The Orbit as a Closed Bony Compartment
The orbit consists of several bony structures. The orbital content is extensively surrounded by a bony shell, which while it gives protection against external incidences on the other hand means that increase of intraorbital pressure can only be very slightly mitigated. There is only the aditus orbitalis through which the orbital structures can expand in order to reduce the pressure. An increase of the intraorbital—especially the retrobulbar—pressure leads to an anterior displacement of the eye bulb, but the resulting decrease in the pressure is very limited. An increase of intraorbital pressure presents a hazard to the optic nerve, although the nerve itself with its axons is usually not damaged by increased pressure. The damage and functional impairment that can be observed arises more from compression of the vessels that supply the dural sheath with blood, and from compression of the nutritive vessels of the nerve itself, with consequent ischemia. As well as this, traction forces act on muscles and the optic nerve following an anterior displacement of the eye bulb. Both aspects will be discussed later in more detail.
Anatomically the optic nerve can be divided into four sections ( ▶ Fig. 2.1):
An intraocular section at the sclera nerve crossover.
An intraorbital section.
An intracanalicular section.
An intracranial/chiasmal section.
Fig. 2.1 Four sections of the optic nerve. The potential for injury to the nerve is different in each section.
The hazardous potential for the optic nerve differs considerably between the sections. A fairly resistant layer of connective tissue is located at the crossover from the sclera to the optic nerve, which decreases its elasticity. Intense rotations of the eye bulb may cause axon damage in this transition zone and can lead to avulsion trauma or to axonotmesis. 1, 2 The potential risk for injury of the optic nerve is relatively low in the intraorbital section, partly because its course is slightly curved, which protects the nerve from traction forces when the eye bulb is dislocated anteriorly, and partly because the nerve is covered by fat tissue in this segment, which acts as a pressure pad and, together with the cerebrospinal fluid, protects the nerve. The optic nerve canal is the narrowest site in the nerve course: the anulus of Zinn is located in this area at the aditus of the nerve canal, which is the starting point for the inner orbital muscles and which has only little elasticity. In this bony channel the nerve cannot compensate increased pressure. It is additionally at risk from avulsion injuries due to skull base fractures, which can pass through the orbit apex and through the optic nerve canal. Injuries of the optic nerve in its intracranial and chiasmal part are rare. In this area the effects of skull base tumors, inflammatory diseases of the central nervous system, or ischemia and tumors of the brain, which can lead to visual impairment, are more important.
There are basically four pathomechanisms, which can be distinguished according to damage to the intraorbital structures and the optic nerve:
Orbital trauma.
Inflammation with infiltration of the nerve.
Tumors.
Increase of the intraorbital pressure, for example, due to acute hemorrhage into the orbit or due to Graves’s ophthalmopathy.
These various pathomechanisms lead clinically to functional impairment, which may manifest in a disturbance of eye bulb motility or—more frequently—in loss of vision.
2.3 Intraorbital Inflammation
Inflammation can affect the structures of the inner orbit in two different ways: as an acute inflammation, or as a chronic inflammation that is often generated by autoimmune processes that can lead, for example, to demyelinization of nerve fibers in cases of disseminated encephalomyelitis. 3, 4 The optic nerve represents an extension of the diencephalon. Its axons are surrounded by oligodendroglia, which increases the velocity of nerve conduction but hampers nerve regeneration following severe inflammation or trauma. This anatomical feature is the reason why diseases of the central nervous system can spread to the optic nerve. 5, 6
The orbital complication of acute sinusitis is a classic example of an inflammation that can spread to the optic nerve, with resulting blindness (see Chapter ▶ 6). In stage III or IV of the disease, diffuse spread of the inflammation into the internal orbital structures can occur with consequent orbital phlegmon, which can lead to an acute neuritis of the optic nerve. The nerve axons can be directly affected by the inflammatory processes. However, this is less likely, because the nerve is covered by a dural sheath: it is rather more likely in the first instance that the meninges surrounding the nerve and their nutritive blood vessels are affected by acute inflammation. 7
Bacterial inflammations result in a number of highly complex reactions of the affected tissue. These pathogen–host cell interactions can be caused by the bacteria themselves as agents (direct adherence) as well as by toxins expressed by the bacteria (endotoxins) 8 ( ▶ Fig. 2.2). Which of these mechanisms predominates depends very much on the bacteria. For example, the pore-forming exotoxin S. aureus α-toxin is expressed by Staphylococcus aureus. At the target cell this toxin leads to the formation of a pore ca. 1 nm in size by interaction with lipid chains and other integral membrane proteins. This pore represents a hydrophilic canal, which allows an ion flow. 7, 9 Consequently, disturbance of the barrier function of the cell membrane occurs; in addition there is activation of particular signal pathways, which will be detailed later in this chapter. As a result, vasoactive substances are expressed by the toxin-exposed endothelial cells, such as prostacyclin (PGI2) and nitric oxide ( ▶ Fig. 2.2), which dilate blood vessels. Calcium ions (Ca2+) play a key role in some of this expression. 7 Activation of the complement cascade occurs parallel to these events. Activated endothelial cells also lose their anticoagulative function, which leads to increased blood clotting. 9 The bacterium Streptococcus pneumonia, which is most frequently identified in orbital complications of acute sinusitis, also expresses an exotoxin (pneumolysin) that has quite similar effects.
Fig. 2.2 Scheme of pathophysiological events when host cells are affected by microbial pathogens. Cell reactions can be triggered by expression of toxins as well as by direct adherence.
The vascular endothelium of the dura and of the nerve’s nutritive vessels plays a fundamental role in the process of an inflammatory reaction. The gathering of bacteria on endothelial cells leads to attachment of the pathogen (adherence). Some surface structures of the pathogen are involved in this process, which imitate endogenous ligands on the endothelial cells. For example, Staphylococcus aureus binds to fibronectin on endothelial cells and to extracellular matrix proteins (e.g., vitronectin; elastin). 9 The inhibition of the β2-integrin-mediated recruitment of inflammatory cells, such as neutrophil granulocytes, is a result of the effects of bacterial adhesion molecules 9, 10 ( ▶ Fig. 2.2).
Bacteria carry molecular structures on their surface (such as lipopolysaccharides, LPS) that can be recognized by the host cells via receptors. Common representatives of these receptors are the toll-like receptors (TLR), which have been intensively investigated and play an essential role in the recognition of microorganisms. 11 An upregulation of TLR, particularly of TLR-2 and TLR-4, activates signal pathways in which tyrosine kinases, mitogen activated protein kinases (MAPK), and transcription factors (e.g., nuclear factor κB [NF-κB]) are involved, 9, 11, 12 leading to recruitment of granulocytes and expression of proinflammatory cytokines (e.g., tumor necrosis factor α). Activation of NF-κB can be caused by bacteria, cytokines, or chemical or physical stimuli. It is normally present inactively in the cytosol of the cell but following activation it reaches the nucleus, where it contributes to the expression of different genes, which are called “early response genes”. 8, 12
Toll-like receptor-mediated activation of host cells by microbial pathogens also lead to stimulation of GTP-binding Rho-proteins (e.g., RhoA). 11, 13 Among other things, Rho proteins regulate functions of cell contraction and cell migration, of phagocytosis, and activity of transcription factors. 9 Along with NF-κB, the p38 mitogen activated protein kinase (p38MAPK), which controls angiogenetic processes as well as proliferation, migration, and cell permeability, serves as a central control unit in acute inflammatory processes. 9, 12, 14
Contact with a microbial pathogen leads first to an unspecific immune response, which is dominated by phagocytosing leukocytes (polymorphonuclear granulocytes; macrophages). Activation of leukocytes can be divided into different stages: (1) the “rolling” stage (in which the cells “roll” at the endothelial surface), (2) tight adhesion to endothelial cells, and (3) transmigration into subendothelial tissue. 9 Several biokines are involved in these processes:
Integrins, particularly of group β. As well as cell-to-cell interaction they mediate important functions of the cell–matrix-interaction, and are of particular importance in both acute and chronic inflammations, such as in middle ear cholesteatoma. 15
Adhesion factors such as E-selectin, P-selectin, intercellular adhesion molecules (ICAM-1 and ICAM-2), which act as ligands for β-integrins, as well as the vascular cell adhesion molecule (CD106 = VCAM), which controls the rolling and adherence of leukocytes on the endothelial surface.
The pathophysiological processes described above, along with products of stimulated granulocytes (oxygen radicals; proteases) constitute a disturbance of endothelial barriers, with increased permeability. 16 Blood plasma extravasated into the tissue and corpuscular blood constituents lead to edema but can also lead to exacerbation of the inflammation. Hypoxia or acidosis of the tissue caused, for example, by edema can also impede the regular metabolism and can therefore contribute to a progression of the pathological process. The vascular endothelial growth factor (VEGF), which can also initiate processes of angiogenesis, might play a key role in edema formation. 9, 12
The severity of clinical inflammation symptoms—edema and blood vessel constriction, later blood vessel dilatation—is considerably influenced by this endothelial activation. 9
Leukocytes (polymorphonuclear granulocytes; macrophages) that migrate into the tissue begin to phagocytose bacteria. Attachment of complement system factors at the bacterial wall predisposes to this process (opsonization). Several specific immunoglobulins that activate the complement system are involved in this. Opsonized bacteria are recognized as “foreign bodies” by the phagocytes and ingested by endocytosis. 9 Pneumococcus has a polysaccharide capsule that prevents complement attachment. For phagocytosis, interleukin-6 (IL-6) is expressed by macrophages.
In a subsequent step or in parallel to this, there is expression of several cytokines, particularly of interleukins 1β, 2, 6, 12 (IL-1β, IL-2, IL-6, IL-12), tumor necrosis factor α (TNF-α), transforming growth factor β (TGF-β), interferons, fibrinogen, bradykinin, and cytokines arising from arachidonic acid metabolism (prostaglandins, particularly PGE2; prostacyclins, particularly PGI2) ( ▶ Table 2.1). These cytokines are expressed by endothelial cells, by macrophages or polymorphonuclear granulocytes, and lead to an accentuation of the immune response (IL-1β, IL-2, IL-12), to vasodilation (bradykinin), or to increased vessel permeability (PGI2; PGE2) ( ▶ Fig. 2.3). As a result, there is first a local disturbance of blood supply with ischemia, which is caused by arteriole spasm and venule constriction. Responsible for these processes are several prostaglandins, along with kinins, which lead to thrombocyte aggregation, to exudation of fluid into the soft tissue, and finally to ischemia. Increase in permeability is influenced strongly by histamine, serotonin, and prostaglandins. 9, 12, 14
Cytokine | Site of expression | Important function |
NF-κB | Macrophages |
|
Histamine | Mast cells basophile Granulocytes |
|
IL-1β | Monocytes |
|
IL-2 | T-helper cells |
|
IL-6 | Endothelial cells Macrophages |
|
IL-12 | Monocytes Macrophages |
|
TNF-α | Macrophages |
|
TGF-β | Macrophages Endothelial cells |
|
Bradykinin | Endothelial cells |
|
Prostaglandins (PGE3; PGI2) | Endothelial cells Macrophages (from arachidonic acid metabolism) |
|
Fig. 2.3 Scheme of the interaction between pathogens (microbial pathogen) and host cells. There is either a cellular reaction mediated by leukocytes or a vascular reaction based on endothelial activation. Release of inflammatory biokines and hyperpermeability result in edema, ischemia, acidosis, and loss of function.
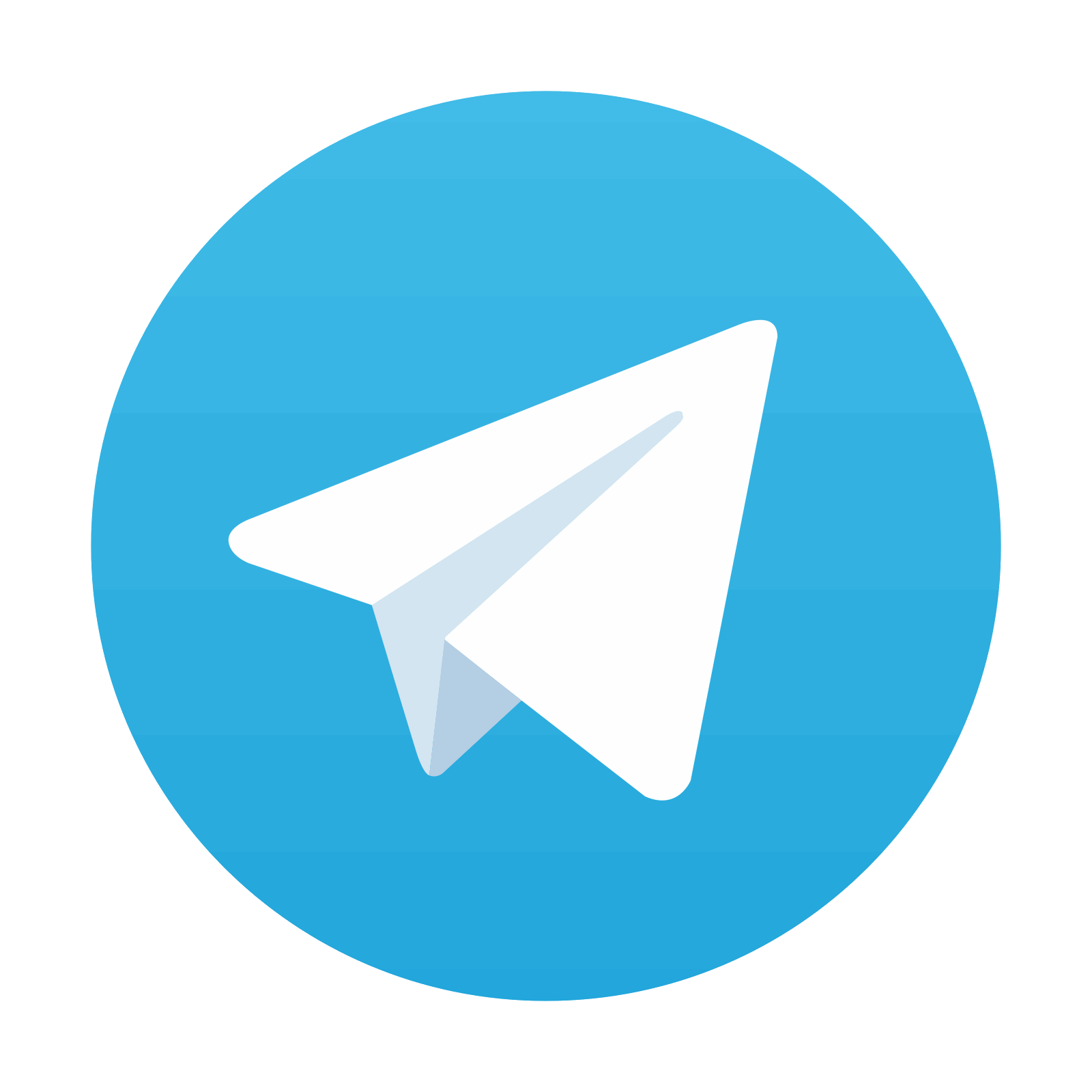
Stay updated, free articles. Join our Telegram channel

Full access? Get Clinical Tree
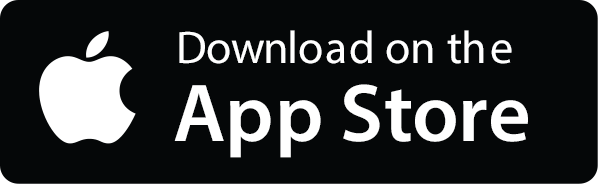
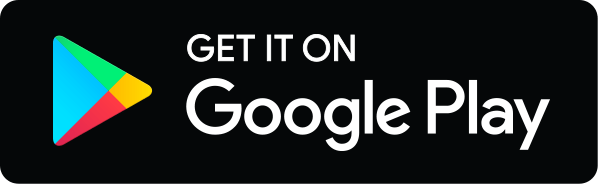