosimetry in order to avoid damage to the surrounding tissue (18). It has been observed that there is a significant photodynamic effect on the choroidal circulation with 50 J/cm2. This parameter was established as a guideline under treatment of age-related macular degeneration with photodynamic therapy (TAP) (19, 20, 21, 22 and 23). It has been demonstrated by indocyanine green (ICG) angiography that the TAP regimen regularly results in damage to the physiologic choroid, showing early and often persistent nonperfusion of the surrounding choroid (19,20). Histopathologic studies show a dose-dependent thrombosis of the choriocapillaris (21) and immunostaining reveals a reactive upregulation of vascular endothelial growth factor (VEGF) (22). Modification of treatment parameters with reduced fluence to 25 J/cm2 allows selective closure of the CNV and reduces the impact on the physiologic choroidal vasculature (18).
CLINICOPATHOLOGICAL FINDINGS AFTER PHOTODYNAMIC THERAPY
Histopathological findings of CNV 2 weeks to 3 months after PDT in neovascular AMD have been published (24, 25 and 26). In these studies, endothelial damage of the CNV was noted. Schnurrbusch, in an electron microscopic study of surgically extracted CNV, showed occluded vessels with thrombotic masses and ultrastructural damage of the neovascular endothelium 3 months after PDT (24). Ghazi also reported endothelial cell degeneration with platelet aggregation and thrombus formation on removed CNV specimens 4 weeks after PDT (25). In a more recent study, the histopathological examination of the excised CNV membranes was done 3 days after PDT (24). It revealed fibrovascular tissue with swollen and disintegrated endothelium. However, neither a red blood cell nor a platelet/fibrin clot was observed (24). Other studies have also reported a uniform occlusion of the healthy choriocapillaris 1 week following PDT in enucleated human eyes (27). The occlusion of the choriocapillaris, perhaps, is the explanation for the nonperfusion of CNV membranes in 3 days following verteporfin PDT.
Whether PDT-induced ischemia of the choriocapillaris influences the reactivation of the CNV by increasing the expression of factors contributing to new vessel formation is not known. The absence of thrombosis in the CNV by light microscopic examination, in the presence of fluorescein angiographically proven nonperfusion of the entire treated area, suggests that choroidal ischemia induces the closure of the CNV vessels in the early period following PDT. The disintegration of the endothelium of the CNV vessels may result in thrombosis in the weeks following treatment (28). Additionally, Tatar et al. studied 50 eyes that underwent removal of choroidal neovascular membranes, 20 of them having received PDT prior to removal. The study histologically revealed many collapsed vessels, damaged endothelial cells, and an occluded choriocapillary layer within the spot produced by the laser. There was intense VEGF synthesis especially prominent in retinal pigment epithelium (RPE) cells induced by hypoxia after PDT (29, 30, 31, 32 and 33). There was prominent inflammatory activity described in post PDT CNV (34). These findings seem to be an important angiogenic stimulus that may lead to increased vascular leakage and development of recurrent CNV (35).
IMMUNOHISTOLOGICAL EVALUATION OF CHOROIDAL NEOVASCULARIZATION POST PHOTODYNAMIC THERAPY
Analysis of vascularization and proliferative activity of surgically extracted CNV following PDT by immunodetection of characteristic markers (CD 34, CD 105, and Ki-67) showed that there is a neoangiogenetic mechanism that leads to the formation of new vessels observed in CNV even after 37 days post PDT (36). However, Schnurrbusch (24) and Moshfeghi (37) reported that new vessel formation does not occur until a minimum of 63 days after such an event.
ANGIOGRAPHIC FINDINGS AFTER PHOTODYNAMIC THERAPY
Fluorescein angiography in animal studies, early after PDT, demonstrated hypofluorescence with perfusion of retinal vessels. Also, ICG angiography demonstrated hypofluorescence in the area treated with PDT, although larger choroidal vessels were perfused. In animal studies, this hypofluorescence on ICG angiography resolved to some extent with follow-up but was still evident at 5 to 8 weeks after PDT. The hypofluorescence may be caused by PDT-induced choriocapillaris occlusion or retinal pigment swelling leading to blocked fluorescence. Follow-up at 2 to 5 weeks after PDT demonstrated staining of the irradiated CNV by ICG angiography and may represent staining of the remaining fibrovascular tissue (29).
OCT AFTER PHOTODYNAMIC THERAPY
Rogers et al. (38) studied the optical coherence tomography (OCT) characteristics following PDT in eyes treated for CNV secondary to AMD. They correlated it to fluorescein angiography findings and proposed an OCT classification system as follows:
In Stage I there is evidence of acute inflammatory response, confirmed at 1 hour and up to 1 week following PDT.
Fluorescein angiography revealed hyperfluorescence of both the CNV and treatment area, with later frames of the angiogram demonstrating increased leakage of fluorescein in the treatment zone. The OCT demonstrated increased accumulation of intraretinal fluid in a circular distribution delineating the treatment spot.
Fluorescein angiography revealed hyperfluorescence of both the CNV and treatment area, with later frames of the angiogram demonstrating increased leakage of fluorescein in the treatment zone. The OCT demonstrated increased accumulation of intraretinal fluid in a circular distribution delineating the treatment spot.
In Stage II there is resolution of subretinal fluid with choroidal hypoperfusion. Approximately 1 to 2 weeks following treatment, there is hypoperfusion of the CNV and choriocapillaris with a well-delineated area of hypofluorescence corresponding to the treatment spot on fluorescein angiography (FA). OCT exhibits resolution of subretinal fluid in the treatment area with the reestablishment of a normal appearing foveal contour. Stage II typically lasts up to 4 weeks or whenever choroidal reperfusion occurs.
In Stage III, there is reaccumulation of subretinal fluid with subretinal fibrosis. They observed that after the fourth week of treatment, reperfusion of the CNV is typically present on FA with variable degrees of leakage. The treatment spot is less visible on angiography, as the choriocapillaris is rapidly perfused. Intraretinal and subretinal fluid reaccumulates and early subretinal fibrosis becomes evident on OCT between the retina and underlying RPE. They propose that Stage III is subdivided into two separate stages: stage IIIa and IIIb, depending on the ratio of fluid to fibrosis on OCT. In stage IIIa retinal fluid to fibrosis ratio is higher, representing a more active neovascular process. In stage IIIb, the fibrotic component of the CNV is prominent with minimal intraretinal fluid on OCT. Stage IIIb lesions are relatively inactive due to the predominance of subretinal fibrosis with minimal intraretinal fluid, which still leak on fluorescein angiography.
In Stage IV, there is subretinal fibrosis with cystoid macular edema (CME). On FA, the borders of the lesion remain relatively fixed with staining of the fibrotic CNV. However, a component of active leakage in FA is present, which is defined on OCT as CME. The cysts are represented by hyporeflective black circular spaces in the retina. The involuting CNV is represented on the OCT as a highly reflective band merging with RPE/choriocapillaris layer (38).
ELECTRO-OCULOGRAPHY AFTER PHOTODYNAMIC THERAPY
Osner et al. found that the Arden ratio of the electro-oculogram (EOG) decreased 1 week after PDT and the differences were statistically significant. The decrease in Arden ratio persisted at the first month, as assessed by EOG recordings. PDT may affect the interaction between RPE and photoreceptors (39).
CLINICAL TRIALS
There are numerous controlled studies that employ PDT. They can be divided into Phase I/II dose-finding studies, where the dose parameters are established, and Phase III placebo-controlled studies that include TAP, verteporfin in photodynamic therapy (VIP), and verteporfin in the ocular histoplasmosis (VOH).
In order to determine an effective treatment regimen as well as the safety and efficacy of Visudyne therapy, a total of 1236 patients were included: 142 in the phase I/II dose-finding study, 609 in the TAP investigation, 459 in the VIP trial, and 26 in the VOH syndrome study.
DOSE-FINDING STUDY (PHASE I/II)
Dose Determination
Some experiments with thermal laser-induced neovascularization in primate eyes were conducted in order to determine the optimal parameters for effective closure of CNV with Visudyne therapy (14, 23, 30, 40, 41, 42, 43, 44, 45, 46, 47, 48, 49, 50, 51, 52, 53, 54, 55, 56 and 57). Maximal closure of CNV was achieved using Visudyne 0.375 mg/kg with light application 20 to 50 minutes after Visudyne injection at a light dose of 150 J/cm2 (light intensity 600 mW/cm2) (56). Lower doses of Visudyne were more selective for CNV, reduced damage to surrounding tissues, and permitted a shorter time interval between Visudyne infusion and light application (56). Light intensities of 300 or 600 mW/cm2 enabled shorter, more practical treatment times and were not accompanied by any apparent adverse effects (57). Light doses of 50 to 600 J/cm2 stopped fluorescein leakage from CNV, but doses of ≥400 J/cm2 were associated with unacceptable toxicity in normal retinal tissues (52). These parameters formed the basis for phase I/II dose-finding studies in patients with CNV.
Phase I/II dose-finding study was carried out in patients with subfoveal CNV (30, 31 and 32). It was a nonrandomized, dosefinding study that was conducted at a total of four centers in Europe and North America. It included 142 patients with subfoveal CNV secondary to AMD (n = 128), pathologic myopia (n = 10), ocular histoplasmosis syndrome (OHS) (n = 1), angioid streaks (n = 1), and idiopathic causes (n = 1). All of them had evidence of classic CNV and were not eligible for laser photocoagulation.
All patients received one of five treatment regimens and were monitored for 12 weeks. Within each regimen, light at 689 nm (intensity 600 mW/cm2) was increased up to a maximum of 150 J/cm2; each light dose was tested on three patients, and the dose was only increased if these patients did not experience adverse events. The maximum tolerated light dose was below 150 J/cm2 and the minimum effective light dose was greater than 25 J/cm2
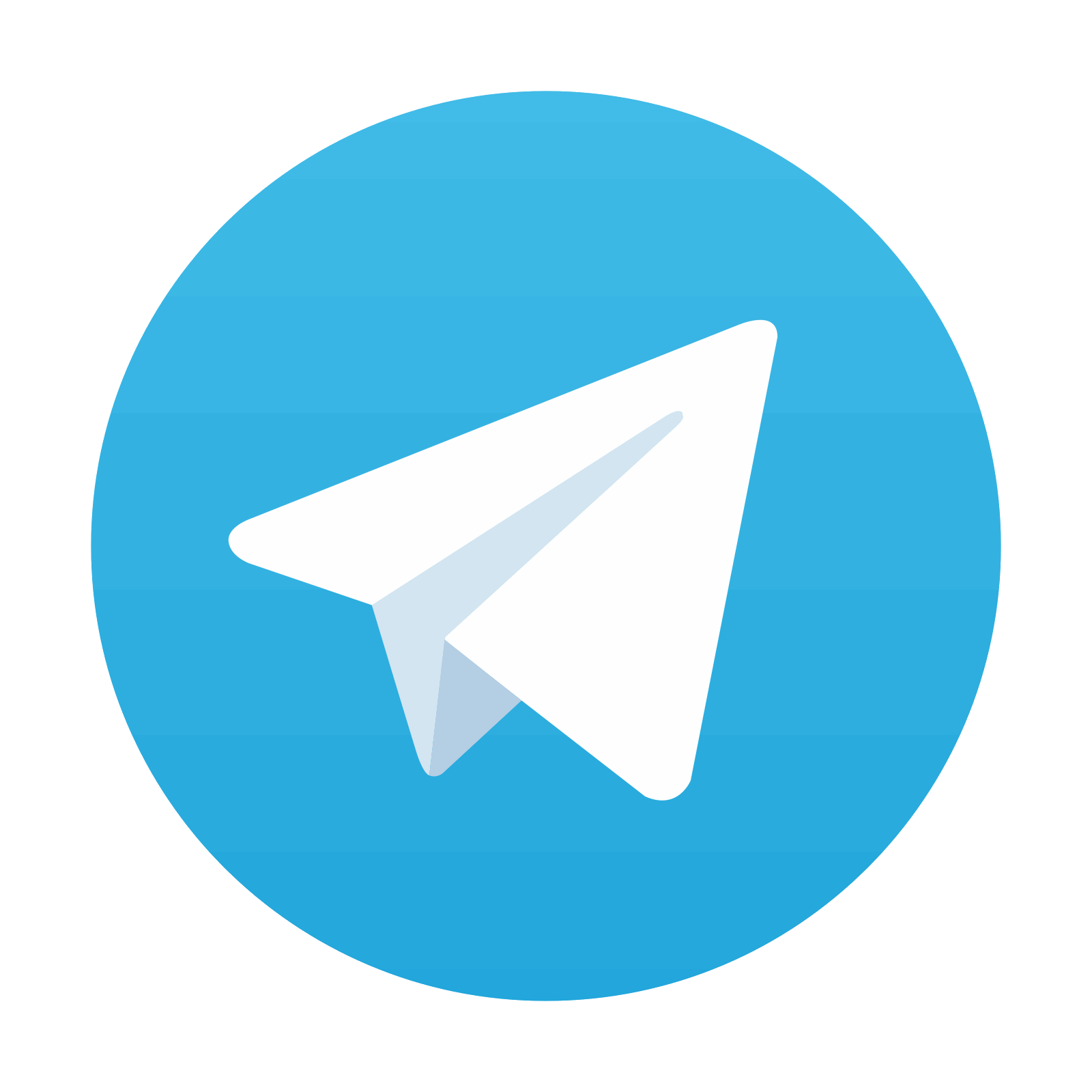
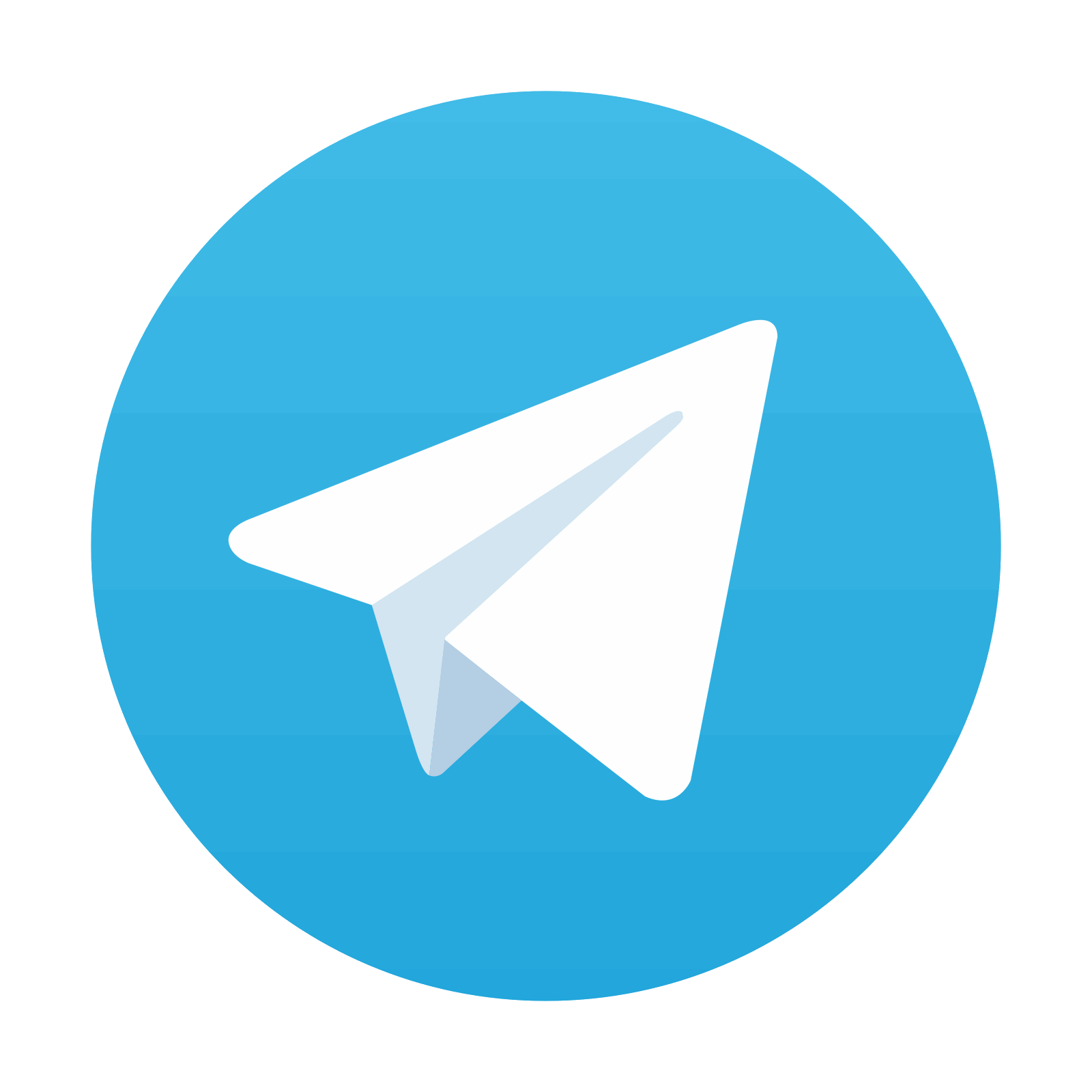
Stay updated, free articles. Join our Telegram channel

Full access? Get Clinical Tree
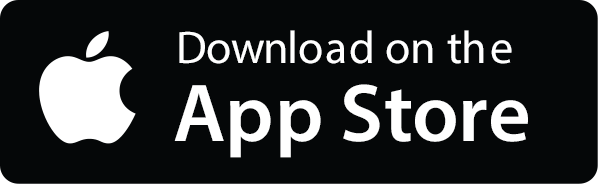
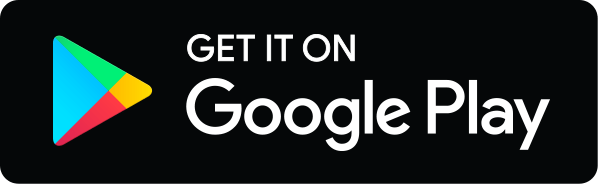
