Pathophysiology of the Blood-Retinal Barrier
Pablo Carnota
Rafael Bueno
Monica Rodriguez-Fontal
John B. Kerrison
INTRODUCTION
In order to maintain the unique microenvironment needed for viability and optimal function of retinal cells, there is a system of dynamic equilibrium adjustments and regulation mechanisms. Akin to the central nervous system, homeostatic regulation is facilitated by a restrictive permeability system known as blood retinal barrier (BRB). Like blood brain barrier (BBB) in brain, BRB restricts the accessibility to the retina of circulating factors such as lipophilic compounds, blood gases, and certain molecules that depend upon active transport.
BRB is known to be disrupted in several pathological situations such as retinal microangiopathies (e.g., diabetic retinopathy), inflammatory-infectious diseases (e.g., Irvine-Gass syndrome) and other exudative conditions (e.g., age-related macular disease (ARMD) or central serous chorioretinopathy (CSC)). This breakdown leads to disturbances of tissue microenvironment, which subsequently accelerates the progression of the pathology (1,2).
INNER VERSUS OUTER BLOOD RETINAL BARRIER
The BRB is composed of two anatomically and functionally different structures, the inner and the outer BRB. The inner BRB is formed by the endothelial cells of retinal capillaries. The outer BRB is formed by the retinal pigment epithelium (RPE).
Inner Blood Retinal Barrier
Endothelial cells of retinal capillaries display distinctive characteristics in comparison with capillaries in other locations such as lack of fenestrations and presence of apical structures known as tight junctions or zonulae occludentes (Fig. 3-1). These structures not only confer a very restrictive permeability at the intercellular clefts but allow close regulation of transendothelial transport as well as maintenance of endothelial polarity. This phenotypic expression of endothelial cells is dynamic and can be altered, as discussed below, by the local extracellular matrix, soluble growth factors, and heterotypic (other) and homotypic (same) cellular interactions via intercellular junctions. The lumen (5 to 6 µm) of retinal capillaries is created by a single layer of endothelial cells surrounded by intramural pericytes and encompassed by a common basement membrane (3, 4 and 5). Pericytes have several functions such as regulation of vascular tone, mechanical support of the vessel wall, production of extracellular matrix, and phagocytosis (6,7). Pericytes exhibit a 1:1 ratio with endothelial cells in normal human retinal capillaries (8), and their pseudopod-like processes encircle the retinal capillary and contain contractile proteins such as smooth muscle type alpha-actin, nonsmooth muscle actin and smooth muscle myosin (6,9) (Fig. 3-2). Additionally, pericytes release extracellular components, such as fibronectin, which provide anchorage points for mechanical force transfer during capillary lumen constriction (6).
Tight junctions are formed by a complex network of proteins that importantly can be regulated and dynamically altered by different conditions and pathologies. There are three types of transmembrane proteins within tight junctions: occludins, claudins, and junctional adhesion molecules (JAM). The transmembrane proteins bind junctional proteins in adjacent cells and create the actual barrier. Other proteins have been identified such as intracellular protein ZO-1 and cadherin, the main component of another type of junction between cells known as adherens junction.
Occludin was the first identified membrane component of tight junctions (10) (Fig. 3-3). However, subsequent studies demonstrated that occludin was not essential for the tight junction assembly and rather was involved in signal transduction of endothelial cells (11,12). Actually, other studies suggested that the main role of occludin could be the control of
paracellular flux, mainly of ions and small organic cations (13, 14 and 15). Thus, occludin contributes both structurally and as signal transducers and regulators of barrier properties. Two studies on diabetic retinas and on bovine retinal endothelial cells treated with vascular endothelial growth factor (VEGF) have revealed decreased occludin content concomitant with increased BRB permeability (16,17). Another protein, tricellulin, has recently been identified as a protein localized specifically at regions where three cells make contact (18). Together, these proteins create the final subset of known transmembrane tight junction proteins.
paracellular flux, mainly of ions and small organic cations (13, 14 and 15). Thus, occludin contributes both structurally and as signal transducers and regulators of barrier properties. Two studies on diabetic retinas and on bovine retinal endothelial cells treated with vascular endothelial growth factor (VEGF) have revealed decreased occludin content concomitant with increased BRB permeability (16,17). Another protein, tricellulin, has recently been identified as a protein localized specifically at regions where three cells make contact (18). Together, these proteins create the final subset of known transmembrane tight junction proteins.
Later, claudins were found to be other components of tight junctions (19) (Fig. 3-4). Accumulative evidence has revealed that they are key molecules in the tight junction assembly by playing a very important role in the control of ion flux (20, 21, 22, 23, 24 and 25). However, the complexity of the system is only beginning to be understood. Claudins are a multigene family of more than 20 members (26, 27 and 28), and the pattern of expression of different claudin family members varies among tissue types, which confers tissue-specific properties to tight junctions (20). Claudins expressed in endothelial cells of neural (and retinal) tissue are claudin-1, claudin-3, claudin-5, and claudin-12 (28, 29 and 30). They are suggested to be candidate molecules responsible for structural endothelial barrier function as shown by a study of claudin-5-deficient mice that disclosed that claudin-5 is indispensable for the barrier function of neural blood vessels for small molecules (29). Alterations in claudins have been associated with a variety of disease states (31, 32). Mutations in claudin 16 exemplify the role of claudins in barrier selectivity since it has been demonstrated as the cause of familial hypomagnesemia with hypocalcemia and nephrocalcinosis (33).
Another integral membrane protein of tight junctions is JAM found at the tight junctions of epithelial and endothelial cells. The exact function of JAM is still unclear. It has been demonstrated that transfection of JAM into epithelial cells does not reconstitute tight junctions strands (34). However, it contributes to tight junctions assembly and cell-to-cell adhesion in epithelial and endothelial cells (35, 36, 37 and 38) and contributes to extravasation of monocytes through endothelial monolayers (38).
Zonula occludens or ZO proteins are intracellular proteins associated with the cytoplasmic surface of tight junctions. The first ZO protein recognized, ZO-1, is a transmembrane protein that acts as a scaffold due to its multiple binding domains; its several domains bind to transmembrane tight junction proteins occludin, claudin, and ZO-2 and to adherens junction proteins afadin and cadherin (39, 40, 41, 42, 43, 44, 45 and 46) (Fig. 3-5). ZO-1 presence can readily be observed in both retinal vascular endothelial cells and RPE. In both cell types, agents that induce permeability such as VEGF or hepatocyte growth factor induce redistribution of ZO-1 from the cell border to the cell interior (47,48). In contrast, increasing barrier properties increases ZO-1 border staining. Elevated hydrostatic pressure across bovine aortic endothelial cells first increases water and solute flux, which then decreases over the next 30 to 60 minutes, reaching a new equilibrium; ZO-1 can readily be seen to increase at plasma membrane during this adaptive response (49,50). Therefore ZO-1 acts as a central organizer of the tight junction complex.
There is another type of junction between endothelial cells called adherens junction. Adherens junctions are characterized by the expression of cadherins (Ca++-dependent
transmembrane adhesion proteins) whose function consists of maintaining a space between cell membranes, while allowing homotypic cell contact (51).
transmembrane adhesion proteins) whose function consists of maintaining a space between cell membranes, while allowing homotypic cell contact (51).
Within this microenvironment several soluble vasoactive molecules have been identified as playing a more or less important role in the regulation of permeability of the inner BRB. Molecules such as nitric oxide, adenosine or CO2 or hypoxia cause retinal pericytes relaxation whereas other molecules such endothelin-1, angiotensin II, ATP or hyperoxia lead to pericyte contraction.
Tissue oxygen concentration is known to influence the expression of various molecules including growth factors, cytokines, and enzymes (52). Hypoxia stimulates cellular production of erythropoietin (53), VEGF (54), tyrosine hydroxylase (55), phosphoglycerate kinase 1, and lactate dehydrogenase A (56). Many of these hypoxia-sensitive molecules are implicated in adaptive processes of organisms to hypoxic circumstances such as erythropoiesis, angiogenesis, increase in respiratory volume and conversion of the metabolism to an anaerobic state. In addition, changes in expression of certain oxygen-sensitive molecules can also trigger the progression of disease processes in patients with cerebral ischemic diseases, diabetic retinopathy and other diseases (57). Among components of tight junctions, occludin, claudin-1, claudin-3 and claudin-5 are reported to be hypoxia-sensitive molecules and promote breakdown of the neural endothelial barrier (52,58 and 59).
Endothelial cells synthesize nitric oxide in response to several stimuli like increased local shear force, bradykinins, insulin-like growth factor 1, acetylcholine, thrombin and various platelet products (60, 61 and 62). Nitric oxide diffuses to the pericyte and smooth muscle cell surface, where it binds to guanylate cyclase, causing intracellular cGMP accumulation and vasodilation (63,64). In addition to its vasodilatory actions, nitric oxide protects vessels by inhibiting platelet aggregation, platelet granule secretion, leukocyte adhesion, and possibly smooth muscle cell proliferation (65). However, high levels of nitric oxide can induce retinal toxicity through neuronal nitric oxide synthase, which may be important during retinal ischemia, degenerative retinal disease, and diabetic vascular dysfunction (66, 67, 68, 69, 70 and 71).
Endothelins are released by endothelial cells on the abluminal side of capillary and bind to receptors on adjacent pericytes and smooth muscle cells (72). Endothelins (ET1, ET2, and ET3) are the most potent vasoconstrictor agents currently known (73). ET-dependent contraction is mediated by three receptor subtypes (ETR A, B, and C) (74). Human studies have shown that vasoconstriction following intravenous injection of ET1 is mediated through ETR-A (75). In general, ETR-A mRNA is localized to pericytes and smooth muscle cells, whereas ETR-B mRNA can be found also in endothelial cells. Animal studies have shown that stimulation with ET1 on pericytes causes contraction, aggregation of actin fibers, and proliferation (76, 77 and 78).
Vascular endothelium generates basal levels of superoxide anions but can be stimulated to produce deleterious amounts of these molecules during many pathologic conditions (79). Superoxide anions inactivate nitric oxide, thereby inhibiting vasodilatory capacity. This pathologic inhibition may mediate vascular changes found in hypertension, hypercholesterolemia, retinopathy of prematurity, diabetes, and ischemia/reperfusion injury (79,80). Superoxide anions may induce endothelial damage resulting in a prothrombotic state; this pathologic mechanism may contribute to diabetic retinopathy (81).
VEGF stimulates occludin phosphorylation through activation of the protein kinase C (PKC) signaling pathway. Binding of VEGF to its tyrosine kinase receptor activates PKC signal transduction pathway and this activation has been correlated to VEGF’s mitogenic effects in endothelial cells (82). In a recent study on bovine retinal epithelium cells, Harhaj et al. showed that VEGF stimulates occludin phosphorylation increasing the endothelial permeability, an effect that can be inhibited using PKC inhibitors or by expressing a dominant negative PKC-bII mutant (83). However, only part of VEGFinduced permeability was prevented by classical PKC inhibitors
indicating additional pathways are likely involved in the regulation of permeability.
indicating additional pathways are likely involved in the regulation of permeability.
Another regulator of vascular permeability is the reninangiotensin system. Human and animal ocular tissues locally express the necessary components (renin, angiotensinogen and angiotensin-converting enzyme, ACE) for an intrinsic renin-angiotensin system (84, 85, 86, 87 and 88). ACE bound to endothelial cell luminal surface rapidly converts angiotensin I to angiotensin II; ACE itself inactivates bradykinin and angiotensin II stimulates retinal vasoconstriction via smooth muscle cells and pericytes (89
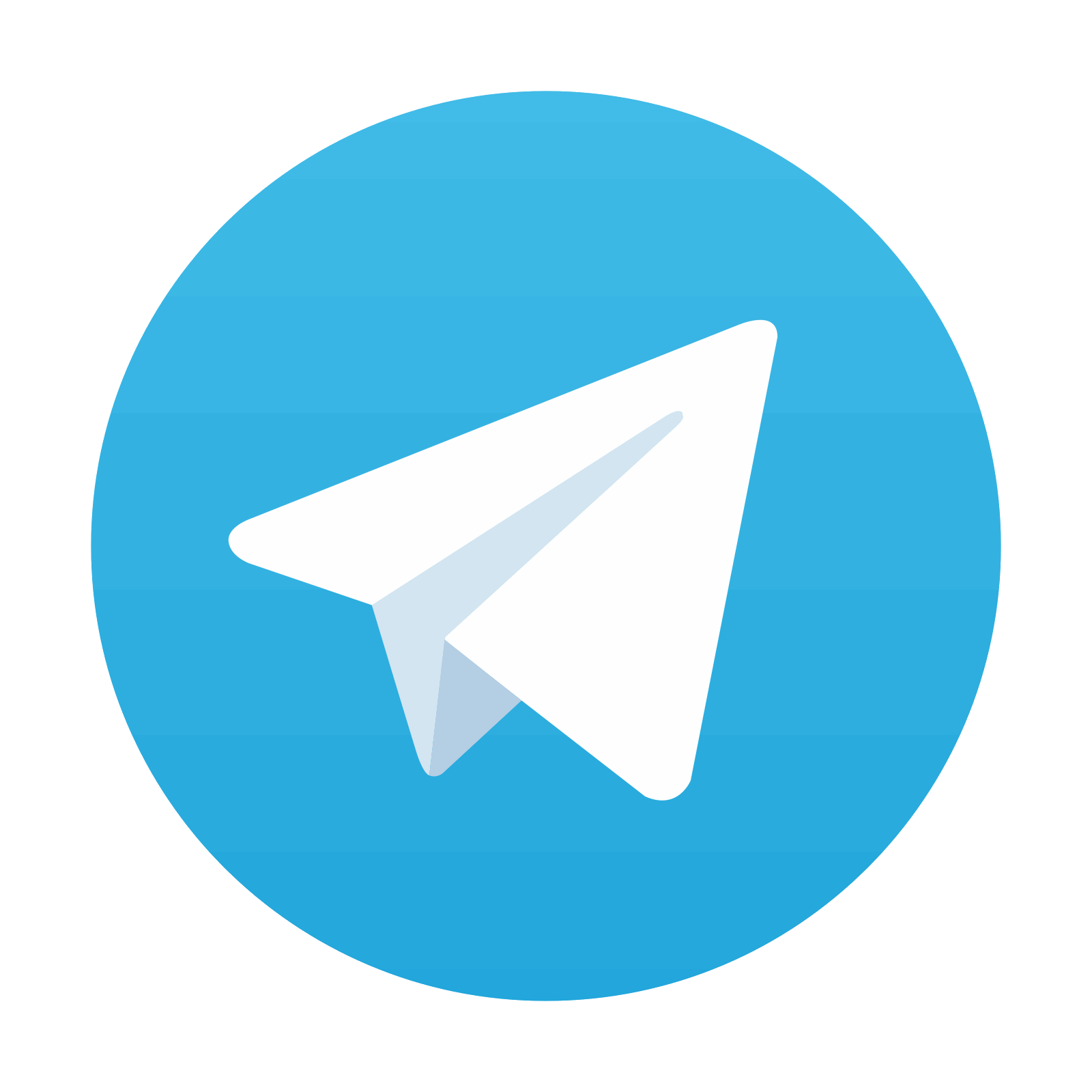
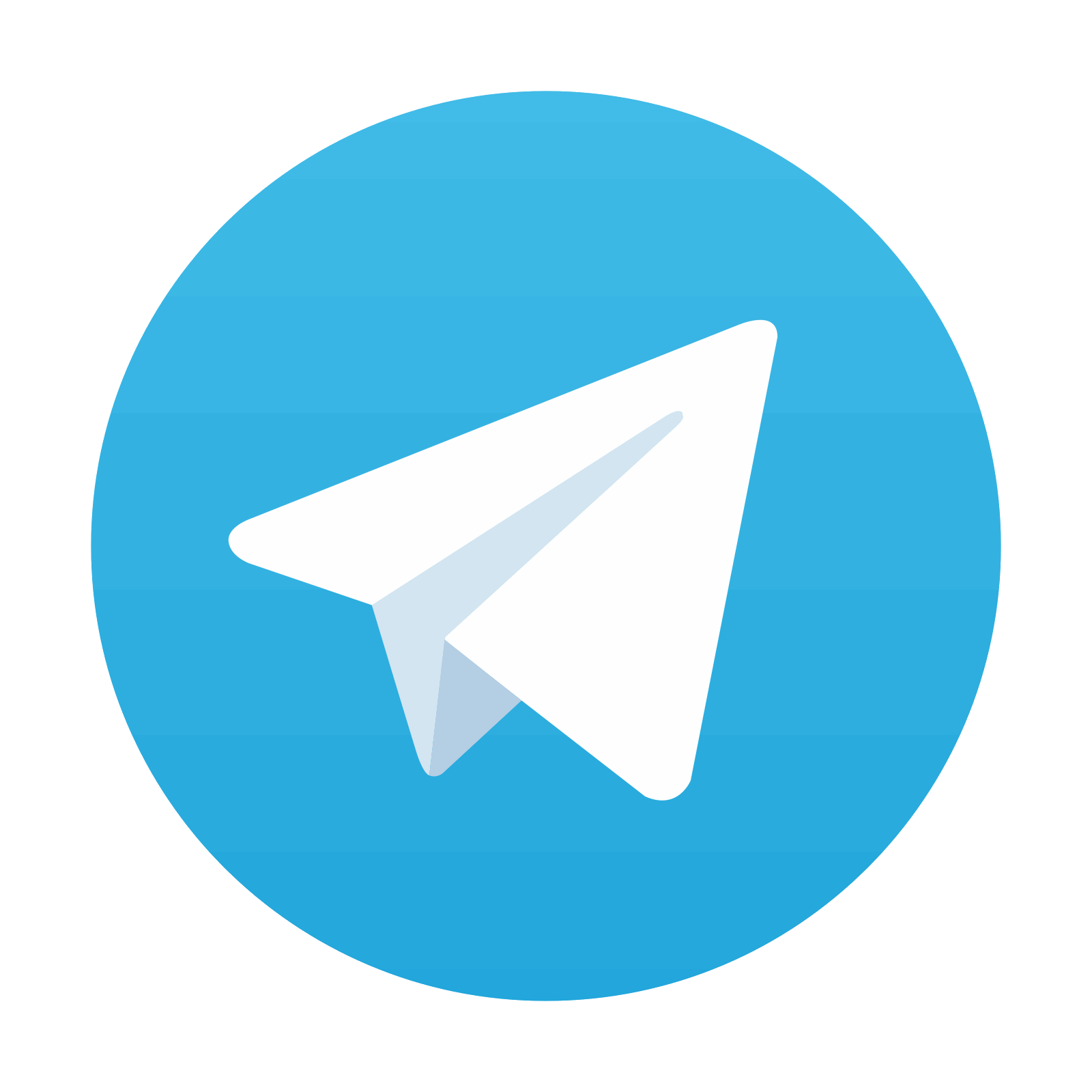
Stay updated, free articles. Join our Telegram channel

Full access? Get Clinical Tree
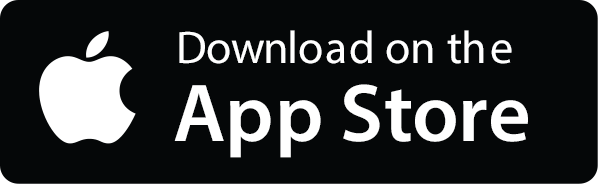
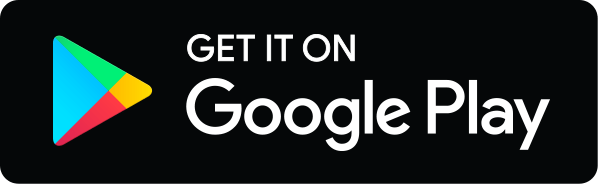
