Optical Coherence Tomography Systems for Angiography
Gangjun Liu, PhD; Alex D. Pechauer, BS; David Huang, MD, PhD; and James G. Fujimoto, PhD
Optical coherence tomography angiography (OCTA) detects blood flow by using motion contrast, computing the changes of optical coherence tomography (OCT) signals introduced by moving blood cells between repeated cross-sectional images (B-frames) at the same location. Acquisition of typical volumetric OCTA data requires high-speed OCT technology. Fourier domain OCT (FD-OCT) systems have inherently higher detection sensitivity, so they can achieve a much higher speed than time domain OCT systems (TD-OCT).1–4 The high speed of FD-OCT allows multiple cross-sectional images to be rapidly acquired at the same location. Therefore, 3-dimensional volumetric OCTA data can be obtained in several seconds, making it practical for clinical imaging. Subsequent processing of images produced by FD-OCT systems allows the visualization of vascular networks down to the capillary level in both the retinal and choroidal circulations. In FD-OCT systems, the interferogram can be measured either with a broadband light source and spectrometer, a technique known as spectral domain OCT (SD-OCT), or as a function of time with a wavelength sweeping laser source and a detector, known as swept source OCT (SS-OCT).
SPECTRAL DOMAIN OPTICAL COHERENCE TOMOGRAPHY
In SD-OCT, a broadband light source is used, similar to traditional TD-OCT systems. The OCT signal and reference beams have a relative time delay determined by the path length difference (Δz) given by the depth of the tissue structure relative to a “zero delay” set by the fixed reference path (Figure 1-1). The interference of the signal and reference beams will have a spectral modulation or oscillation that can be measured using the spectrometer and high-speed line scan camera. The periodicity of this oscillation will be inversely proportional to the echo time delay Δz. Larger echo delays will produce higher frequency spectral oscillations. The echo delays can be measured by Fourier transforming the interference signal. This produces an axial scan measurement (A-scan) of the magnitude vs echo delay of the backreflected/backscattered light from the tissue. SD-OCT can also be understood by noting that an interferometer acts like a periodic frequency filter, where the filter period depends on the path difference Δz between the sample and reference paths. Spatial features are encoded in frequency, similar to magnetic resonance imaging. Because all the light echoes are detected simultaneously, sensitivity is dramatically higher than TD-OCT, in which the light echoes are detected sequentially.
Figure 1-1. Broadband light is split by a beam splitter into reference and sample beams. A fixed reference mirror establishes a zero delay. Backscattered light from the reference path and sample interfere at the beam splitter. The interference spectrum is spatially dispersed by a diffraction grating and detected with a high-speed line scan camera.
SWEPT SOURCE OPTICAL COHERENCE TOMOGRAPHY
SS-OCT is another type of FD detection. SS-OCT uses a narrow linewidth light source that is swept in time over a broadband wavelength (Figure 1-2). The OCT signal and reference beams have a relative time delay determined by the path length difference (Δz) given by the depth of the tissue structure relative to a “zero delay” set by the fixed reference path. The interference of the signal and reference beams produces an oscillation or beat frequency because of the frequency difference between the 2 light waves. Different echo time delays of light are encoded as different oscillation frequencies at the output of the interferometer as the laser is frequency swept. A high-speed photodetector is used, without requiring a spectrometer or line scan camera. The oscillation frequency is related to the echo time delay Δz, with larger echo delays producing higher frequency oscillations. Similar to SD-OCT detection, in SS-OCT the echo magnitude vs delay, or A-scan, can be measured by Fourier transforming the detector signal that is acquired over one frequency sweep of the light source. Because each frequency sweep generates one A-scan, the imaging rate is determined by the sweep repetition rate of the light source. SS-OCT can also be understood by noting that the frequency sweep of the light source essentially labels different times with different frequencies of the light.
Depth Range and Sensitivity Roll-Off
Since increasing imaging speeds reduces OCT signal levels, high sensitivity is important for achieving the high speeds necessary for OCTA. FD-OCT using SD-OCT or SS-OCT is 10 to 100 times more sensitive than TD-OCT. However, FD-OCT systems typically have a sensitivity that depends on depth Δz. There is a “sensitivity roll-off” where the sensitivity decreases for structures that are further away from the zero delay position. This can be understood by recalling that the echoes that are further from zero delay correspond to finer oscillations in SD-OCT (see Figure 1-1) or higher frequency interference in SS-OCT (see Figure 1-2). In SD-OCT, the spectrometer has limited resolution and is less effective in detecting higher frequency spectral oscillations that correspond to echoes that are further from zero delay (see Figure 1-1). In SS-OCT, the spectral purity or linewidth of the swept light source determines how far from zero delay the interference between the OCT signal and reference frequency sweeps can be detected (see Figure 1-2). Figure 1-3 shows the sensitivity roll-off performance of SD-OCT and SS-OCT by comparing images of the same retina at different positions relative to zero delay.5 SS-OCT usually has less sensitivity roll-off than SD-OCT because the spectrometers used in SD-OCT have limited spectral resolution.
In addition, SS-OCT instruments typically have a longer imaging range than SD-OCT instruments. This is because detecting light echoes that are further from zero delay requires high spectral resolution. The example in Figure 1-3 shows a 2.2 mm imaging range for SD-OCT compared with a 2.9 mm imaging range for SS-OCT. The exact imaging ranges in commercial OCT instruments depend on details of design. The combination of long imaging range and reduced sensitivity roll-off within the imaging range makes SS-OCT suitable for wide-field and long-range imaging.6,7 Recently, SS-OCT was demonstrated with an extremely long imaging range of 50 mm, which could be used to span the entire eye length.7
Finally, SS-OCT instruments typically operate with longer wavelengths of light than SD-OCT. The penetration of 1050 nm wavelength is better than 840 nm because attenuation from ocular opacity and scattering is less.8,9 This improves visibility of large vessels in the choroid as well as the ability to image below edema, choroidal neovascular membranes, or drusen.
Figure 1-2. Wavelength (frequency) swept light is split by a beam splitter into reference and sample beams. A fixed reference mirror establishes a zero delay. Backscattered light from the sample and reference paths interfere at the beam splitter. The interference spectrum is directly detected by a photodetector.
Figure 1-3. Cross-sectional OCT images of a normal retina acquired using an 840 nm SD-OCT and a 1050 nm SS-OCT system.5 SS-OCT has less sensitivity roll-off with depth than SD-OCT. (Reprinted with permission from Potsaid B, Baumann B, Huang D, et al. Ultrahigh speed 1050 nm swept source/Fourier domain OCT retinal and anterior segment imaging. Optics Express. 2010;18(19):20029-20048.)
COMMERCIAL OPTICAL COHERENCE TOMOGRAPHY ANGIOGRAPHY INSTRUMENTS
Currently, there are several commercial instruments and software that perform OCTA: the AngioVue on the Avanti RTVue XR (Optovue Inc), the AngioPlex on the Cirrus 5000 (Carl Zeiss Meditec Inc), and the DRI OCT Triton (Topcon Medical Systems Inc). The Optovue and Zeiss systems have been approved by the Food and Drug Administration for use in the United States; other instruments are approved outside the United States. Table 1-1 lists these OCTA systems.
OPTICAL COHERENCE TOMOGRAPHY IMAGING WAVELENGTHS
The wavelength of the OCT light source determines type of imaging application and its performance. In order to achieve improved OCT imaging depth in a highly scattering tissue, a long wavelength is required. However, the choice of wavelength is limited by the absorption spectrum of water (Figure 1-4).10 OCT at 1310 nm wavelength is used for imaging the anterior eye.11 However, this wavelength is highly absorbed by the vitreous and cannot be used for retinal imaging.
OCT retinal imaging is performed at wavelengths where the water absorption is low. Most commercial instruments are SD-OCT systems that operate at 840 nm wavelengths. This wavelength offers several benefits for commercial use. Broadband light sources are available that can achieve fine axial image resolution and are relatively low cost. Line scan cameras, either charge-coupled devices (CCDs) or complementary metal-oxide semiconductor (CMOS) cameras using silicon semiconductor technology, are also relatively low cost and can detect these wavelengths.
OCT retinal imaging can also be performed at 1050 nm wavelengths, where there is an absorption window in water.9 Early studies demonstrated that these longer wavelengths were less sensitive to ocular opacities, such as cataracts, and enabled an improved imaging depth in the choroid. An SD-OCT system at 1050 nm would require a more expensive line scan InGaAs camera technology in order to detect this longer wavelength. Therefore, most 1050 nm OCT instruments are based on SS-OCT. Although swept lasers are also expensive, SS-OCT enables higher acquisition speeds than SD-OCT. Table 1-2 shows a comparison between 840 nm and 1050 nm wavelengths for retinal imaging.
Figure 1-4. Light absorption spectrum of water.10 For retinal imaging, OCT systems operate at wavelengths in the low-absorption windows around 840 nm or 1050 nm. The 1050 nm wavelength has reduced attenuation from scattering or ocular opacity. Longer wavelengths at 1300 nm are too strongly absorbed by water to enable retinal imaging but are used in the anterior eye.
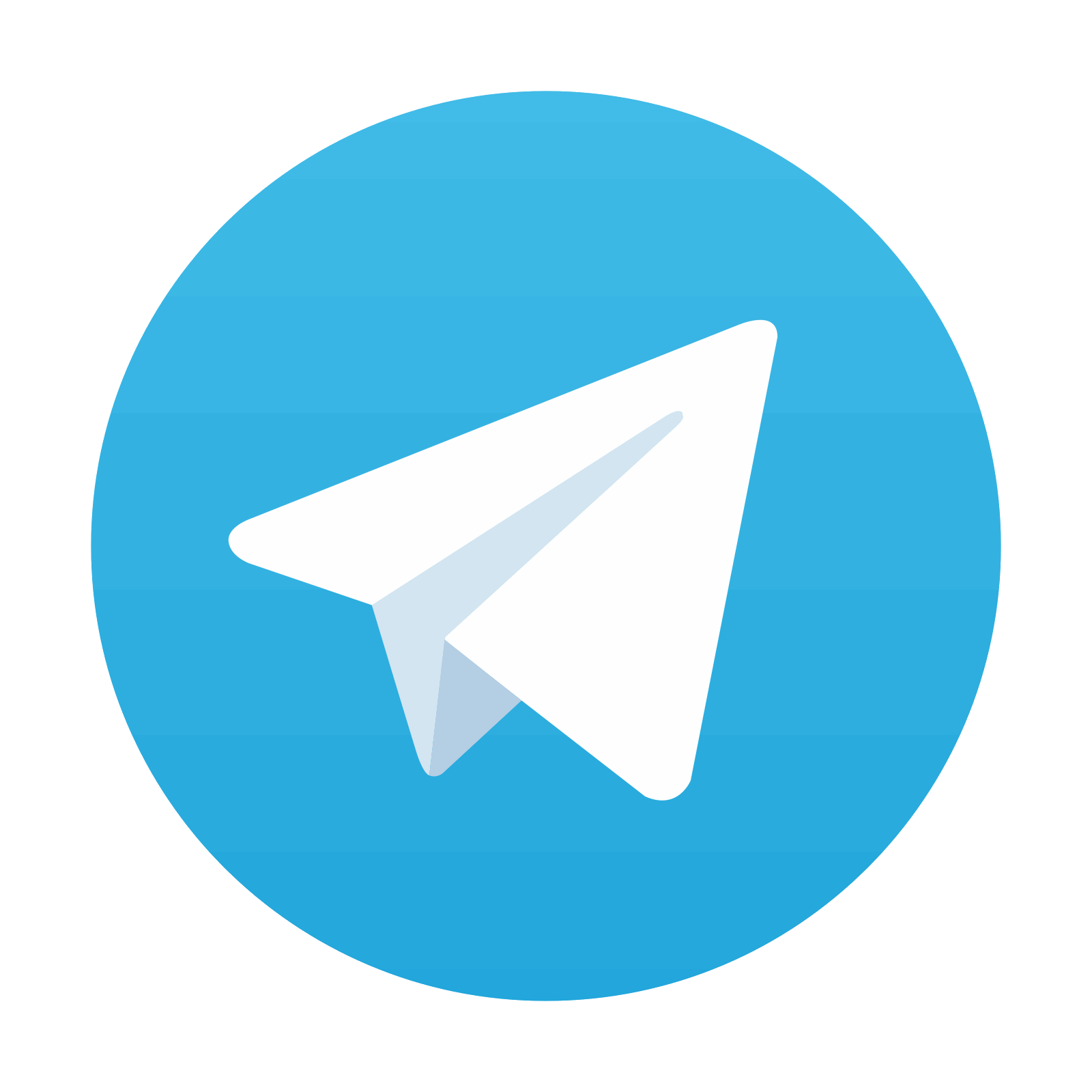
Stay updated, free articles. Join our Telegram channel

Full access? Get Clinical Tree
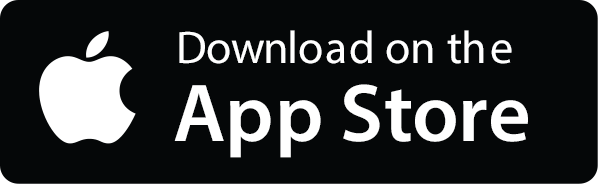
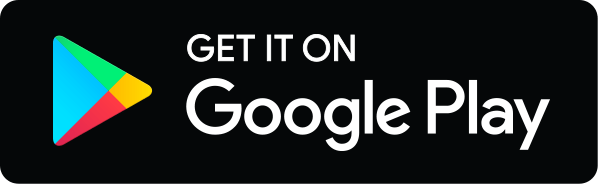