5 Pearls in Femtosecond Laser-Assisted In Situ Keratomileusis LASIK Surgery
Summary
FS lasers gave their debut as tools in anterior segment surgery in LASIK surgery, revolutionizing the safety of the procedure. The chapter gives an introduction in the engineering concepts which define the tool: Ultrashort infrared laser pulses generate optical breakdown in the irradiated tissue. Computerized scanning patterns are needed to create cleavage planes in the cornea to separate cellular structures without cutting. Keeping these principles in mind, the surgeon may make best use of the technology in the LASIK procedure. The flap is defined by the FS laser pattern yet created by the surgeon in the process of lifting. The disruption of residual tissue bridges is a central part of the surgical manipulation. A critical step of the process is optimal docking of the eye to the FS laser device. The dispersion of the gas in the cleavage plane indicates the advancement of the laser effects, however may also impede subsequent surgical steps if an opaque bubble layer is formed. A paramount advantage of FS laser flap creation is the option to create angulated edges which greatly enhance postoperative flap stability, minimizing flap-associated complications. The entire surgical procedure of FS laser flap design, definition and surgical handling is profoundly different from microkeratome surgery. The chapter covers a number of central aspects related to the surgery.
Keywords: Photodisruption, Laser induced optical breakdown, Opaque bubble layers, Cleavage pattern, Tissue bridges, Flap architecture, Angulated flap edges, Flap stability
5.1 Introduction
When laser-assisted in situ keratomileusis (LASIK) was developed to be a routine clinical application in the 1990s, a major technical milestone for the procedure was the development of the automated microkeratome. 1 At that time, excimer lasers had made their way for a decade into ophthalmic surgery, enabling high predictability and high precision of tissue ablation. Also, excimer laser photorefractive keratectomy had become a procedure of a hitherto unknown degree of automatization due to computerized pattern control mechanisms. However, downsides of surface ablation such as stromal haze became obvious in patients with higher degree myopia.
In addition, the perspective of prolonged pain in the postoperative period deterred candidates from the procedure. 2, 3, 4, 5 Hence, performing the ablation within the corneal stroma was recognized as preferable with a better risk profile as to the postoperative situation. However, as ultraviolet light energy emitted by the excimer laser is absorbed at the surface of the treated tissue, this created at the same time the need for a superficial flap to obtain access to the corneal stroma. The mechanical microkeratome at the time was the key to this challenge. A tool optimized to slice a thin dish of tissue from the superficial layers of the cornea facilitated the introduction of this auxiliary step in refractive surgery. While the incidence of previously known side effects of surface ablation such as inflammatory haze receded, a whole plethora of novel risks appeared: free cap, buttonhole, striae were among the phenomena that made their appearance on the stage of refractive surgery at that time, contributing to potentially devastating complications in terms of the patients’ vision. 6 In fact, with the widespread adoption of LASIK technique, flap-related complications would dominate over ablation-related complications. Innovation in digital technology and growing insight into the biomechanics of corneal photoablation allowed rapid improvement of the result quality in laser vision correction procedures. Microkeratomes could not keep up to the pace of progress, as their basic concept is rooted in a mechanical blade temporarily locked upon the eyeball.
The advent of femtosecond laser (FS) technology around the millennium and its availability for ocular microsurgery marked a turning point to this challenge. Even more so, retrospectively it opened the door for a novel type of surgical procedures in ophthalmology. With options intrinsically reaching far beyond the mere job of corneal flap creation, it provided the ophthalmic surgeon’s fantasy a plethora of novel applications for this kind of virtual blades. The chance for extremely precise microsurgical action within a tissue without opening the integrity of the surface was quickly recognized as a former coveted utopia in surgery.
5.2 Understand the Working Principles of Your Tool
Under natural conditions, infrared (IR) light energy is absorbed by the cornea and transformed into thermal energy, that is, heat the tissue. However, the same wavelength, when delivered in ultrashort and high-energy pulses in the FS range, may be focused to cause a photodisruptive effect. 7 A material which is transparent for light has its electrons associated in a fixed orbital correlation to the atomic nucleus. When photons with high energy impact onto such material, this electrostatic bond of the electrons in the atomic compound is overcome, releasing the negatively charged electrons. This means that light energy at a naturally transmissible wavelength is absorbed if delivered as an ultrashort laser pulse. This phenomenon is called laser-induced optical breakdown (LIOB). 8 The cascade of released electrons with a negative charge displays mutual repulsion causing an explosive centrifugal movement (plasma). Thus, a cavitation bubble is formed. It expands as a shockwave initially at a supersonic speed to the point of internal versus external pressure equality. 9 This expansion that is immediately followed by a collapse causes the disruption of the material. By delivering not only a single FS pulse but also a fast sequence of pulses, this separative effect may be utilized to create a contiguous line of tissue separation.
This observation and the insights into the laws of such a process were at the origin of FSs as a surgical tool for precise tissue separation. The phenomenon is termed optoacoustic coupling to describe the translation of electromagnetic into acoustic (kinetic) energy. 10 Ultrashort pulses in the femtosecond range do not entail a spatial deflection of atoms, hence do not lead to a warming of the material. This is another major difference to lasers operating at the same wavelength with pulses in the millisecond or microsecond range which induce thermal coupling, that is, a rise of temperature at the focal point of absorption.
This complex combination of physical conditions renders an FS a delicate concept to be used as a tool for tissue separation. Indeed physics is only one part of the equation—the other, equally relevant one is physiology and cell biology. If it were about dissecting a homogeneous material with precisely defined properties, we would almost be there. However, the human cornea is a compound, multilayered tissue with varying elasticity across its profile. In the early days of prototypes of FS microkeratomes, one of the major challenges for routine applicability was the attunement of the determining factors: energy per pulse and spatial and timely distribution of the sequence of pulses forming the pattern of disruption points to achieve a smooth tissue cleavage. Unless this multifactorial equation could be iteratively optimized, we would see tissue samples with isolated points of disruption, however nothing like a continuous line of cleavage.
5.3 The Pattern of Cleavage—Tissue Bridges
As the previous paragraph has reminded us of the physics behind the FS tool, we may now turn our attention to the details of creating a corneal flap. It may be helpful to keep the process of delivering myriads of tiny explosion bubbles in mind when separating the anterior lamella from its stromal bed: even if ideally it may feel like a sliced piece of tissue, there are occasionally tissue bridges which require manual disruption (▶ Fig. 5.1). 11 You may encounter such points of resistance both in the side cut and in the plane of cleavage. The reason can be with the settings of the femtosecond device itself, with the cornea or with the interface between the machine and the patient.
Fig. 5.1 Tissue bridges between single effect bubbles from femtosecond laser action in cornea. Note the undisrupted continuity of collagen lamellae in the immediate vicinity of the void spaces where the collagen lamellae were set apart by the laser pulse. Experimental setting with first-generation femtosecond laser microkeratome, porcine cornea, HE (hematoxylin-eosin) staining ×40 magnification.
Unless the settings of the laser device are optimized concerning the spot-line spacing of the laser pulses and the energy of the pulses, tissue bridges can be a common challenge when opening the flap. 12 This cause can be recognized by a high degree of resistance across the entire plane of tissue cleavage, that is, if a lot of mechanical strain is required to form and lift the flap. Although an FS-delineated flap may not be handled like truly sliced, that is, with zero resistance to your instrument, if only by plucking with a spatula under the flap-to-be, it is the technician’s turn to modify the settings. Some machines are equipped with an expert mode that allows independent modification of the laser patterns, but still drawing on the service-engineer’s experience and expertise instead of blank iteration is a wise step. For some physical and mathematical reasons, FS sources, the inner heart of these devices, are not perfectly alike between them. Their energy emission characteristics vary from one to the other as well as over the lifetime of the laser source, and there are also influences from the climate and the air pressure in the operating room that contribute to such individual characteristics. In fact, comparing a laser device to a grand piano has some truth: though made on the same construction scheme from the same materials, no two pieces are perfectly equal.
If solid islands of tissue bridges remain after a seemingly correct laser procedure, check the video footage of the start of your procedure, particularly the moment of docking the patient’s eye to the interface. Tiny air bubbles trapped in the fluid film between the docking glass and the epithelium deflect the laser, shielding the underlying tissue from the disruptive effect as the laser pulse becomes defocused. This results in undisturbed tissue integrity at the location of the bubbles; that is, when opening the flap, these spots have to be ruptured. It is obvious that such a brute-force approach is not compatible with the intention of an FS tissue cleavage boasting a no-touch separation. To avoid this complication, it is advised to ensure a solid and homogeneous lubrication of the corneal epithelium before docking the glass. This applies to both applanating and curved patient interfaces as designed for the various machines.
Another reason for portions of virgin tissue in the intended plane of laser separation is sometimes on the patient’s side: If the docking is sufficient but not perfect, even gentle movements of the patient’s eye—or the head with the eye—may cause a minimal excursion of the cornea. This can be either in the horizontal or in the vertical axis. 13 If the patient interface is clutching a small portion of the conjunctiva, a minuscule degree of rotation of the eye is possible without losing critical suction which would lead to the interruption of the procedure. In an outside-in scanning system, the patient’s view of the fixation light is blurred when the separation of the flap-bed is completed. If this results in the patient trying to search for the fixation light, a minimal saccade may result in a minimal offset of the side cut against the bed. Reaching under the flap from the side cut with the spatula meets a solid resistance. How do you avoid this? The curved interface must not be too large, lest a lobe of conjunctiva is trapped, making the position potentially instable. Soaking the tear film from the conjunctiva with a Weck-Cel or Merocel sponge helps ensure a good grip of the docking piece. Also, ensuring sufficient topical anesthesia before the procedure avoids excessive tear production during the docking. And—last but not least—the patient should be informed ahead of the surgery about the steps of the procedure, how it will feel and look. Between the docking and the undocking, it has proved helpful for both the surgeon and the patient to reassure the patient about his or her perfect cooperation telling him or her that everything runs smoothly as scheduled.
Finally, though this is both obvious and rare, defects in the corneal integrity with even a minimum degree of opacity can be the cause of inconsistent tissue separation under the FS. 14 These may be scars from previous accidental subepithelial foreign bodies or corneal surgery—admittedly, such eyes will not go unnoticed, if at all into a FemtoLASIK procedure. However, if you apply an ink-stamp marking on the epithelium to have an additional reference for astigmatism correction, the blue ink may be sufficient to shield the laser pulses and leave the tissue underneath untreated. 15, 16
5.4 The Pattern of Cleavage—Unwanted Gas
We have discussed the mechanism of action of the FS in the first paragraph: you remember the gas? Let us transform the bubbles into the next “pearl.”
In a regular procedure, the gas bubbles form a layer in the cleavage plane and dissipate over the first few minutes after completion. However, in some instances, the gas tends to go astride and creates clouds in the stroma. The effect is a thorough opacification of the cornea in the affected area and may lead to incomplete tissue separation. Again, the dispositions of the spot-line spacing as well as the energy play a role in this issue. An overly dense pattern of laser pulses with formation of excess gas bubbles can lead to accumulation of gas. However, this will not lead to an opaque layer unless the gas finds a way to propagate between stromal layers. The histoarchitecture of the cornea that determines corneal hysteresis, elasticity, thickness, resistance, and curvature plays a determining role for the phenomenon of opaque bubble layer (OBL) formation. 17 The IntraLase FS device includes a “pocket” in the cleavage pattern to minimize this problem: at the position of the hinge, a deep line of tissue separation is created to serve as an exhaust valve. 8, 17 This was introduced in an early version of the machine resp. software, as the machine has a line scanning pattern starting at the “pocket.” Also, earlier machines had less subtle energy settings than current versions of the device. In the Wavelight FS 200 Laser, an exhaust vent to the rear side of the hinge is created with the same purpose (▶ Fig. 5.2).
Fig. 5.2 (a) The red arrows indicate the position of the small bubbles that subsequently will be shown to block the regular tissue cleavage from the femtosecond (FS) laser pulses. In the periphery of the microscope view is the front of the cleavage plane visible. (b) The FS laser pulse pattern has reached the position of the bubbles (red arrows). Void spaces are visible in the regular gas pattern. (c) At the end of the FS laser procedure, interruptions of the cleavage plane are visible at the spots of the initial bubbles (red arrows).
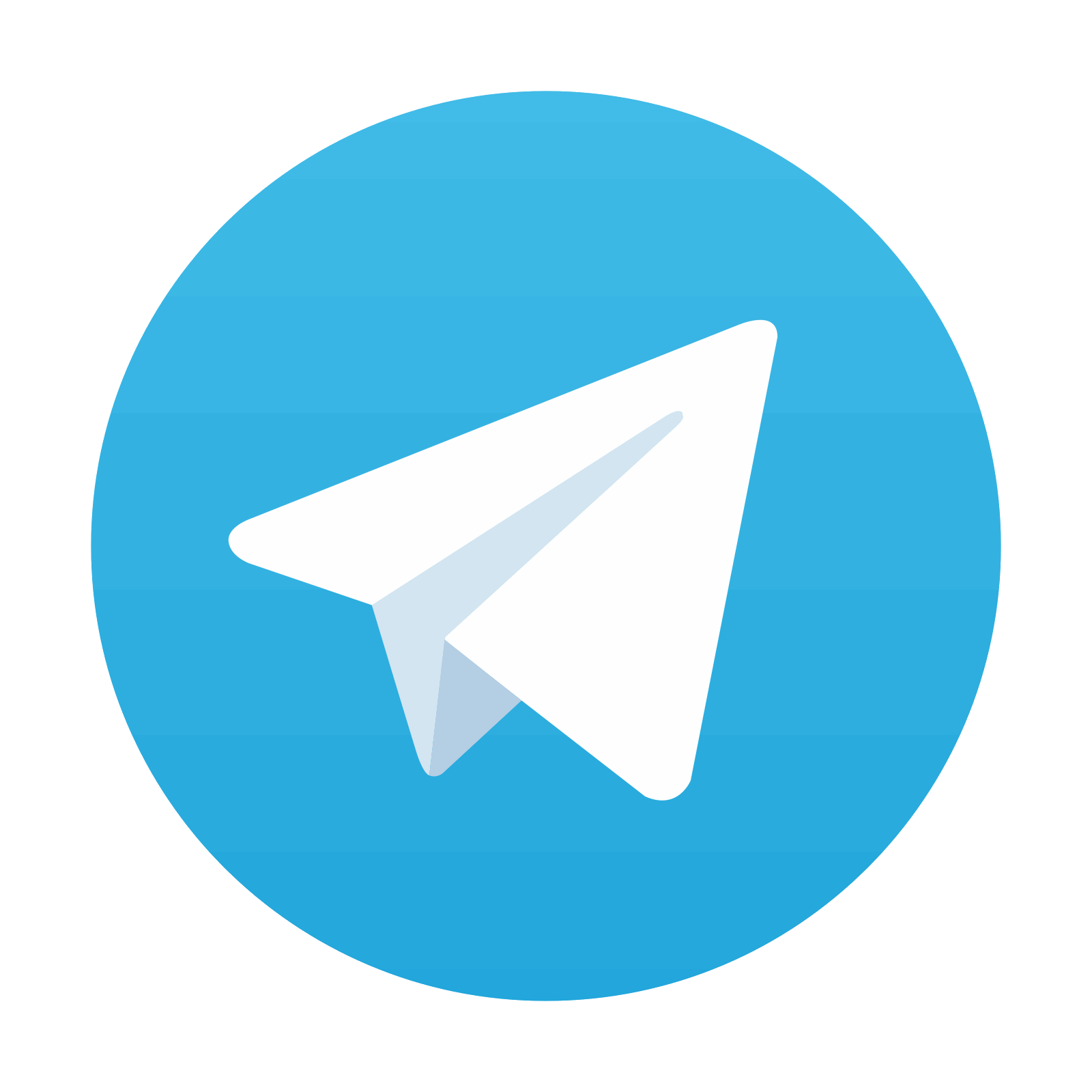
Stay updated, free articles. Join our Telegram channel

Full access? Get Clinical Tree
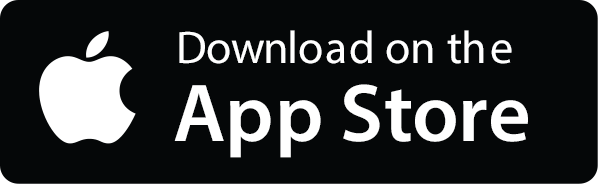
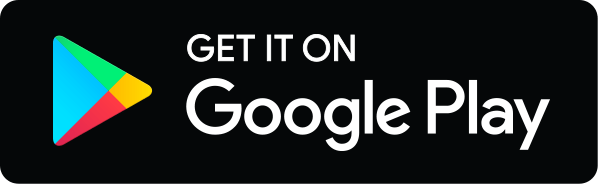