20
Pharmacotherapy of Diabetic Retinopathy
Yoshihiro Yonekawa
Aziz A. Khanifar
Donald J. D’Amico
R.V. Paul Chan
Diabetes mellitus (DM) is a major cause of morbidity and mortality throughout the world. Over 170 million people are currently affected, and the prevalence is projected to more than double by 2030 (1). Diabetic retinopathy (DR) is the leading cause of blindness among working-age adults in the United States, and annually blinds over 12,000 patients (2). Similar to diabetic nephropathy and neuropathy, DR is a microvascular complication of both type 1 and type 2 diabetes. After 20 years of diagnosis, nearly all patients with type 1 and approximately 60% with type 2 DM have some degree of DR (3, 4). DR is broadly categorized into either nonproliferative (NPDR) or proliferative DR (PDR) (5), depending on the presence of retinal fibrovascular proliferation.
Historically, the mainstays of DR treatment are glucose, blood pressure, and cholesterol control, combined with photocoagulation and/or vitrectomy as necessary. However, recent advances in our understanding of the molecular mechanisms behind the pathogenesis of DR have catalyzed the development of pharmacologic therapies such as intravitreal corticosteroids and antivascular endothelial growth factor (VEGF) agents. Many other exciting pharmacological developments have taken place as well.
PATHOPHYSIOLOGY
Vascular basement membrane thickening, an early morphologic feature of DR (6), may cause both filtration defects and dysfunction of cell proliferation and differentiation (7, 8). The loss of intramural capillary pericytes is another early histologic hallmark (9, 10). Pericyte loss is thought to weaken the capillary wall, leading to microaneurysms, which are often the first clinically visible signs of DR.
Microaneurysms are hypercellular saccular outpouchings of the capillary wall (11), and an increase in their number is associated with progression of retinopathy (12, 13). Microaneurysms cause disruption of the blood-retinal barrier, leading to capillary permeability, resulting in intraretinal and subretinal fluid accumulation. The fluid tends to collect in the macular area, causing diabetic macular edema (DME). Increased expression of VEGF in regions with relative hypoxia and inflammatory factors also play a role in the development of DME in addition to fibrovascular proliferative disease. Severity of retinopathy is directly correlated with the likelihood of developing DME and retinal neovascularization.
DME can be focal or diffuse, and the pathophysiology, clinical appearance, and treatment modalities are different. Hard exudates are common findings in DME that correlate with serum lipid levels (14–17). The Early Treatment Diabetic Retinopathy Study (ETDRS) defined clinically significant macular edema (CSME) as thickening of the retina within 500 µm of the center of the fovea, hard exudates within 500 µm of the fovea associated with thickening of the adjacent retina, or retinal thickening of at least one disc diameter in size, within one disc diameter of the foveal center (18).
Retinal capillary occlusion causes increasing ischemia, which can lead to the development of cotton-wool spots, retinal hemorrhages, venous beading, and intraretinal microvascular abnormalities (IRMA). Retinal ischemia upregulates signaling molecules such as VEGF that promote fibrovascular proliferation, leading to PDR, which can cause significant visual loss by vitreous hemorrhage and/or tractional or combined tractional-rhegmatogenous retinal detachment. Neovascularization of the iris (NVI) may also occur as a result of retinal ischemia.
METABOLIC CONTROL
Insulin and Hypoglycemic Agents
Pharmacologic primary and secondary interventions are essential in lowering the systemic risk factors for developing DR. Currently, the most effective method of preventing and slowing the progression of diabetic retinopathy is tight glycemic control. The Diabetes Control and Complications Trial (DCCT) (19–36) showed that in comparison to conventional insulin therapy, intensive glucose monitoring and aggressive insulin therapy to maintain strict glycemic control lowered the risk of developing DR in type 1 diabetics with no baseline retinopathy by 76% (95% confidence interval or CI, 62 to 85). Notably, intensive therapy lowered the risks of nephropathy and neuropathy as well. The DCCT cohort was followed by the Epidemiology of Diabetes Interventions and Complications (EDIC) study (20), which showed that former tight glucose control provided continued benefits after seven years.
The U.K. Prospective Diabetes Study (UKPDS) (21) demonstrated that in type 2 diabetics, intensive treatment with sulfonylureas (chlorpropamide, glibenclamide, or glipizide) or insulin had a protective relative risk of 0.79 (p ∇ 0.015) for progression of DR, and 0.71 (p ∇ 0.0031) for requiring retinal photocoagulation.
The American Diabetes Association currently recommends maintaining a hemoglobin A1c of <7.0%, preprandial capillary plasma glucose of 70 to 130 mg/dL, and peak postprandial capillary plasma glucose of <180 mg/dL for nonpregnant adult diabetics (22).The main concern of intensive glycemic control is hypoglycemic episodes, which were more common in the intensive treatment arms of both the DCCT and UKPDS.
ANTIHYPERTENSIVES
Hypertension is associated with the development and progression of DR. The UKPDS randomized 3867 type 2 diabetics to tight (aiming for <150/85), or milder blood pressure control (<180/105) with captopril or atenolol (23). The tight control group had a 34% (99% CI, 11 to 50) risk reduction of DR progression, and a 47% (99% CI, 7 to 70) risk reduction of visual acuity deterioration by three lines. Deaths related to diabetes and stroke were also reduced (24).
In 2008, the Diabetic Retinopathy Candesartan Trials (DIRECT) reported that although candesartan reduced the incidence of new DR by 18% (p ∇ 0.051) in type 1 diabetics, there was no effect on DR progression for those with baseline retinopathy (25). However, the Renin-Angiotensin System Study (RASS) published in July of 2009 indicated that enalapril and losartan reduced the odds of early DR progression in type 1 diabetics by 65% (p ∇ 0.02) and 70% (p ∇ 0.008), respectively (26). These effects appear to be independent of blood pressure changes (26, 27).
ANTILIPIDS
The Wisconsin Epidemiologic Study of Diabetic Retinopathy (WESDR) (14) and the ETDRS (15) both found associations between serum lipid levels and retinal hard exudates. Lipid control may be beneficial in DR because visual acuity loss correlates with the degree of hard exudates, and permanent retinal damage can be caused by subretinal fibrosis (28).
Preliminary studies suggested the use of clofibrate to be beneficial (16, 17). The Fenofibrate Intervention and Event Lowering in Diabetes (FIELD) study (29) recently demonstrated that fenofibrate reduces the rate of first laser therapy for DR or DME, from 4.9% of patients on placebo to 3.4% of patients on fenofibrate (p ∇ 0.0002), but without an overall effect on the progression of DR (30). Statins have also been shown to improve DME in preliminary studies (31–33). Randomized clinical trials (RCTs) that are underway include the Atorvastatin Study for Prevention of Coronary Heart Disease Endpoints in Non-Insulin-Dependent Diabetes Mellitus (ASPEN) (34), and the Action to Control Cardiovascular Risk in Diabetes Eye (ACCORD-EYE) studies (35).
ANTIPLATELETS
Platelet microthrombi have been identified in retinal capillaries of diabetics, and have been proposed to be a possible mechanism for the capillary occlusions resulting in retinal ischemia (36, 37). The Dipyridamole Aspirin Microangiopathy of Diabetes (DAMAD) study randomized patients with mild DR to aspirin alone, aspirin combined with dipyridamole, or placebo. The treatment arms developed fewer microaneurysms on fluorescein angiograms, but there was little difference in visual acuity and ophthalmoscopy endpoints (38). Similarly, the Ticlopidine Microangiopathy of Diabetes study (TIMAD) showed that while ticlopidine slowed the formation of microaneurysms, there were no clinical benefits (39). The ETDRS concluded that aspirin therapy does not have clinical effects in DR (40), but there are no ocular contraindications (41) to taking aspirin for cardiovascular or other conditions (42).
The Steno-2 study examined the effects of multifactorial intensive therapy consisting of tight glucose control combined with renin-angiotensin system blockers, lipid-lowering drugs, and aspirin (43). There was a 43% risk reduction of DR progression (p ∇ 0.01), and a 55% risk reduction in requiring laser treatment for PDR or DME (p ∇ 0.02). However, multifactorial treatment of systemic risk factors was still insufficient to prevent DR progression in many patients. This highlights the importance of targeted therapies, discussed below, to be administered in conjunction with optimal metabolic control and current ophthalmic therapies.
ALTERATION OF BIOCHEMICAL PATHWAYS
Aldose Reductase
Several biochemical mechanisms have been proposed as links between hyperglycemia and diabetic microvascular complications (19, 44). Aldose reductase is the first enzyme in the polyol pathway that catalyzes the nicotinamide adenine dinucleotide phosphate (NADPH) – dependent reduction of aldose sugars into sugar alcohols. Hyperglycemia activates the pathway and glucose becomes reduced to sorbitol, which is thought to cause osmotic stress. Aldose reductase’s NADPH-consuming reactions also may cause oxidative damage by decreasing the NADPH available for glutathione reductase (45).
Clinical trials of aldose reductase inhibitors (ARIs), however, have not been promising. Data from the Sorbinil Retinopathy Trial (SRT) (46), a Phase III RCT of 497 insulin-dependent diabetics, showed no significant difference in the progression of retinopathy between subjects randomized to sorbinil or placebo. Subsequent trials of other ARIs such as zenarestat, tolrestat, and lidorestat, were prematurely halted due to toxicities (47–49).
NONENZYMATIC GLYCATION
Advanced glycation end-products (AGEs) are found in retinal vessels (50) and renal glomeruli of diabetic patients (51). Glycation of proteins alters their functions, causing them to bind to AGE receptors on endothelial cells and macrophages, which induces receptor mediated production of reactive oxygen species, proinflammatory and procoagulative molecules, growth factors, and transcription factors that cause pathologic gene expression (45). Studies suggest that AGE receptors also promote capillary wall hyperpermeability by inducing VEGF expression (52).
Aminoguanidine (Pimagedine) is a hydrazine derivative that binds AGE precursors to decrease AGE formation (53). The efficacy and safety of aminoguanidine against DR is currently controversial (54, 55). Another anti-AGE drug, alagebrium chloride (ALT-711), is being investigated in diabetic macrovascular disease (56–58).
Protein Kinase C
Protein Kinase C (PKC) is a family of serine-threonine kinases (59) that appear to mediate vascular contractility, hemodynamics, and cellular proliferation to modulate diabetic microvascular disease (60, 61). Hyperglycemia induces the synthesis of diacylglycerol (DAG), which in turn activates PKC. The β isoform has been linked most strongly with diabetes. Animal models have demonstrated that PKC-β increases retinal vascular permeability and neovascularization (62–64), most likely by involving VEGF (64, 65).
Staurosporin (PKC412) is a nonselective PKC inhibitor that also inhibits VEGF receptors 1 and 2, the Platlet-derived Growth Factor (PDGF) receptor, and stem cell factor receptor. In a Phase I/II RCT of 141 patients with DME, oral staurosporin decreased retinal thickening (p ∇ 0.032) and slightly improved (4.36 letters; p ∇ 0.007) visual acuity at 3 months (66), but further studies were abandoned due to gastrointestinal side effects and hepatotoxicity.
Ruboxistaurin (RBX/LY333531) is a selective inhibitor of PKC-β (61). The PKC-β Inhibitor Diabetic Retinopathy Study (PKC-DRS) randomized 252 subjects with moderately severe to severe NPDR to placebo or RBX (67). Doubling of the visual angle was delayed with 32 mg/day of RBX (p ∇ 0.012), but there was no difference in the progression of NPDR to PDR. In PKC-DRS 2 (68), the follow up Phase III study with 685 subjects and 36 to 42 months of follow-up, the relative risk reduction of moderate visual loss was 40% compared to placebo (p ∇ 0.034). RBX reduced initial photocoagulation in eyes that had not received laser treatment before baseline (p ∇ 0.008), and decreased the progression of DME to the center-involved stage (p ∇ 0.003), but there was no difference in the composite end point of DR progression. Notably, unlike staurosporin, RBX had minimal side effects.
The PKC-DME study was another Phase III RCT with 686 subjects, and examined RBX with DME progression or requirement of laser treatment as primary end points, but found no composite benefit (69). The US FDA approval of RBX for DME is currently pending on the results of further trials (70, 71).
CORTICOSTEROIDS
Intravitreal Triamcinolone
Laser treatment is currently the first line therapy for DME, and can halve the risk for visual loss in eyes with focal DME (19, 21, 72). However, the results for diffuse DME have been less promising (18); perhaps this is because photocoagulation does not address the underlying cause of the edema. Increasing evidence suggests that the microvascular changes in DR and DME are at least in part secondary to inflammation (73). Leukocytes migrate and adhere to retinal vasculature in experimental models of diabetes (74, 75), which has been shown to cause premature endothelial cell death, capillary ischemia, and breakdown of the blood-retinal barrier (76–78).
Intravitreal corticosteroids bypass the blood-retinal barrier and can achieve intraocular therapeutic levels while minimizing systemic toxicities. Dexamethasone was initially investigated, but was limited by its short half-life in the vitreous (79). Attention shifted to triamcinolone acetonide, which is less potent but has a longer half-life due to its minimal solubility in water (80). For example, a 4 mg dose remains measurable in the eye for approximately three months (81), and a 25 mg dose for nine months (82). It is also well tolerated (83, 84). Other delivery methods, such as peribulbar triamcinolone, was recently shown to be ineffective in a Phase II RCT (85).
A number of small RCTs suggested the efficacy of intravitreal triamcinolone (IVTA) in treating advanced DME (86–90), but most studies lacked power and follow-up. The Diabetic Retinopathy Clinical Research Network (DRCR.net) was formed in 2002 as a collaborative network of sites in the United States (US) to facilitate multicenter trials.
The optimal dose of IVTA is unclear, but 4 mg is generally used in the US (80). The DRCR.net found no functional or anatomical outcome differences between 1 mg and 4 mg IVTA doses, while 4 mg had more side effects, suggesting that lower doses may be acceptable (91). Timing of reinjection is another uncertainty, because peak concentration and half-life may be heterogenous between patients (81). A study using optical coherence tomography (OCT) to monitor central macular thickness showed that recurrence of diffuse DME after one IVTA injection occurs around 24 weeks (90).
A recent Phase III DRCR.net trial of 840 eyes demonstrated that IVTA is not as effective as focal/grid photocoagulation, and is associated with cataract formation and ocular hypertension (91). At 4 months, the mean visual acuity was best in the 4 mg IVTA group (p ≤ 0.001), but by one year, there was no difference, and at two years, visual acuity in the laser group was better than the 1 mg (p ∇ 0.02) and 4 mg IVTA groups (p ∇ 0.002). OCT analysis of macular thickness also paralleled the visual acuity results. Outcome measures were comparable at three years (92), suggesting that photocoagulation should remain the gold standard to compare other therapies. A Phase III DRCR.net trial is now evaluating a combined photocoagulation-IVTA regimen, in comparison to laser alone, intravitreal ranibizumab plus laser, and intravitreal ranibizumab plus deferred laser (93).
The role of IVTA in PDR is less defined. Although large scale studies are lacking, IVTA appears at least in the short term to be well tolerated (94–97). Some surgeons also use intraoperative triamcinolone to improve visualization of the posterior hyaloid face. A Phase III DRCR.net study scheduled to enroll 381 subjects will be evaluating the adjunctive use of IVTA and ranibizumab following panretinal photocoagulation (PRP) for the treatment of PDR (98).
CORTICOSTEROID IMPLANTS
Intravitreal and retinal implant devices are being developed to allow sustained drug delivery to avoid the repeated injections of local steroids. Retisert (Bausche & Lomb, Rochester, New York) is a surgically implanted fluocinolone acetonide releasing intravitreal device that is US Food and Drug Administration (FDA) approved to treat chronic non-infectious posterior segment uveitis (99). A Phase II/III RCT with 197 subjects and 3 years of follow up found that 58% of implanted eyes compared to 30% of eyes treated with laser/observation had resolution of DME (p < 0.001) (100). However, of the implanted eyes, 95% of phakic eyes required cataract extraction, 35% developed ocular hypertension, and 5% necessitated implant removal to control intraocular pressure (100). Medidur is a nonsurgically implantable fluocinolone acetonide releasing device that is currently undergoing Phase II and III trials (101).
Ozurdex (Allergan, Irvine, California) is an injectable, biodegradable intravitreal dexamethasone drug delivery system (DDS). In June of 2009, the US FDA approved its use for treatment of macular edema secondary to retinal vein occlusions (RVO) (102). Data from two parallel Phase III studies demonstrated that the time to achieve ≥ 15 letters improvement in best corrected visual acuity was faster in eyes treated with 700 ug of Ozurdex (p < 0.01) (103). During the first 6 months of follow up, intraocular pressure elevation and cataract formation occurred in 25% and 4% of treated eyes, respectively. The preapproval Phase II RCT showed comparable effects between eyes with RVO and DME, suggesting that Ozurdex may be an effective and relatively safe DME treatment (104).
ANTI-VEGF THERAPIES
Basic Science of VEGF
VEGF is a key regulator of angiogenesis and has been implicated in the pathogenesis of neovascular diseases including DR, neovascular age-related macular degeneration (AMD), retinopathy of prematurity, and retinal vein occlusion. In diabetes, VEGF gene expression is upregulated in response to several factors including hypoxia, growth factors, PKC, AGEs, and reactive oxygen species (52, 105, 106). VEGF levels have been found to correlate with disease severity (107–109). It is produced by retinal pigment epithelial cells, glial cells, capillary pericytes, endothelial cells, Müller cells, and ganglion cells (110).
The VEGF family includes VEGF-A, -B, -C, -D, -E and placenta growth factor (PIGF)-1 and -2. VEGF-A (hereinafter referred to as VEGF) has been most frequently implicated in pathologic neovascularization. Through alternative splicing of VEGF’s eight exons, isoforms with 121, 145, 165, 183, 189, and 206 amino acids are created (111). VEGF165 is thought to be one of the key pathologic isoforms (112, 113).
The VEGF family exerts their functions via membrane-bound tyrosine kinase receptors VEGFR-1, -2, and -3. VEGFR-2 appears to be the major mediator of VEGF’s angiogenic properties. Soluble VEGFR-1 (sFlt-1) has also been identified as a decoy receptor that sequesters VEGF and has been shown to play a role in preventing corneal vascularization (114, 115). Its levels have been found to be lower in eyes with PDR (116). Gene delivery of sFlt-1 for the treatment of DR and DME is currently under investigation (117, 118).
VEGF is an attractive target for potential therapies not only because of its role in neovascularization as seen in PDR, but due to its proinflammatory and capillary permeability properties (119–121) that have been linked to the development and progression of DME. In fact, most anti-VEGF clinical research has focused on DME treatment.
Pegaptanib (Macugen)
Pegaptanib (Macugen, Eyetech, New York) is a pegylated neutralizing RNA aptamer, which selectively binds VEGF165. Data from a Phase II RCT of 172 patients with DME randomized to repeated intravitreal pegaptanib or sham injections showed that at 36 weeks, eyes treated with pegaptanib had better visual acuity (p ∇ 0.03); mean improvement was ≶4.7 letters with 34% improving 10 letters or more (122). Pegaptanib also reduced central retinal thickness (p ∇ 0.02), and further photocoagulation (p ∇ 0.04). Retrospective subgroup analysis of eyes with PDR at baseline showed that pegaptanib promoted regression of neovascularization and/or fluorescein leakage from neovascularization in eight of 13 (62%) eyes, compared to 0 of seven fellow, nonstudy eyes or those that received sham injections (123).
Ranibizumab (Lucentis)
Ranibizumab (Lucentis, Genentech, South San Francisco, California) and bevacizumab (Avastin, Genentech, South San Francisco, California) are both molecules that inhibit all VEGF-A isoforms. Bevacizumab is a monoclonal antibody against VEGF-A, while ranibizumab is the Fab fragment of the same antibody that has been affinity matured to provide greater affinity to VEGF. Ranibizumab was developed to treat neovascular AMD (124–126), but increasing evidence suggests that it is also useful for DR and DME (127, 128). The DRCR.net (93), RESOLVE (129), READ-2 (130), and the RISE and RIDE (131) studies are several upcoming Phase II and III RCTs that are evaluating the effects of ranibizumab in DME.
Bevacizumab (Avastin)
Bevacizumab is ranibizumab’s parent molecule. It is US FDA approved for treatment of metastatic colon cancer but is being used off-label as an anti-VEGF agent for ocular diseases such as neovascular AMD and DME (Fig. 20-1). It is unknown if bevacizumab and ranibizumab have similar efficacy and safety, but some practitioners prefer bevacizumab due to its lower cost. An upcoming National Eye Institute study will be comparing the two drugs head-to-head in the treatment for AMD (132).
In Haritoglou et al’s prospective case series of 51 eyes with diffuse DME, 1.25 mg of intravitreal bevacizumab reduced macular thickness at 12 weeks (p ∇ 0.001). Visual acuity improvement was noted at 6 weeks (p ∇ 0.02), but the improvement was not sustained at 12 weeks (133). Their follow-up study of continuous injections for chronic diffuse DME resulted in improved anatomic (p < 0.001) and visual (p ∇ 0.025) outcomes at 12 months (134).
The Pan-American Collaborative Retina Study Group (PACORES) reported a retrospective study of 78 eyes with DME and treated with at least one injection of 1.25 mg or 2.5 mg of intravitreal bevacizumab. Improvements in best-corrected visual acuity (BCVA) (p < 0.0001) and central macular thickness (p < 0.0001) were noted at 6 months (135), and the benefits continued at 12 months (136).
The DRCR.net conducted a Phase II multi-center trial of 121 eyes with DME randomized to focal photocoagulation, intravitreal bevacizumab, or both (137). At 12 weeks, eyes treated with intravitreal injections of 1.25 mg or 2.5 mg of bevacizumab had better visual acuity outcomes compared to laser treatment (p ∇ 0.01, 0.003, respectively). Central subfield thickness also decreased, but the benefit disappeared after 3 weeks. Combined bevacizumab-focal photocoagulation resulted in no apparent benefit. However, larger trials are necessary to make clinically meaningful conclusions on safety and effectiveness.
In regard to intravitreal bevacizumab for the treatment of PDR (138–145) and NVI (146, 147), the efficacy of bevacizumab alone or in conjunction with PRP and/or vitrectomy have also been demonstrated by small, mostly retrospective studies.
Figure 20-1. A: StratusTM optical coherence tomography (OCT) of a diabetic patient’s right eye, consistent with marked diabetic macular edema (DME) with large cystoid spaces. B: Repeat OCT of the same eye 4 weeks after injection of intravitreal bevacizumab shows decrease of DME with return of the foveal contour. The patient’s visual acuity improved by 3 lines on the Early Treatment Diabetic Retinopathy Study visual acuity chart. C: Color fundus photograph of the same eye demonstrating several hard exudates and macular edema. D: Color fundus photograph following bevacizumab injection, demonstrating less exudates and edema. E, G, I: Early, mid, and late-frame fluorescein angiographs (FAs) of the same eye showing significant leakage from microaneurysms. F, H, J: Early, mid, and late-frame FAs following bevacizumab injection. Although the light exposure is different on this visit, a decreased amount of leakage can be appreciated.
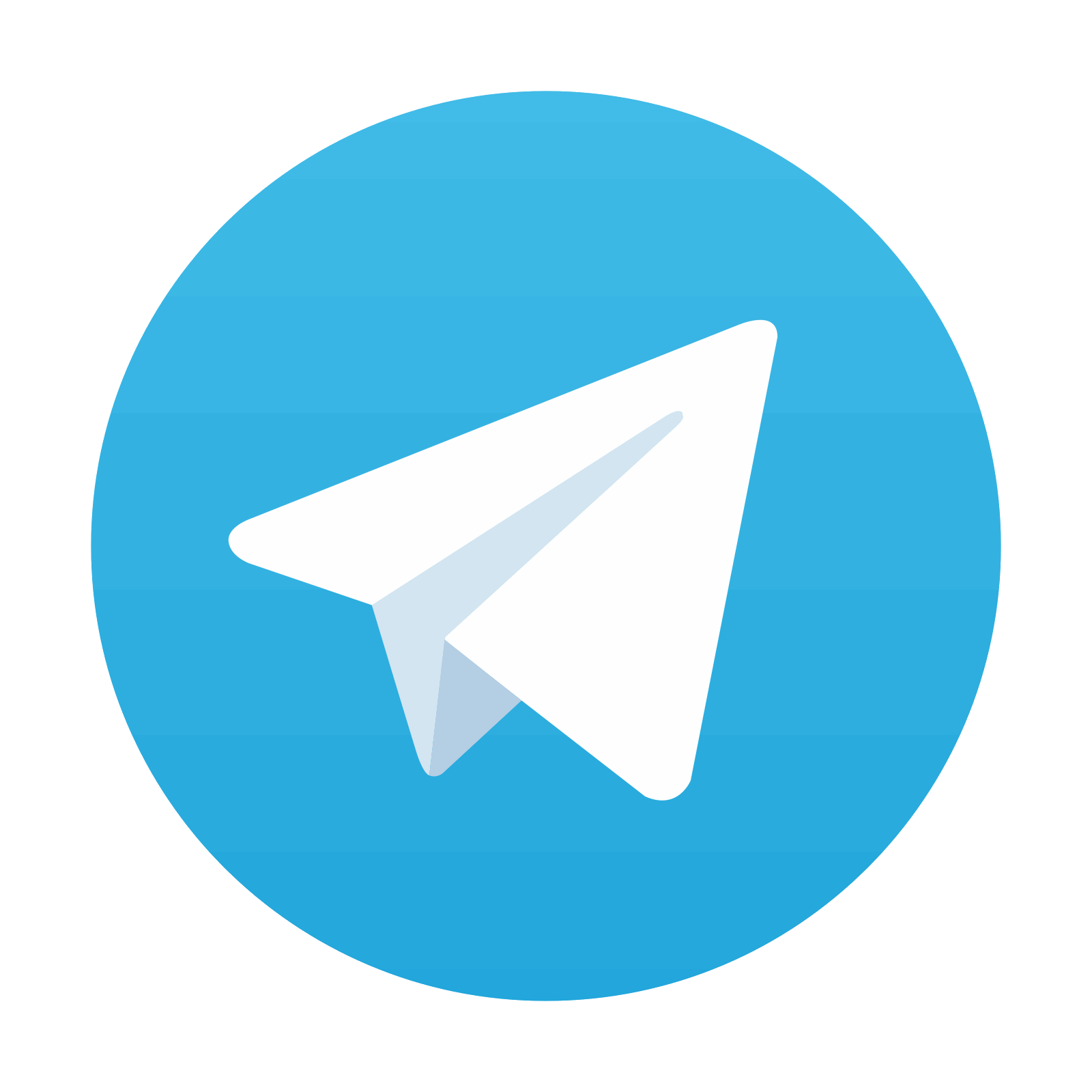
Stay updated, free articles. Join our Telegram channel

Full access? Get Clinical Tree
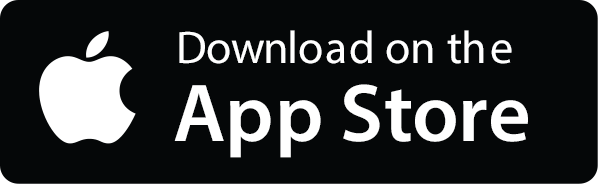
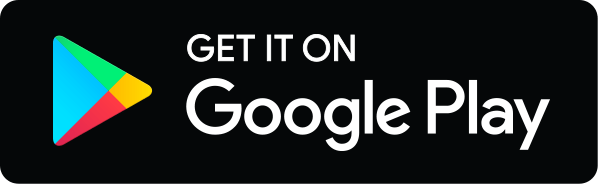