Pharmacology of Ocular β-Adrenoreceptor Antagonists
Paul S. Lee MD
Daniel A. Chruscicki MD
Prior to the introduction of prostaglandin analogues in the treatment of glaucoma, the most commonly used topical medications for reducing intraocular pressure (IOP) were topical β-adrenoreceptor antagonists. Often referred to as topical β-blockers, this group of medication is a highly efficacious class of compounds in lowering IOP, second only to prostaglandins. They can be prescribed once or twice daily and are relatively well tolerated.
β-Adrenoreceptor Pharmacology
β-adrenergic antagonists competitively (reversibly) inhibit the binding of catecholamines at the β-adrenoreceptor. β-adrenergic receptors are found in many different tissues (vascular, cardiac, pulmonary, and ocular). The receptors in the heart and blood vessels are primarily β1, whereas those in the lung are primarily β2.1 Agents that block both β1- and β2-receptors are classified as nonselective, whereas agents that primarily block β1-receptors are classified as cardioselective or simply as selective β1. Blockade of β1-receptors in the heart can induce or exacerbate heart block, bradycardia, and cardiac failure. Blockade of β2-receptors can precipitate bronchospasm, dyspnea, and respiratory failure, especially in patients with asthma or chronic obstructive pulmonary disease.
Although topical β-blockers have been used clinically for many years, their precise mechanism for lowering IOP is still not well understood. Several different theories have been proposed in attempting to explain the pharmacology and mechanism of topical β-blockers. The basic mechanism of action is accepted to be the same for all ocular β-blockers. The sites for receptor interaction of these drugs are suggested to be β-adrenergic receptors in the iris and ciliary body. Wax and Molinoff studied the distribution and properties of β-adrenergic receptors in the iris-ciliary body of humans.2 β-adrenergic receptors from the iris-ciliary body of human eyes removed shortly after death were studied using membranes prepared by isopycnic centrifugation of tissue homogenates. The affinities of the receptors for a series of agonists and antagonists were determined. The order of potency for the inhibition of the binding of the radioligand by antagonists was ICI 118,551 > MK950 > propranolol > ICI 89,406 > metoprolol. This order of potency is characteristic of β-adrenergic receptors of the β2 subtype. The highest density of receptors was located in the ciliary processes (180 fmol/mg of protein), whereas the density of receptors in the iris (98 fmol/mg of protein) and ciliary body (42 fmol/mg of protein) was notably lower. β1-adrenergic receptors comprise about 10% of the total number of β-adrenergic receptors in the whole iris-ciliary body. Most of the β-adrenergic receptors in the human iris-ciliary body are of the β2 subtype. Wax and Molinoff also confirmed the presence of β2-adrenergic receptors in cultured human trabecular cells and in human trabecular meshwork.3
The most commonly accepted theory of the mechanism of IOP reduction by topical β-adrenergic antagonists is that by blocking the β-receptors in the ciliary epithelium, aqueous production is suppressed, thereby lowering IOP. The biologic principles of ocular β-adrenergic agents were reviewed by Mishima.4 Mishima suggested that although β-adrenergic agents, such as epinephrine, decrease IOP by decreasing outflow resistance, β-adrenergic antagonists lowered IOP by reducing aqueous humor formation.
Neufeld attempted to explain the mechanism of action of timolol at the cellular level.5 In Neufeld’s studies, topically applied timolol did not increase cyclic adenosine monophosphate (cAMP) levels in the aqueous humor of rabbit eyes or stimulate cyclic AMP formation by ocular tissues, indicating that the drug has no intrinsic β-adrenergic agonistic activity (adrenergic agonists in many tissues exert their effects by activation of adenylyl cyclase and the cAMP pathway).6 Neufeld postulated that timolol may cause vasoconstriction of blood flow to the ciliary body, thus decreasing aqueous humor production, but was not able confirm this theory experimentally. Several decades later, Van Buskirk experimentally confirmed that topical β-blockers, namely timolol maleate and betaxolol hydrochloride, cause substantial, localized constriction in the arterioles that supply the ciliary processes.7 However, Millar and Wilson found no change in vascular resistance in arterially perfused bovine eyes after administration of timolol.8
Crook and Riese studied the effects of adrenergic agonists and antagonists on Na+, K+, Cl- cotransport in fetal human nonpigmented ciliary epithelial cells.6 Timolol, propranolol, and betaxolol inhibited the cotransport of the ions in ciliary epithelial cells, suggesting that aqueous humor production decreases due to the inhibition of Na+/K+/Cl- cotransport. Inhibition of Na+/K+/Cl- cotransport also inhibits cAMP formation, indicating that β-adrenergic antagonists may inhibit cAMP formation in ciliary epithelial cells.
Although Maren was able to confirm that carbonic anhydrase inhibitors decreased the flow of sodium from plasma to aqueous, timolol was shown to have no effect on movement of sodium from plasma to aqueous. Maren did confirm that aqueous humor production was reduced with timolol, but did not find similar reduction of sodium transport from plasma to aqueous humor as seen with carbonic anhydrase inhibitors.9
More recently, Kiland et al. studied the mechanisms of timolol on aqueous suppression and ultrafiltration and confirmed that timolol does decrease the formation of aqueous humor, but does not enhance filtration or increase the outflow facility of aqueous humor through the trabecular meshwork.10 Although aqueous humor production was reduced with timolol treatment, outflow facility through the trabecular meshwork was also reduced, even compared to untreated eyes, indicating that over time, reduction of aqueous humor production may lead to reduction in outflow facility. Kiland measured aqueous humor ascorbate and fibronectin levels in monkey eyes to better elucidate the mechanisms of timolol on aqueous humor production. Timolol was shown to have no effect on ciliary epithelial transport of ascorbate or aqueous fibronectin levels. If timolol affects the Na+/glucose transporter, as has been theorized previously, then ascorbate levels would also be expected to be decreased due to ascorbate’s link to the Na+/glucose transporter. Kiland’s findings that timolol does not affect levels of ascorbate in the aqueous humor indicate that timolol may not inhibit the Na+/glucose transporter.
Topically applied timolol is not metabolized in the eye, at least in rabbits and monkeys,11 but undergoes extensive hepatic elimination after it reaches the systemic circulation.12 The time of onset of timolol is short. After topical application in rabbits, the drug appears rapidly in the aqueous humor.13 Phan determined plasma and aqueous humor levels of four ophthalmic β-blockers: timolol, betaxolol, levobunolol, and carteolol in rabbit eyes.14 Levels of β-antagonist were measured in the plasma and aqueous humor 1 hour and 12 hours after instillation of 50 μL of timolol, betaxolol, levobunolol, or carteolol. Plasma levels and aqueous levels were elevated 1 hour after instillation of β-blocker drops and continued to be elevated up to 12 hours after instillation. Peak aqueous humor concentrations of all four β-blockers were extremely elevated at 1 hour after administration (timolol 1613.58 ng/mL; betaxolol 866.06 ng/mL; levobunolol 750.89 ng/mL; and carteolol 859.18 ng/mL). At 1 hour after administration, the plasma concentration of timolol (9.89 ng/mL), levobunolol (1.60 ng/mL), and carteolol (8.00 ng/mL) were markedly elevated and at levels 11.82 to 29.22 times their respective Ki values, suggesting virtual blockage of both β1- and β2-receptors systemically and also suggesting significant systemic absorption. Betaxolol has a peak 1-hour plasma level of 22.28 ng/mL, which is equivalent to only 3.08 times its Ki (binding affinity) for the β1-receptor and 0.36 times its Ki for the β2-receptor, indicating less systemic β1 blocking activity compared to the other three medications and minimal systemic β2 blocking activity. At 12 hours after administration, plasma levels of all four β-blockers remained moderately elevated (timolol 0.94 ng/mL; betaxolol 9.43 ng/mL; levobunolol 0.66 ng/mL; and carteolol 1.61 ng/mL).
Ohtori used a microdialysis method to study the pharmacokinetics of topical β-blockers in rabbit aqueous humor.5 Ohtori confirmed Phan’s earlier findings that ophthalmic β-blockers achieve high levels in the aqueous humor shortly after instillation. Within 60 minutes, timolol and carteolol levels reached maximal levels in the aqueous humor after topical instillation to the eye.
Recently, more work has been done to determine the levels of β-blockers circulating in the system after topical administration. Oral β-blockers are known to undergo a significant first pass effect, thereby reducing the amount of drugs that will be available to reach end organs.16 In humans, oral timolol undergoes moderate first-pass metabolism resulting in 50% bioavailability after an oral dose with a half-life of 3 to 5 hours.17 Topical ophthalmic administration of timolol, however, bypasses first-pass metabolism.
Kaila was able to determine the concentration of timolol in plasma with a sensitive radioreceptor assay.18 The method was later modified for betaxolol. With this method, topically applied ocular timolol was shown to circulate systemically for at least 12 hours after administration.19 Plasma kinetics and antagonist activity of timolol were studied in 12 patients scheduled for extracapsular cataract extraction and intraocular lens implantation. The patients received 40 μL of 0.25% timolol into the lower cul-de-sacs of each eye prior to surgery. Blood samples were collected over a period of 12 hours and plasma concentrations of timolol were analyzed using the radioreceptor assay. Timolol was rapidly absorbed into the systemic circulation and occupied, on average, close to 68% of β1-receptors and 87% of β2-receptors. Twelve hours after the single dose, β1- and β2-receptor occupancy decreased slowly and was, on average, 38% and 64%, respectively. The calculated mean area under the concentration-time curve of timolol in plasma was 10.26 ng/mL/hr and the mean half-life 4.6 hours. Both values were much higher than those found in healthy young volunteers following an intravenous 0.25 mg dose of timolol.20
Vuori studied plasma and aqueous humor concentrations of topical betaxolol and timolol in humans and noted rapid systemic absorption of both medications with noticeable systemic side effects even with trace amounts of β-blockers in plasma.21 Forty-five patients scheduled for extracapsular cataract extraction and intraocular lens implantation were randomly divided into three groups and received 40 μL of 0.5% betaxolol, 0.25% timolol, or placebo into the lower cul-de-sacs of both eyes immediately prior to undergoing cataract surgery. Blood samples were collected immediately before and 5, 10, 15, 30, 60, 120, 180, and 240 minutes after instillation of the drug. At the beginning of the operation, aqueous humor samples were aspirated. Plasma and aqueous humor concentrations of timolol and betaxolol were analyzed using a sensitive radioreceptor assay. Timolol and betaxolol were both absorbed rapidly from the eye into the systemic circulation. The highest average plasma timolol concentrations (mean 1.05 ng/mL, SD 0.58 ng/mL) were found as soon as 15 minutes following drug administration. Timolol plasma levels decreased slowly, so that the mean concentration was 0.82 ng/mL SD 0.11 after 240 minutes. The mean peak concentration (Cmax) was 1.36 ± 0.55 ng/mL, and the mean time to peak value was 80 ± 76 minutes. The plasma concentrations of betaxolol were lower than those of timolol. The highest average betaxolol concentration was 0.7 ± 0.8 ng/mL, which was detected 120 minutes after drug instillation. The mean peak concentration of betaxolol was 1.1 ± 0.8 ng/mL, and the mean time to peak value was 89 ± 79 minutes. The mean betaxolol concentration 4 hours after administration of 40 μL of 0.5% betaxolol hydrochloride to both eyes was 0.5 ng/mL. The mean concentration of timolol in the aqueous humor was 1.65 ± 0.78, 59 ± 19 minutes after drug application, and the mean betaxolol concentration was 4.1 ± 1.4 μg/mL, 63 ± 12 minutes after drug instillation.
Binchetti et al. reported that the elimination half-life of betaxolol is lengthened in the elderly.22 Vaino-Jylha studied the plasma concentration of betaxolol in elderly patients during the normal 12-hour dosing interval of the drug and found surprisingly high concentrations of betaxolol in plasma after topical application in elderly patients up to 12 hours after dosing.23 Plasma concentrations of betaxolol were measured 12 hours after instilling 20 μL 0.5% betaxolol hydrochloride in both eyes of nine glaucoma patients. The mean concentration of betaxolol was measured to be 0.4 ng/mL with a variation from 0.2 to 0.8 ng/mL, indicating that betaxolol remains circulating in the plasma for much longer than 4 hours. Additionally, individual variation in the systemic absorption of betaxolol was large.
In conclusion, several studies have confirmed that ocular β-blockers achieve high concentrations in the aqueous humor rapidly and that ocular β-blockers are also absorbed into the system rapidly. After rapid absorption, binding of β-adrenergic receptors may continue up to 12 hours after the application of topical ocular dose. Higher amounts of the drug were measured in plasma of elderly patients compared to younger patients, which helps explain why elderly patients are more susceptible to the systemic side effects of topical β-blockers.
As of 2006, six β-adrenergic antagonist agents are available for ophthalmic use: timolol maleate, timolol hemihydrate, levobunolol, metipranolol, carteolol, and betaxolol. Several different formulations of timolol maleate gel and solution exist, which contain varying amounts of preservative (Table 1). Sorenson, Brooks, and Zimmerman have conducted reviews of ocular β-blockers and summarized the pharmacologic properties of ocular β-blockers according to their β-blocking potency, partial agonist activity, β1 selectivity, local anesthetic effect, and serum half-life (Table 2).24,25,26
TABLE 1. Nonselective and Selective β-Adrenergic Antagonists | |||||||||||||||||||||||||||||||||||||||||||||
---|---|---|---|---|---|---|---|---|---|---|---|---|---|---|---|---|---|---|---|---|---|---|---|---|---|---|---|---|---|---|---|---|---|---|---|---|---|---|---|---|---|---|---|---|---|
|
|
The efficacy in lowering IOP of the various formulations of topical β-adrenergic antagonists is comparable when administered once or twice daily. Several large clinical studies have confirmed that β-blockers have similar levels of efficacy in reducing IOP (Table 3). In contrast to the prostaglandin analogues that have been shown to have an IOP lowering effect during the nocturnal period, β-blockers are less effective in reducing IOP during the nocturnal period. Topper and Brubaker demonstrated that the rate of aqueous flow at night is only half of the daytime value, and topical timolol treatment has no measurable effect on nighttime aqueous humor flow.27 It has been suggested 28 that this is at least partly caused by the levels of circulating catecholamines, which also display a circadian rhythm.29
TABLE 3. Clinical trials of topical β-adrenergic antagonists | |||||||||||||||||||||||||||||||||||||||||||||
---|---|---|---|---|---|---|---|---|---|---|---|---|---|---|---|---|---|---|---|---|---|---|---|---|---|---|---|---|---|---|---|---|---|---|---|---|---|---|---|---|---|---|---|---|---|
|
Subsequent studies have confirmed that β-blockers do not reduce IOP in the nocturnal period as effectively as prostaglandins. Liu et al. measured the diurnal and nocturnal IOP curves of 18 patients with ocular hypertension or early primary open-angle glaucoma who were treated with timolol in the morning or latanoprost in the evening. Although the diurnal IOP curves were similar for timolol and latanoprost, the nocturnal curve of timolol was not different from patients who were on no medication and was higher than patients who were treated with latanoprost.30 Orzalesi et al. also compared the IOP lowering effects at nighttime of timolol, latanoprost, and dorzolamide and determined that timolol was less effective in reducing IOP during the nighttime compared to latanoprost and dorzolamide.31 Finally, Ong et al. compared dosing of timolol GFS as monotherapy in the morning compared to dosing as monotherapy in the evening and found that dosing timolol GFS in the morning was more effective in lowering IOP compared to evening dosing.32
Recently, many glaucomatologists have supported the recommendation for dosing of once-daily β-blockers in the morning. Patients should be instructed to administer once-daily β-blockers every 24 hours because the maximum efficacy may wane in some patients after 24 hours.33 Thus, in patients taking nonselective β-blockers once daily, they should be reminded to instill the drops at the same time each morning.
After the introduction of topical β-adrenergic antagonists for the management of glaucoma, several studies have confirmed that β-blockers can reduce IOP in the contralateral eye despite not having received a dose of medication. Dunham confirmed reduction of IOP in the contralateral eye of patients given topical timolol and timolol lingually, which suggests that the contralateral reduction in IOP from timolol is caused by systemic absorption.34 In conjunction with the Ocular Hypertension Treatment Study, which enrolled over 1,500 patients and randomized more than 800 subjects to receive topical medication and more than 800 subjects to be monitored closely, a mean reduction in IOP in the β-blocker-treated eye was around 6 mm Hg, whereas the contralateral eye had a mean IOP reduction of 1.5. Of the contralateral eyes, 35% showed a reduction of 3 mm Hg or more and 10% showed a reduction of 6 mm Hg or more. The contralateral effect of the relatively selective β-blocker betaxolol did not differ from that of any of the nonselective β-blockers.35
In order to decrease systemic absorption of ocular medications and increase ocular bioavailability, many physicians recommend occlusion of the nasolacrimal duct to block passage of topical medication into the duct. Zimmerman studied the effect of nasolacrimal occlusion on the therapeutic index of various glaucoma medications.36 With timolol, nasolacrimal occlusion collapsed the dose-response curve and extended the duration of action. The effect of nasolacrimal occlusion on systemic side effects was not evaluated in this particular study. Kaila examined systemic absorption of timolol after nasolacrimal occlusion in eight healthy volunteers.37 Although total timolol absorption was decreased with nasolacrimal occlusion, great individual variation occurred in timolol absorption. Several studies have confirmed improved efficacy (through lower IOP) of topical glaucoma medications after nasolacrimal occlusion.38,39
Due to the prevalence of cardiovascular disease, many glaucoma patients are on concurrent systemic β-adrenergic antagonist therapy. A few studies have evaluated the effect of oral β-blockers on the effectiveness and side effect profile of topical β-blockers. Theoretically, concurrent use of systemic and topical ocular β-blockers could cause an additive effect on the cardiovascular system. An initial study indicated minimal interaction between oral and topical timolol.40 In a double-masked trial of 31 patients with open-angle glaucoma, the use of topical timolol 0.5% twice daily for 1 week did not enhance the pulse and blood pressure-lowering effects of timolol 20 mg orally twice a day. In another study, seven healthy patients receiving 80 to 160 mg of propranolol orally daily were treated once with timolol 0.5% in a single eye and showed no significant effect on pulse rate or blood pressure.41 In a more recent study, Schuman collected data from two prospective, randomized, actively controlled 12-month clinical trials comparing the impact of systemic β-blockers on the efficacy and safety of topical brimonidine and topical timolol.42 Of 66 patients on concurrent systemic β-blocker therapy, 34 had been assigned to the brimonidine group and 32 to the timolol group. Timolol-treated subjects concurrently taking systemic β-blockers had smaller decreases in IOP, a greater mean change in systolic and diastolic blood pressure and a significantly greater mean decrease in heart rate compared with timolol subjects not taking β-blockers. A few possible explanations for this finding may be that preexisting ciliary body β-adrenergic receptor blockade occurs with chronic systemic β-blocker therapy or that desensitization of the β-adrenergic receptor occurs with chronic systemic β-blocker therapy.42
Gerber et al. conducted an extensive review of systemic drug interactions with topical glaucoma medications, compiling evidence from the literature including individual case reports and also using the Physician’s Desk Reference (PDR) and the United States Pharmacopeia Drug Information (USPDI) directory.43 Despite the numerous warnings listed of potential interactions between topical glaucoma medications and systemically administered drugs in the PDR and USPDI, limited literature supports and clinically confirms these interactions and many of the interactions are anecdotal. In Gerber’s review, potential interactions between systemically administered drugs, such as quinidine, xanthines, and β-adrenergic agonists and topical β-adrenergic antagonists, were well supported. There are many reports of patients on bronchodilators and xanthines with chronic obstructive pulmonary disease experiencing respiratory distress due to ophthalmic β-blocker administration.44,45,46,47
Timolol, in blocking β-adrenergic cardiac receptors, has been shown to exacerbate the cardiodepressant activity associated with quinidine.48 Edeki et al. further studied excessive β-blockade in patients receiving topical timolol who were also taking quinidine.49 Edeki postulated that because quinidine inhibits the cytochrome P-450 enzyme CYP2D6, which also metabolizes timolol maleate, patients taking both medications would have higher plasma levels of timolol and more systemic side effects. In the study, plasma concentrations of timolol and exercise-induced heart rate were measured in two groups of patients: extensive metabolizers of the enzyme CYP2D6 and poor metabolizers of the enzyme CYPD26. Both groups of patients received ophthalmic timolol maleate 0.5% directly to the nasal mucosa. Plasma concentrations of timolol and exercise-induced heart rate were measured in both groups. At a subsequent session, the extensive metabolizers received either oral quinidine or placebo 30 minutes prior to topical administration of timolol. The results indicated that the exercise-induced heart rate in both groups was depressed compared with the placebo, and the plasma level of timolol was greater. Furthermore, the impact of this combination on exercise-induced heart rate was greater among the poor metabolizers compared with the extensive metabolizers. Higginbotham emphasized the significance of this study, noting that 8% of the white population, 1% of the Asian population, and 2% to 4% of the African American population are thought to be poor metabolizer phenotypes.50
Timolol maleate
Timolol maleate, a nonselective β-adrenergic antagonist, has been available in the United States since 1978. Timolol is also useful in many cases of secondary glaucoma, aphakic glaucoma, and ocular hypertension.51,52,53,54 The attraction of timolol lies in its long-term efficacy in the treatment of various types of glaucoma, the low incidence of ocular side-effects, and relatively long duration of action (12 to 24 hours), which allows for once-daily or twice-daily application.
Topical ocular administration of 0.25% and 0.5% timolol maleate results in lowering of IOP in normal and glaucomatous eyes.55,56,57,58,59 Topical timolol is effective in reducing IOP 20% to 30% compared with pretreatment values.57,60 The maximum hypotensive effect of timolol is reached with the dose range of 0.3% to 0.5%.56 The onset of action of timolol can usually be detected within one half hour after a single dose. The maximum effect usually occurs in one to two hours.21 Timolol maleate is the prototype medication for β-adrenoreceptor antagonists; thus, the other medications in this class can be expected to have a similar time of onset and time to maximal effect as timolol maleate (Fig. 1).
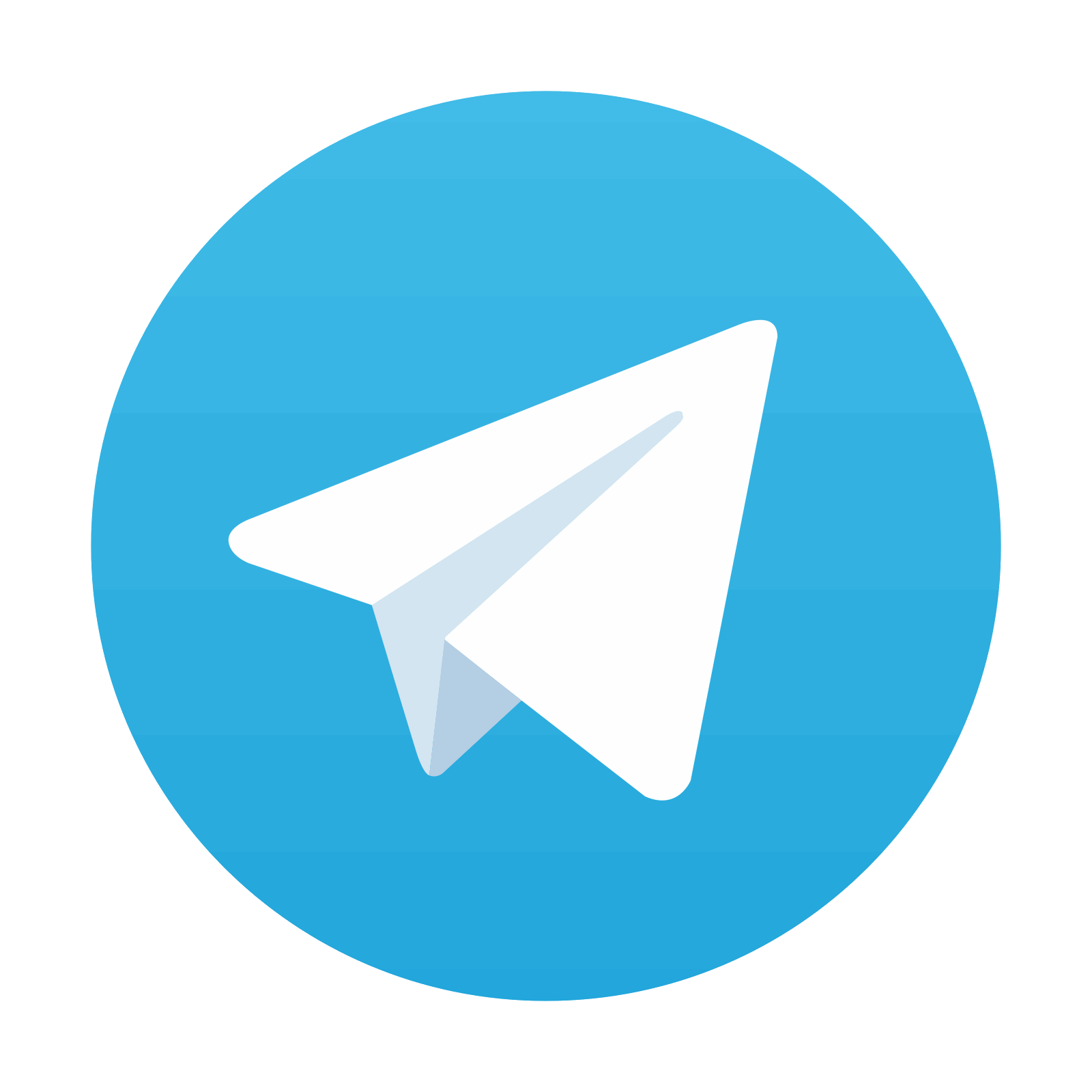
Stay updated, free articles. Join our Telegram channel

Full access? Get Clinical Tree
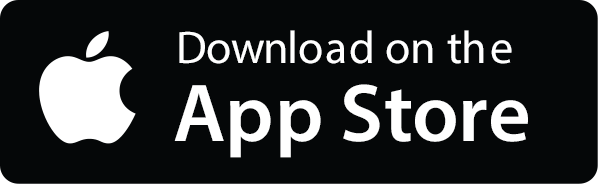
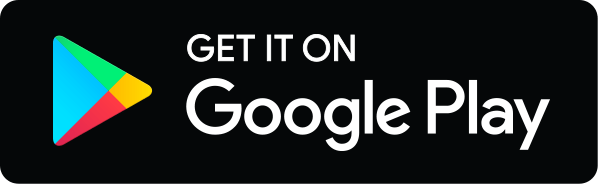