Pathophysiology of the Retinal Pigmented Epithelium and Choroid
James T. Handa
INTRODUCTION
The retinal pigmented epithelium (RPE)-Bruch’s membranechoroid complex is a vital structure that maintains the health of the neurosensory retina. The RPE interacts closely with the photoreceptors of the neurosensory retina, and has multiple functions including photoreceptor outer segment (POS) turnover, neutralization of photo-oxidative stress, heat dissipation, and vitamin A homeostasis. For example, the RPE processes the phagocytosis of 25,000 to 30,000 POS tips every day (1). The Bruch’s membrane is a complex pentalaminar extracellular matrix composed of the RPE basement membrane, inner collagenous layer, middle elastic layer, outer collagenous layer, and the choriocapillaris basement membrane. This specialized membrane is critical for maintaining special diffusional capabilities between the RPE and choriocapillaris. The choroid is composed of three layers including the choriocapillaris, medium vessels, and large vessels. The choriocapillaris is the main oxygen source for the photoreceptors.
The health of each of these layers contributes to specific diseases of the macula. This tissue, on a histopathological basis, responds in predictable fashion that can be clinically useful. With discovery of the molecular pathogenetic causes of macular disease, a new spectrum of diseases may become amenable to surgical management. The purpose of this chapter is to describe the basic histopathologic responses of the RPE-Bruch’s membrane-choroidal complex, how the overlay of aging can affect this response, and how new discoveries may influence our surgical management of the macula in the near future.
GENERAL PATTERNS OF PATHOLOGIC CHANGE TO THE RETINAL PIGMENTED EPITHELIUM
Regardless of the stimulus, the RPE responds to a stress in several stereotypic pathologic ways that have clinical and surgical relevance. Understanding these responses will help the clinician better diagnose and manage RPE disease. In response to insult or disease, the RPE can undergo hypertrophy, metaplasia, migration, or hyperplasia. More advanced or sustained insults can result in atrophy and, ultimately, cell death. The RPE is normally a cuboidal epithelium. The apical region is rich in microvilli to help with the phagocytosis of POSs. The basal region has infoldings to increase surface area for the exchange of materials with the choriocapillaris. The RPE is packed with melanin granules to improve visual resolution and neutralize photo-oxidative stress. It is rich with mitochondria because of the high metabolic demands.
Hypertrophic RPE cells become enlarged. Melanin granules are enlarged without increasing in their number. The result is a dark, jet-black appearance to the RPE. Hyperplastic RPE cells are the result of proliferation and migration. The cell number is increased. For example, RPE cell hyperplasia can appear as “bony spicules” in a perivascular configuration when they migrate into the neurosensory retina along retinal vasculature.
The RPE is a postmitotic simple epithelium that typically undergoes little cell division. However, in response to injury, inflammation, or another similar stimulus, it can undergo metaplasia. A classic example is during a retinal tear where RPE cells are dislodged from its monolayer and released into the vitreous cavity where they can attach to the vitreous and develop a fibroblastic phenotype. With altered cell-matrix interactions, the cell undergoes migration, metaplasia, and proliferation. Crypopexy can exaggerate this process by introducing inflammation and cellular injury that augment this response. Clinically, the consequence is an epiretinal membrane, or in severe cases, proliferative vitreoretinopathy membranes can develop on the inner and outer surface of the neurosensory retina and induce a tractional retinal detachment.
RETINAL PIGMENTED EPITHELIUM ATROPHY AND APOPTOSIS IN AGING AND AGE-RELATED MACULAR DEGENERATION
With aging, the RPE undergoes progressive degeneration that can lead to atrophy. The RPE loses its normal cuboidal morphology to become irregular in shape and flattened, or atrophic. The atrophic cells contain fewer pigment granules than normal RPE (2, 3 and 4). Clinically, this can be visualized by hypopigmentation in affected areas of the macula (Fig. 2-1).
Ultrastructurally, aging atrophic RPE cells contain few pure melanin granules, and instead, are filled with melanolipofuscin and melanolysosomes (5). Many of these changes are due to the relentless accumulation of lipofuscin, or insoluble biomolecules that arise from incomplete digestion of phagocytosed POSs beginning in the first or second decade of life (6,7). Lipofuscin is contained within lysosomes. Remarkably, lipofuscin can occupy up to 19% of the RPE cell by the time the patient is 80 years of age (7). The RPE basal infoldings are fewer in number, and some RPE can be detached from the RPE basement membrane, due to the development of basal laminar deposits (BlamD) or accumulations of heterogeneous material between the RPE and its basement membrane.
With flat mount sections, the RPE cells appear hexagonal and more densely packed in the macula than in the periphery in young eyes, but they lose their hexagonal shape and resemble nonfoveal RPE with aging (8). Young macular RPE has a homogeneous cell density that becomes heterogeneous with aging (9). Overall, the number of RPE cells in the macula declines with aging and age-related macular degeneration (AMD), although this number is more variable than in young maculas. The major pathophysiologic mechanism for the cell loss is apoptosis. Del Priore et al. showed that the proportion of apoptotic human RPE cells increases significantly with age (10). The apoptotic RPE are confined mainly to the macula, and account for the preferential decrease of RPE cells in the macula. Dunaief et al. showed that RPE cell loss in AMD is due to apoptosis (11). Apoptotic cells are seen in areas of RPE atrophy at the edge of geographic atrophy. The factors that cause RPE cells to enter apoptosis are unclear, but at present include oxidative stress, photo-oxidative stress, lipid accumulation, or uncontrolled complement mediated inflammation.
CHANGES TO BRUCH’S MEMBRANE WITH AGING AND AGE-RELATED MACULAR DEGENERATION
Coincident with atrophy and potentially loss of the RPE, Bruch’s membrane undergoes a series of histopathologic changes. The hallmark change of aging and AMD is the development of basal deposits, or accumulation of heterogeneous debris. The location and composition of basal deposits define whether the changes are due to aging or age-related disease (Fig. 2-2). As early as in the second decade of life, the outer collagenous layer accumulates homogenous material (12). In addition, accumulations between the RPE and its basement membrane known as BlamD also develop with aging. If the accumulations become thick and contain heterogeneous debris such as inflammatory proteins, cellular fragments, lipoproteins, and “long spacing collagen,” they are a marker for AMD. The accumulation of apolipoprotein B100 containing lipoproteins within the inner collagenous layer during aging is a recent discovery of significant importance (13). Its accumulation most likely creates a “lipid” barrier restricting the normal diffusional characteristics of Bruch’s membrane that can lead to injury to the RPE (14). Importantly, these lipoproteins have been hypothesized to induce basal linear deposit (BlinD) formation, or accumulations in the inner collagenous layer. BlinDs are one of the strongest histopathological markers for AMD. Clinically, basal deposits are typically not visualized. However, a later stage change is the appearance of drusen, yellowish deposits that are the clinical hallmark of aging and AMD (15).
CONSEQUENCES OF SUBRETINAL FLUID
Visual recovery after rhegmatogenous retinal detachment is negatively influenced by subretinal fluid under the macula. While there are direct adverse effects on the neurosensory macular retina, the subretinal fluid clearly alters the RPE, which can contribute to a poor outcome. In experimental models of rhegmatogenous retinal detachment, RPE migration and proliferation are observed within a day. The proliferation occurs at the interface between the RPE apex and photoreceptor tips (16). The RPE also undergoes morphologic alterations including retraction of apical microvilli, which are typically in contact with the photoreceptors, and a rounding of
the cells at the apex (17). These proliferative and metaplastic changes to the RPE can adversely influence the recovery of the photoreceptors, and when there are changes to the RPE, the morphologic recovery of the RPE is protracted and correlates with the slow recovery of vision. Clinically this is manifested by macular pigmentary changes. Hackett et al. examined RPE migration and proliferation by adding blood-free subretinal fluid to RPE cells in culture (18). Several variables were examined for correlation with migration and proliferation, including age and sex of the patient; extent, duration, and height of the detachment; protein content of the fluid; and amount of cryopexy. They found that retinal cryopexy enhanced RPE cell migration activity in subretinal fluid, while RPE proliferative activity increased with the size and duration of detachment. While the vitreoretinal surgeon has little control over the size and duration of any retinal detachment, these results suggest that the surgeon should judiciously use cryopexy during retinal reattachment surgery.
the cells at the apex (17). These proliferative and metaplastic changes to the RPE can adversely influence the recovery of the photoreceptors, and when there are changes to the RPE, the morphologic recovery of the RPE is protracted and correlates with the slow recovery of vision. Clinically this is manifested by macular pigmentary changes. Hackett et al. examined RPE migration and proliferation by adding blood-free subretinal fluid to RPE cells in culture (18). Several variables were examined for correlation with migration and proliferation, including age and sex of the patient; extent, duration, and height of the detachment; protein content of the fluid; and amount of cryopexy. They found that retinal cryopexy enhanced RPE cell migration activity in subretinal fluid, while RPE proliferative activity increased with the size and duration of detachment. While the vitreoretinal surgeon has little control over the size and duration of any retinal detachment, these results suggest that the surgeon should judiciously use cryopexy during retinal reattachment surgery.
The overlay of aging changes to the RPE on recovery from subretinal fluid or another insult can predictably impair the recovery process and restoration of visual acuity. For example, several groups have shown that advanced age negatively influences visual recovery after repair of a macula off retinal detachment (19, 20 and 21).
GENETIC DISEASES
The RPE can be the target of specific genetic diseases. Mutations in the RPE65 gene result in Leber Congenital Amaurosis. RPE65 is involved in retinoid metabolism. It catalyzes the critical step in the visual cycle converting all-trans-retinyl ester to 11-cis-retinol that permits normal photoreceptor function and vision. Its absence results in undetectable rhodopsin, rod photoreceptor dysfunction, the development of inclusions in the RPE, and subsequent retinal degeneration. 9-cis-retinal can restore visual pigmentation and function in mice. One intriguing observation is that patients with this mutation have disproportionately greater photoreceptor layer thickness than cone photoreceptor function (22). This highlights the fact that the primary abnormality lies in the RPE and is distinct from primary retinal degenerations where the photoreceptor layer is thin, consistent with lost photoreceptor function. Theoretically, it could indicate that therapeutic rescue is possible in the late stages of the disease if the photoreceptor layer remains relatively intact.
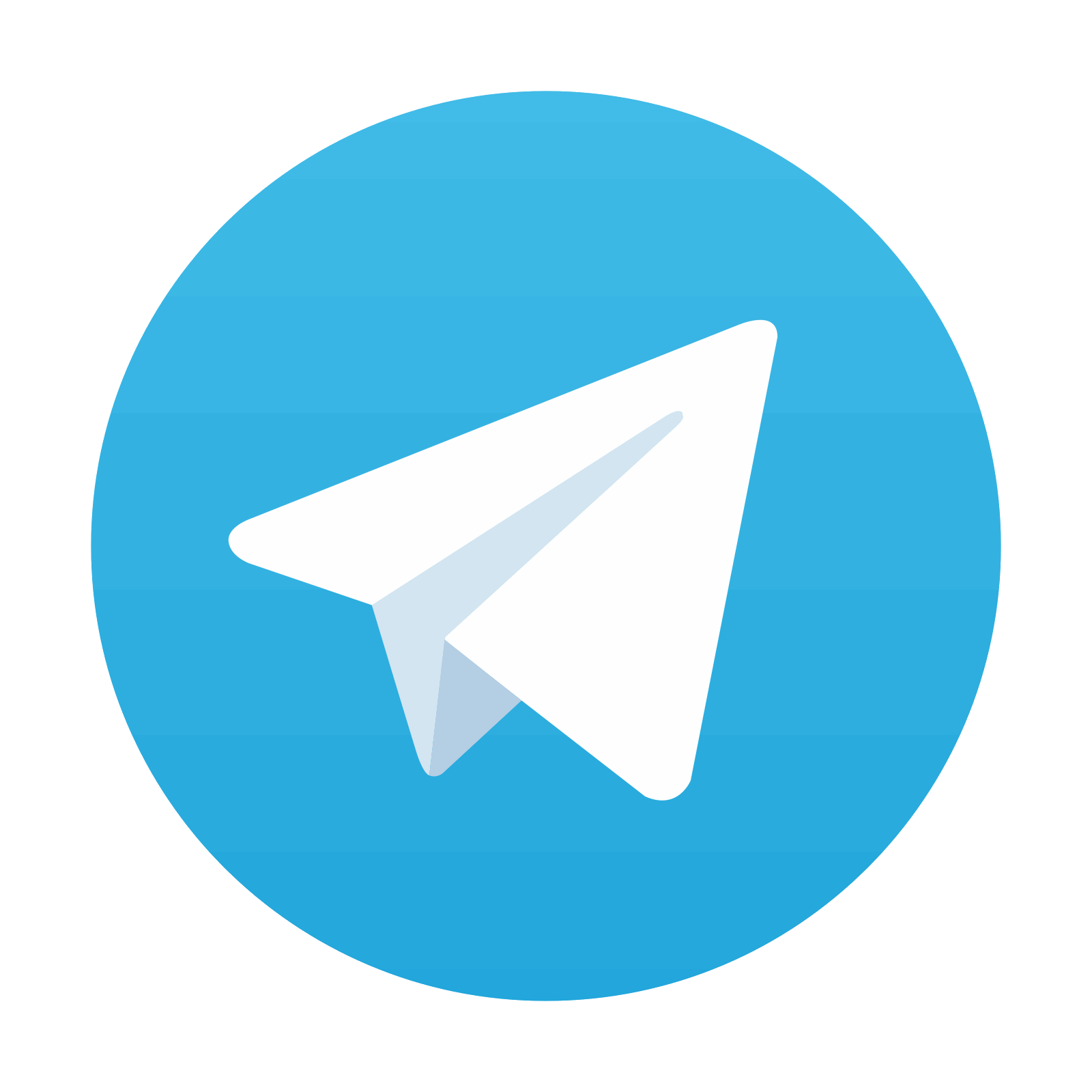
Stay updated, free articles. Join our Telegram channel

Full access? Get Clinical Tree
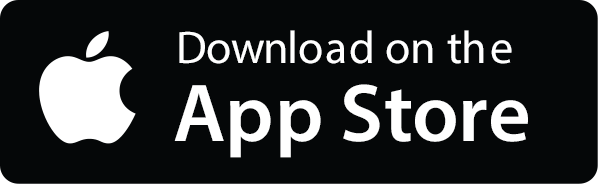
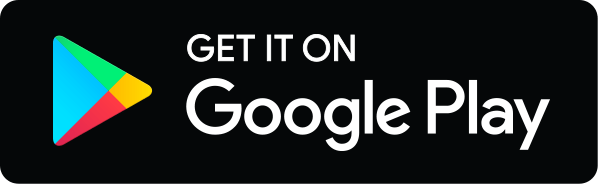