Purpose
To describe ultrastructure and immunocytochemistry of internal limiting membrane peelings after unsuccessful treatment with ocriplasmin, to compare with untreated eyes, and to correlate with clinical imaging data.
Design
Interventional comparative case series.
Methods
Internal limiting membrane specimens were removed from 10 eyes with small macular holes and vitreomacular traction during vitrectomy after intravitreal ocriplasmin injection without release of traction or closure of macular holes during follow-up. Based on optical coherence tomography analysis, specimens from 10 other eyes without ocriplasmin treatment served as controls. All specimens were processed as flat mounts for phase-contrast microscopy followed by immunolabeling for fluorescence microscopy and embedding in epoxy resin with serial sectioning for transmission electron microscopy.
Results
Despite the absence of contractive epiretinal membranes on optical coherence tomography, we found epiretinal cells and vitreous collagen fibrils on the internal limiting membrane in specimens removed from eyes with and without previous pharmacologic vitreolysis. Immunolabeling revealed glial cells and hyalocytes in macular holes, whereas myofibroblasts were predominant in vitreomacular traction. There was no apparent damage of the vitreoretinal interface after unsuccessful pharmacologic vitreolysis compared to untreated controls.
Conclusions
Epiretinal cell proliferation and vitreous collagen fibrils with close adhesions to the internal limiting membrane are not always detectable by optical coherence tomography or may not have been recognized. Since they are associated with unsuccessful ocriplasmin treatment, presence and topography of epiretinal cells and vitreous collagen remnants on the internal limiting membrane should be further elucidated in order to refine criteria and indications for case selection in pharmacologic vitreolysis.
Intravitreal injection of ocriplasmin for the treatment of vitreomacular traction is currently one of the most debated issues among vitreoretinal surgeons. Pharmacologic vitreolysis with ocriplasmin (Jetrea; ThromboGenics, Leuven, Belgium) was approved by the US Food and Drug Administration in October 2012 for patients with symptomatic vitreomacular adhesion and by the European Medicines Agency for the treatment of vitreomacular traction, including association with a macular hole ≤400 μm in diameter. Vitreomacular adhesion is defined as adhesion of the vitreous to the macula within a 6-mm central retinal field surrounded by elevation of the posterior vitreous cortex in the context of incomplete posterior vitreous detachment. Persistent vitreomacular adhesion leads to vitreomacular traction, thereby exerting tractional forces onto the macula that contribute to vision loss and metamorphopsia.
At times when ocriplasmin was not available, symptomatic vitreomacular adhesion and vitreomacular traction alone or in association with a macular hole required surgical intervention. Today, traction release or closure of macular holes can be achieved in up to 50%–70% of cases after a single intravitreal injection of ocriplasmin (0.125 mg/0.1 mL), as recently confirmed by subgroup analyses in eyes with vitreomacular traction.
Ocriplasmin is a recombinant truncated form of the human serine protease plasmin and exerts proteolytic activity on laminin and fibronectin, both major components of the vitreoretinal interface. In animal models and human donor eyes, ocriplasmin was consistently shown to rapidly induce posterior vitreous detachment with an internal limiting membrane devoid of any collagen fibrils.
However, there is no detailed information on structure of the vitreoretinal interface following intravitreal ocriplasmin injection in clinical practice. Given that absence of contractive epiretinal membranes increases the success rate of pharmacologic vitreolysis, pathologic analysis of the vitreomacular interface after ocriplasmin treatment is of particular importance for pathophysiological understanding, and for clinical case selection before intravitreal injection of ocriplasmin. The aim of this study was to describe the ultrastructure and immunocytochemistry of the internal limiting membrane surgically removed following unsuccessful pharmacologic vitreolysis with ocriplasmin compared to untreated eyes, and to correlate the observations obtained with previously recorded clinical imaging data in a clinicopathologic case series.
Methods
This is an interventional comparative case series of surgically excised internal limiting membrane specimens obtained from 10 eyes after unsuccessful pharmacologic vitreolysis with ocriplasmin. We included 10 patients who underwent vitrectomy with internal limiting membrane peeling at the Department of Ophthalmology, Ludwig-Maximilians-University, between October 1, 2013 and May 31, 2014. Four eyes showed persistent small idiopathic macular holes (diameter <250 μm) and 6 eyes presented with persistent symptomatic vitreomacular traction as confirmed by high-resolution optical coherence tomography. Macular surgery followed ocriplasmin injection after a mean period of 2.5 months (range 1.0–4.5 months). Pharmacologic vitreolysis with ocriplasmin was applied according to guidelines of the German Ophthalmological Society, the Retina Society, and the Professional Association of German Ophthalmologists for therapeutic intravitreal application of ocriplasmin. For clinical analysis, patients’ records were reviewed for age, sex, preoperative and postoperative best-corrected visual acuity, period of follow-up, and pre- and postoperative state of the lens. Visual acuity was measured best-corrected with the decimal system, and then converted to logarithm of minimal angle of resolution (logMAR) units for the statistical analysis. The Institutional Review Board and the Ethics Committee of the Ludwig-Maximilians-University Munich approved the retrospective review of the patients’ data as well as the histopathologic preparation and analysis of the patients’ specimens (No. 471-14). Informed consent was obtained from each patient. This study was conducted according to the tenets of the Declaration of Helsinki.
Based on optical coherence tomography, matched specimens from 10 other eyes of 10 other patients without ocriplasmin treatment were selected and served as controls; they had been operated at times when ocriplasmin was not available. In the control group, we also included specimens removed from 4 eyes with small idiopathic macular holes and 6 eyes with vitreomacular traction that underwent vitrectomy between February 1, 2011 and December 31, 2011. All control specimens were selected from our Register of Electron Microscopy and have been removed from eyes meeting the indication criteria for pharmacologic vitreolysis, retrospectively.
For high-resolution spectral-domain optical coherence tomography analysis (SpectralisOCT; Heidelberg Engineering, Heidelberg, Germany), we used volume B-scans of baseline and follow-up examinations. Maximal macular hole diameter and maximal length of basis of vitreomacular adhesion were reevaluated using the calliper function.
Surgical Procedure and Specimen Removal
The surgical technique consisted of a 23 gauge 3-port pars plana vitrectomy with peeling of eventual epiretinal membranes and the internal limiting membrane. All patients were operated by 2 experienced surgeons. Vitrectomy was performed as follows. Before opening of the infusion line, the status of the posterior hyaloid was determined using a plano-concave contact lens. If the vitreous was attached or partially attached to the retina, posterior vitreous detachment was induced by suction with the vitrectomy probe over the optic disc or in the midperiphery. The posterior hyaloid was detached from the retina and excised up to the periphery. To improve the precision of internal limiting membrane peeling and to avoid incomplete removal, brilliant blue G (0.2 mL, brilliant peel; Geuder, Heidelberg, Germany) was used to visualize the internal limiting membrane. No other dye was applied. The dye solution was injected onto the vitreomacular interface and immediately washed out. Peeling of the internal limiting membrane was performed using end-gripping Eckardt forceps. If necessary, removal of the internal limiting membrane was performed en bloc with epiretinal tissue. Surgery was completed by checking the retinal periphery for breaks. In eyes with small macular holes, the vitreous cavity was perfused with a mixture of 15% C 2 F 6 following a fluid-air exchange, with the pressure being set at 35 mm Hg. In eyes with persistent vitreomacular adhesion, balanced salt solution was left in the vitreous cavity at the end of the procedure. The removed internal limiting membrane specimens were harvested and immediately placed into a mixture of 2% paraformaldehyde and 0.1% glutaraldehyde for fixation.
Immunocytochemistry
Interference microscopy and indirect immunocytochemistry was performed of all specimens after flat-mount preparation. There was no selection of specimens before the preparation procedure. If more than 1 internal limiting membrane specimen was removed from 1 eye, all specimens were prepared and analyzed. If fewer than 3 internal limiting membrane specimens were removed, large specimens were segmented in order to allow for labeling combinations of all 9 primary antibodies.
Flat-mount preparation of the whole internal limiting membrane specimens was performed on glass slides with antifading mounting medium 4′,6-diamidino-2-phenylindole (DAPI) (ImmunoSelect; Dianova, Hamburg, Germany) for cell nuclei staining. A cover slide was added. After photodocumentation with the fluorescence microscope (Leica DM 2500; Leica, Wetzlar, Germany) at magnifications between ×50 and ×400, specimens were processed for immunocytochemical staining.
Staining procedures were performed as follows. Specimens were rinsed in 0.1 M phosphate-buffered saline at pH 7.4 before incubation with 0.1% pepsin. After the specimens were rinsed again, they were incubated with the primary antibodies (1:50) overnight at room temperature. Nine primary antibodies were used for glial cells (anti–glial fibrillary acidic protein and anti-vimentin; DAKO, Hamburg, Germany), hyalocytes (anti-CD45 and anti-CD64; Santa Cruz Biotechnology, Heidelberg, Germany), myofibroblasts (anti-α-smooth muscle actin and anti-collagen type I; Santa Cruz Biotechnology), vitreous cortex collagen (anti-collagen type II; Biotrend, Cologne, Germany), and adhesion glycoproteins (anti-laminin and anti-fibronectin; DAKO). After a washing procedure, the secondary antibodies (donkey anti-mouse CY3, donkey anti-rabbit CY2, and donkey anti-goat CY5; Dianova, Hamburg, Germany) were added together (1:100) for 2 hours at room temperature. Finally, specimens were rinsed in phosphate-buffered saline again, and then postfixation with 2% glutaraldehyde followed.
Preparation of negative controls was performed in 2 different manners. In the first, primary antibodies were substituted with diluent, and specimens were incubated with the secondary antibody alone. In the second, the primary antibody was substituted with isotype control antibodies (IgG2a and IgG1, monoclonal mouse antibodies; DAKO). All other procedures were identical to the procedures illustrated above.
Transmission Electron Microscopy
Postfixation in osmium tetroxide 2% and uranyl acetate, as well as dehydration in graded concentrations of ethanol with embedding in Epon 812, followed. Semithin sections of 1000 nm were stained with an aqueous mixture of 1% toluidine blue and 2% sodium borax. Ultrathin sections of 60 nm were obtained by series-sectioning, and were contrasted with uranyl acetate and lead citrate. Ultrastructural imaging and analysis were performed by 2 masked observers independently using a Zeiss EM 900 transmission electron microscope (Zeiss, Jena, Germany).
Results
Clinical Data Analysis
Following pharmacologic vitreolysis with ocriplasmin, we included 3 right eyes and 7 left eyes from 3 women and 7 men who required vitrectomy for full-thickness macular holes and persistent vitreomacular traction. As presented in Table 1 , patients’ age ranged from 55 years to 88 years (mean, 71.3 ± 11.5 years; median, 73 years). Eyes with macular holes were operated after a mean period of 2.0 months following ocriplasmin injection (range, 1.0–3.5 months; median, 1.0 months), whereas eyes with vitreomacular traction were operated after a mean period of 2.8 months (range, 1.5–4.5 months; median, 2.8 months). Follow-up period ranged from 3 to 15 months (mean, 9.5 months; median, 8 months).
No | Sex / Age | Diagnosis | Previous Ocriplasmin Injection (+/−) | Months Between Ocriplasmin Injection and Vitrectomy | BCVA Before Ocriplasmin Injection (LogMAR) | Preoperative BCVA (LogMAR) | Postoperative BCVA (LogMAR) | Preoperative Status of the Lens | Postoperative Status of the Lens | Follow-up (Months) |
---|---|---|---|---|---|---|---|---|---|---|
1 | M/71 | MH | + | 3.5 | 1.0 | 0.8 | 0.1 | Phakic | IOL | 14 |
2 | F/55 | MH | + | 1.0 | 0.4 | 0.5 | 0.5 | Phakic | IOL | 6 |
3 | F/58 | MH | + | 1.0 | 0.5 | 1.0 | 0.5 | Phakic | IOL | 7 |
4 | M/57 | MH | + | 2.5 | 0.4 | 0.6 | 0.1 | Phakic | IOL | 10 |
5 | M/88 | VMT | + | 2.0 | 0.7 | 1.3 | 1.3 | IOL | IOL | 13 |
6 | M/84 | VMT | + | 3.0 | 0.5 | 0.4 | 0.3 | IOL | IOL | 15 |
7 | M/75 | VMT | + | 2.5 | 0.7 | 1.0 | 0.5 | Phakic | IOL | 3 |
8 | M/78 | VMT | + | 3.5 | 0.2 | 0.5 | 0.0 | Phakic | IOL | 11 |
9 | F/78 | VMT | + | 1.5 | 1.0 | 1.3 | 0.6 | IOL | IOL | 8 |
10 | M/69 | VMT | + | 4.5 | 0.4 | 0.4 | 0.1 | Phakic | IOL | 8 |
11 | F/74 | MH | − | – | – | 0.7 | 0.1 | IOL | IOL | 31 |
12 | F/68 | MH | − | – | – | 1.0 | 0.7 | IOL | IOL | 36 |
13 | M/60 | MH | − | – | – | 0.5 | 0.0 | Phakic | IOL | 8 |
14 | F/72 | MH | − | – | – | 1.0 | 0.1 | Phakic | IOL | 12 |
15 | M/70 | VMT | − | – | – | 0.3 | 0.2 | IOL | IOL | 26 |
16 | F/66 | VMT | − | – | – | 0.7 | 0.2 | IOL | IOL | 14 |
17 | F/69 | VMT | − | – | – | 0.4 | 0.6 | IOL | IOL | 22 |
18 | F/85 | VMT | − | – | – | 0.4 | 0.1 | Phakic | IOL | 12 |
19 | M/71 | VMT | − | – | – | 0.5 | 0.0 | IOL | IOL | 10 |
20 | F/72 | VMT | − | – | – | 0.3 | 0.1 | Phakic | IOL | 6 |
In eyes with macular holes, mean best-corrected visual acuity (logMAR) before intravitreal injection of ocriplasmin was 0.57 ± 0.29 (median 0.45). Mean best-corrected visual acuity (logMAR) after intravitreal injection of ocriplasmin and before vitrectomy was 0.72 ± 0.22 (median 0.70). Mean best-corrected visual acuity (logMAR) at last follow-up was 0.3 ± 0.23 (median 0.30). In eyes with vitreomacular traction, mean best-corrected visual acuity (logMAR) before intravitreal injection of ocriplasmin was 0.58 ± 0.28 (median 0.60). Mean best-corrected visual acuity (logMAR) after intravitreal injection of ocriplasmin and before vitrectomy was 0.82 ± 0.43 (median 0.75). Mean best-corrected visual acuity (logMAR) at last follow-up was 0.46 ± 0.47 (median 0.40). Overall, in eyes following ocriplasmin treatment best-corrected visual acuity mostly improved during the follow-up period, as seen in 7 patients; remained unchanged in 2 patients; and decreased in 1 patient. All patients were pseudophakic at time of last follow-up. Patient 2 experienced a retinal detachment 1 week after ocriplasmin injection. Patient 3 had persistent macular hole and required secondary vitrectomy 2 months after primary surgery. Patient 5 had an additional diagnosis of age-related macular degeneration, and required intravitreal application of ranibizumab during the follow-up period. Patient 6 presented with persistent macular edema after internal limiting membrane peeling. Adverse events such as acute vision loss or subretinal fluid accumulation have not been seen in this series.
In the matched control group, we included 5 right eyes and 5 left eyes corresponding to 7 women and 3 men who primarily underwent vitrectomy for full-thickness macular holes and vitreomacular traction at times when ocriplasmin was not available. Patients’ age ranged from 60 years to 85 years (mean, 70.7 ± 6.4 years; median, 70 years). Follow-up period ranged from 6 to 36 months (mean, 17.6 months; median, 13 months).
In eyes with macular holes from the control group, mean best-corrected visual acuity (logMAR) before vitrectomy was 0.80 ± 0.24 (median 0.85). Mean best-corrected visual acuity (logMAR) at last follow-up was 0.22 ± 0.32 (median 0.10). In eyes with vitreomacular traction from the control group, mean best-corrected visual acuity (logMAR) before vitrectomy was 0.43 ± 0.15 (median 0.40). Mean best-corrected visual acuity (logMAR) at last follow-up was 0.20 ± 0.21 (median 0.15). Mostly, best-corrected visual acuity improved during the follow-up period, as seen in 9 patients, and decreased in 1 patient. All patients were pseudophakic at time of last follow-up. Patient 12 showed persistent macular hole and refused further surgery. Patient 15 presented with secondary epiretinal cell proliferation and required surgery with internal limiting membrane peeling again. Patient 17 was seen with persistent macular edema after internal limiting membrane peeling.
Optical Coherence Tomography and Cell Distribution Analysis
In the group of eyes treated with ocriplasmin, high-resolution optical coherence tomography analysis revealed a mean macular hole diameter of 162 ± 55 μm (median, 171 μm; range, 95–211 μm) before intravitreal application of ocriplasmin. Although all eyes with macular holes showed resolution of vitreomacular adhesion after intravitreal injection of ocriplasmin, the hole diameter of all treated eyes with macular holes enlarged to a mean of 342 ± 82 μm (median, 339 μm; range, 245–445 μm) after attempted pharmacologic vitreolysis, as presented in Table 2 .
No | Previous Ocriplasmin Injection (+/−) | MH Diameter or Basis of VMT Before Ocriplasmin Injection (μm) | MH Diameter or Basis of VMT Before Vitrectomy (μm) | Presence of Eccentric Epiretinal Cell Proliferation | Area of Peeled ILM (mm 2 ) | Number of Cells | Cell Density (Cells/mm 2 ) | Cell Distribution | ||
---|---|---|---|---|---|---|---|---|---|---|
Single Cells | Homogenous Layer of Cells | Cluster of Cells | ||||||||
1 | + | 211 | 245 | + | 7.3 | 3041 | 419 | – | x | – |
2 | + | 203 | 331 | − | 14.6 | 167 | 11 | x | – | – |
3 | + | 139 | 445 | − | 13.7 | 188 | 14 | x | – | – |
4 | + | 95 | 347 | − | 1.7 | 0 | 0 | – | – | – |
5 | + | 587 | 615 | + | 5.5 | 785 | 142 | – | – | x |
6 | + | n.a. | 1358 | + | 3.03 | 185 | 61 | – | – | x |
7 | + | 349 | 325 | + | 5.1 | 91 | 18 | x | – | – |
8 | + | 622 | 591 | + | 7.0 | 235 | 34 | x | – | – |
9 | + | 359 | 316 | + | 5.9 | 817 | 140 | – | x | – |
10 | + | n.a. | 1204 | + | 4.4 | 191 | 44 | x | – | – |
11 | − | – | 236 | − | 12.9 | 465 | 36 | – | – | x |
12 | − | – | 128 | + | 15.0 | 1282 | 85 | – | x | – |
13 | − | – | 82 | − | 13.1 | 626 | 48 | – | x | – |
14 | − | – | 140 | + | 13.2 | 1153 | 88 | – | – | x |
15 | − | – | 1262 | + | 8.2 | 1565 | 191 | – | x | |
16 | − | – | 1328 | + | 7.0 | 1057 | 150 | – | x | |
17 | − | – | 936 | − | 3.0 | 97 | 32 | x | – | – |
18 | − | – | 356 | − | 7.0 | 101 | 14 | – | – | x |
19 | − | – | 825 | + | 10.4 | 1688 | 162 | – | x | – |
20 | − | – | 1189 | + | 11.4 | 2554 | 224 | – | x | – |
In vitreomacular traction cases, we measured a mean base of vitreomacular traction of 478 ± 145 μm (median, 473 μm; range, 349–622 μm). In 2 eyes with vitreomacular traction, optical coherence tomography data before ocriplasmin treatment was lacking because patients were injected with ocriplasmin elsewhere. After treatment with ocriplasmin, base of vitreomacular traction was measured with a mean of 735 ± 444 μm (median, 602 μm; range, 316–1358 μm).
In eyes treated with ocriplasmin, retrospective analysis of all optical coherence tomography scans revealed presence of a thin eccentric epiretinal membrane in 7 of 10 eyes (1 eye with macular hole, 6 eyes with vitreomacular traction) before vitrectomy ( Table 2 , Figure 1 ). In 3 of these eyes, there was a thin foveal epiretinal proliferation detected before surgery that had not been seen before ocriplasmin injection. In control group eyes without ocriplasmin treatment, we found eccentric epiretinal cell proliferation in 6 of 10 eyes (2 eyes with macular holes, 4 eyes with vitreomacular traction) before vitrectomy.
In eyes with ocriplasmin treatment before surgery, area of peeled internal limiting membrane ranged from 1.7 mm 2 to 14.6 mm 2 (mean, 6.8 ± 4.2 mm 2 ; median, 5.5 mm 2 ). Cell density ranged between none and 419 cells/mm 2 (mean, 88 ± 126 cells/mm 2 ; median, 39 cells/mm 2 ). The cells were mostly arranged in a single-cell fashion on the internal limiting membrane. In addition to single cells, 2 specimens showed homogenous layer of cells and 2 other presented with clusters of cells.
In control group eyes without previous ocriplasmin treatment, high-resolution optical coherence tomography analysis revealed a mean macular hole diameter of 146 ± 64 μm (median, 134 μm; range, 82–236 μm) before vitrectomy and a mean base of vitreomacular traction of 983 ± 363 μm (median, 1062 μm; range, 356–1328 μm). The area of peeled internal limiting membrane was measured between 3.0 mm 2 and 15.0 mm 2 (mean, 10.1 ± 3.7 mm 2 ; median, 10.1 mm 2 ). Cell density ranged between 14 cells/mm 2 and 224 cells/mm 2 (mean, 103 ± 73 cells/mm 2 ; median, 86 cells/mm 2 ). The cells were mostly arranged as a homogenous layer of cells. In 1 eye single-cell distribution was seen, and in 3 other eyes clusters of cells were found.
Immunocytochemical Analysis
Analysis of flat-mounted specimens showed no differences in immunoreactivity comparing specimens removed after previous ocriplasmin treatment and specimens without ocriplasmin treatment ( Table 3 , Figure 2 ). Rather, we found differences between eyes with macular holes and eyes with vitreomacular traction.
Anti- | Target Structure | Immunoreactivity a | |||
---|---|---|---|---|---|
ILM Specimens With Previous Ocriplasmin (N = 10) | ILM Specimens Without Previous Ocriplasmin (N = 10) | ||||
MH (N = 4) | VMT (N = 6) | MH (N = 4) | VMT (N = 6) | ||
Glial fibrillary acidic protein | Glial cells | ++ | + | ++ | + |
CD45 | Hyalocytes | (+) | ++ | + | ++ |
Alpha-smooth muscle actin | Intracellular actin filaments | (+) | + | (+) | + |
Collagen type I | Newly formed collagen | + | + | + | + |
Collagen type II | Vitreous cortex collagen | (+) | ++ | + | ++ |
Laminin | Gylcoprotein | + | + | + | + |
Fibronectin | Glycoprotein | + | + | ++ | ++ |
MMP-2 | Matrix metalloproteinase-2 | − | − | − | − |
MMP-9 | Matrix metalloproteinase-9 | + | + | + | + |
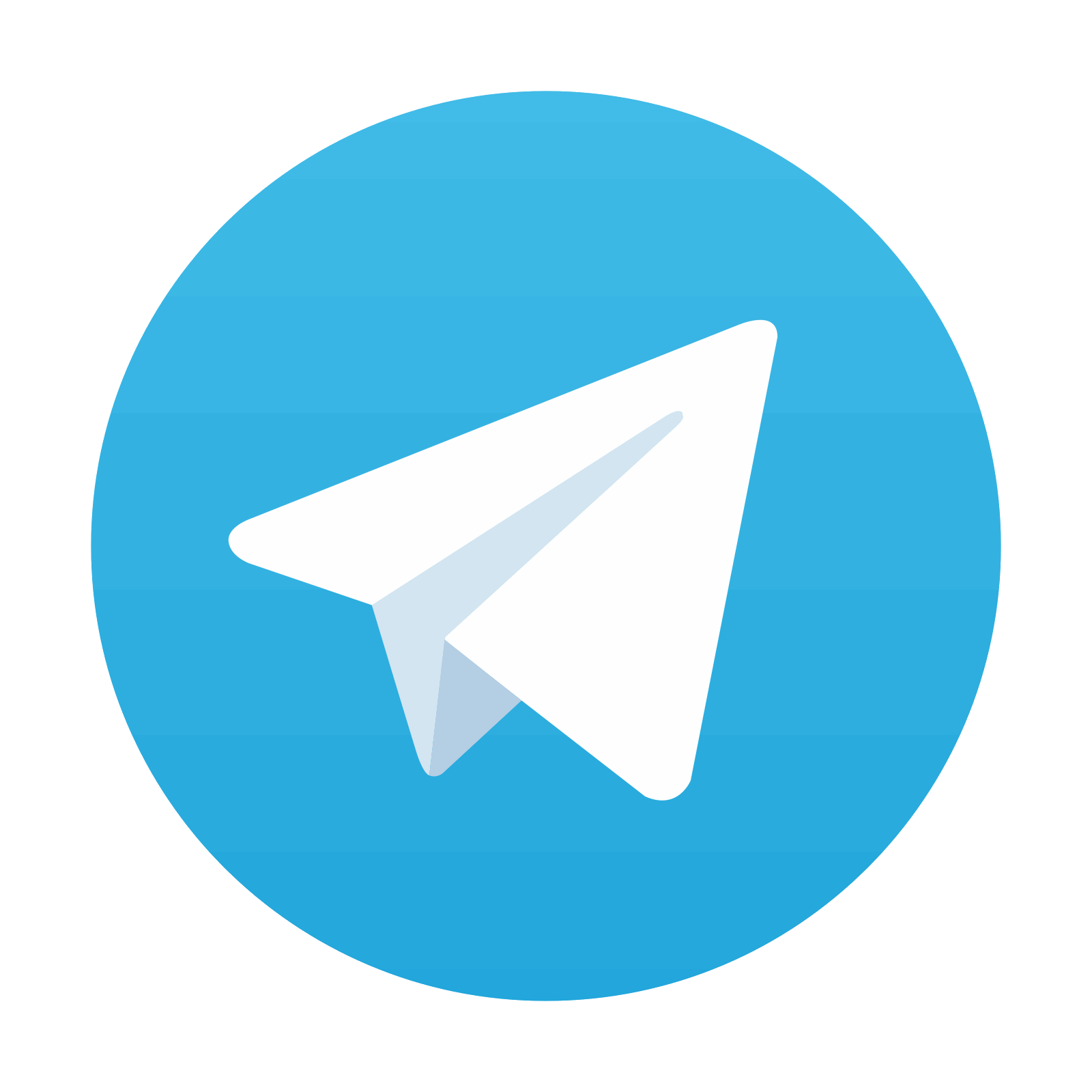
Stay updated, free articles. Join our Telegram channel

Full access? Get Clinical Tree
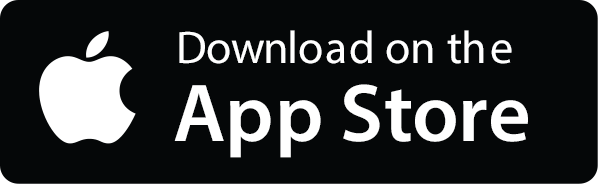
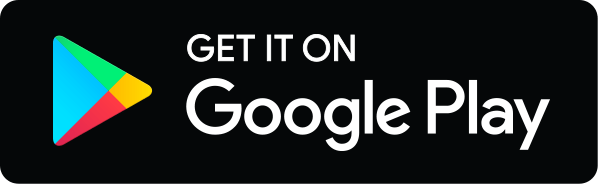
