Optical Coherence Tomography Angiography
David Huang, MD, PhD and Simon S. Gao, PhD
STRUCTURAL OPTICAL COHERENCE TOMOGRAPHY
This refers to the conventional use of optical coherence tomography (OCT) systems where the OCT signal intensity representing tissue reflectance is displayed in a gray or color scale to provide structural information with micron-scale resolution.
Reflectance
Reflectance is the amount of light backscattered or reflected by the tissue sample and detected by the OCT system. Reflectance has its origin in refractive index variations within the tissue microstructure. Thus, the reflectance signal is the basis of structural OCT images. Attenuation of the OCT beam due to tissue scattering and absorption also affect the amount of light detected by the OCT system. Thus shadowing by overlying structures is always a consideration in OCT interpretation. Defocus, aberrations, polarization mismatch, and other OCT system factors that reduce beam coupling can also affect the reflectance signal.
Reflectivity
Reflectivity is a property of the tissue material and structure that determines the fraction of incident OCT beam that is backscattered. Basically, it is the reflectance after compensating for the effects of beam attenuation and coupling.
Signal Amplitude and Intensity
Because OCT measures the interference between sample and reference light, the OCT signal is proportional to the optical field (electromagnetic field) amplitude reflected from the sample and channeled into the sample arm optical fiber. Intensity is related to the optical power reflected from the sample, and is obtained by squaring the amplitude. Both signal amplitude and intensity measure the reflectance of anatomic structures. For example, the retinal pigment epithelium (RPE) is more reflective than surrounding tissues. Thus, it produces a strong OCT signal and appears brighter than adjacent layers on structural OCT images.
Signal Strength
Signal strength refers to the overall reflectance signal level in an OCT image. Assuming that tissue structure has similar reflectivity from eye to eye, the signal strength is primarily determined by the quality of OCT beam coupling (ie, defocus/aberrations, polarization mismatching, and other losses in the OCT system and ocular media).
OPTICAL COHERENCE TOMOGRAPHY ANGIOGRAPHY
Optical coherence tomography angiography (OCTA) is a functional extension of OCT that allows for the visualization of vasculature in biological tissues. Originally an offshoot of Doppler OCT that detects only axial flow, more recent implementations assess variations in the OCT signal amplitude and/or phase in order to detect both transverse and axial flow.
Flow Signal
OCTA detects flow by looking for change in the OCT signal amplitude, phase, or intensity over time. Different OCTA methods employ different strategies for quantifying the change. The output is a flow signal whose value is low in static tissues and high in blood vessels. Although different OCTA methods can produce similar results and images, the flow signal values from different OCTA methods are not directly comparable.
Amplitude/Magnitude/Intensity/Speckle Variance/Decorrelation
These refer to OCTA methods that quantify the change in the OCT signal over time by the variance or decorrelation of signal amplitude (either magnitude only, or complex amplitude that includes phase), intensity, or their log transforms.1,2 Decorrelation is equal to the variance divided by the average and quantifies flow with less dependence on the average signal strength. The decorrelation value ranges from 0 (no variation) to 1 (maximum variation). A higher decorrelation value implies higher flow velocity, up to a limit.3 The slowest flow that can be detected is the sensitivity limit, while the saturation limit is the fastest flow beyond which the decorrelation value cannot increase further. A linear range lies between the sensitivity and saturation limits. Amplitude variance is also called speckle variance,2 which is an insightful name as all amplitude/phase variance/decorrelation angiography methods are based on changes in the speckle pattern caused by motion of the blood cells relative to the OCT beam.
Phase Variance
Phase variance uses changes in the phase of the OCT signal as the means of detecting flow.4,5 Phase variance relates to flow velocity in a way similar to amplitude decorrelation, with sensitivity and saturation limits.6
Optical Microangiography
Optical microangiography (OMAG) is an OCTA technique that assesses the change in both the amplitude and phase of the OCTA signal in detecting flow.7
Split-Spectrum Amplitude-Decorrelation Angiography
Split-spectrum amplitude-decorrelation angiography (SSADA) is an efficient algorithm that improves the signal-to-noise ratio of flow detection. This is achieved by splitting the OCT spectrum, which increases the number of usable image frames for averaging and reduces noise from axial bulk motion.8 The signal gain is based on the principle that speckle variation is different for each spectral split, and contains independent flow information.
Variable Interscan Time Analysis
Variable interscan time analysis (VISTA) is a method to vary the velocity sensitivity and saturation limits (or dynamic range) of OCTA by adjusting the time interval between B-scans used for angiographic (decorrelation or variance) analysis.9 Increasing the time between B-scans used for analysis improves the sensitivity to low velocity flow but also decreases the saturation limit.
AngioPlex
AngioPlex is the OCTA software developed by Zeiss Medical Technology. It has been incorporated into the Cirrus spectral domain OCT system and uses the OMAG algorithm to detect flow. AngioPlex takes advantage of the FastTrac eye tracking system in the Cirrus to reduce motion error.
AngioVue
AngioVue is the software developed by Optovue Inc to perform OCTA on the RTVue-XR Avanti spectral OCT system. It combines the SSADA algorithm to detect flow and DuoTrac orthogonal registration and infrared video tracking technology to reduce motion error. AngioAnalytics is an extension of AngioVue that incorporates measurement tools to quantify OCTA data.
ANALYSIS OF OPTICAL COHERENCE TOMOGRAPHY ANGIOGRAPHY
En Face Projection
Volumetric OCTA scans are most often visualized en face (front view of the retina akin to a fundus photograph). En face images are generated by projection, which produces 2-dimensional views of segmented tissue layers called “slabs.” En face structural OCT images are typically generated by average-reflectance projection, in which the reflectance signal (usually on a logarithmic scale) is averaged over the thickness of the slab. OCT angiograms are usually generated by maximum flow projection, in which the highest flow signal value over the axial range of the slab is used. Average projection can also be used for flow signal in uniformly vascular layers such as the choriocapillaris.
Segmentation
Automated segmentation of key anatomic layers is required to define the slabs used for en face display of both OCTA and structural OCT. Segmentation is performed on the structural OCT images. The inner limiting membrane (ILM), outer boundary of the nerve fiber layer (NFL), outer boundary of the inner plexiform layer (IPL), outer boundary of the outer plexiform layer (OPL), RPE, and Bruch’s membrane (BM) are some of the important boundaries used to define retinal and choroidal slabs.
Automated segmentation algorithms work well in healthy eyes, but can have trouble when pathology distorts the retinal anatomy. In such cases, manual correction may be required.
Slabs and Slices
These refer to the tissue volume used to project part of a 3-dimensional volume into a 2-dimensional image. Slabs refer to thick tissue sections such as the inner retina or outer retina, whereas slices refer to thin sections of one to a few voxels thick used to examine flat thin structures, as the outer limiting membrane or the inner-outer segment junction. Generally, slabs and slices refer to en face sections that follow segmented retinal layers. However, cross-sectional slabs and slices along x-z and y-z planes are also possible and useful.
En face slabs of the retina are most usefully defined relative to anatomic boundaries. Commercial OCT systems currently split the inner retinal circulation into 2 plexuses, superficial and deep, when displaying en face angiograms. The boundary separating the plexuses is typically at the outer boundary of the IPL. This is problematic given that the outer boundary of the IPL is actually the lamellar plane where the intermediate capillary plexus (ICP) (see the complexes listed next) is located. Both histology and projection-resolved OCTA show there are 4 retinal plexuses. We recommend organizing these into 2 complexes as below:
- Superficial vascular complex.
a. Radial peripapillary capillary plexus (RPCP): all of the NFL.
b. Superficial vascular plexus (SVP): inner 80% of the ganglion cell complex (GCC, between ILM and the outer boundary of IPL), excluding the RPCP.
- Deep vascular complex.
a. ICP: outer 20% of GCC and inner 50% of the inner nuclear layer (INL).
b. Deep capillary plexus: outer 50% of INL and all of the OPL.
Bulk Motion Artifacts/Line Artifacts
These terms refer to artifactual flow signal generated by bulk motion. They appear as bright lines on en face OCTA in the fast scan direction. The brightest lines are typically caused by microsaccadic eye movements. The artifactual flow signal can be reduced by subtracting the median flow signal or other histogram parameters from each B-frame. However, residual bulk motion artifacts remain in highly backscattering structures such as the RPE.
Flow Projection Artifacts
Flow projection artifacts are the result of flickering shadows cast by flowing blood in superficial vasculature that cause variation of the OCT signal in deeper layers. This reflectance variation will be detected as a flow signal by OCTA algorithms. The artifact is most noticeable in deep reflective structures such as the RPE and can be recognized by the replication of a superficial vascular pattern on the en face angiogram of a deeper layer.
Flow Index
The flow index is calculated as the average flow signal value in the region of interest on an en face OCTA. Since the flow signal is proportional to flow velocity in small vessels (capillaries) but saturates (reaches an upper limit) in large vessels, the flow index increases with blood flow, but not in a simple linear fashion. The flow index is a more sensitive indicator of physiologic state or metabolic rate. However, it is not as reproducible as vessel density parameter (following) as a measure of vascular structures.
Vessel Area Density
The vessel area density is calculated as the percentage of area occupied by vessels in the selected region. Vessel area density is calculated from the binarized en face OCTA where each pixel is assigned a value of 1 or 0 based on whether the flow signal is above a threshold that differentiates flow from static pixels. Some authors use the term flow area density or flow density instead of vessel density. We do not recommend that as the word flow should be reserved for parameters having to do with volumetric flow rate, and not parameters that simply indicate the presence of vessels without quantifying the flow within them.
Vessel Length Density
Vessel length density is the length of the vascular network divided by the area of interest in units of mm–1. It is calculated from skeletonized en face OCTA.
Vessel Density Map
The vessel density map is an output from the AngioAnalytics software.10 It is created by determining the vessel density within small regions of the angiogram and mapping the vessel density values to a color scale. The final output is a semitransparent, color-coded map that is overlaid on top of the original angiogram.
Avascular Area/Nonflow Area
Avascular area is a significant area (larger than the normal gap between capillaries) devoid of flow pixels on an en face angiogram. On a retinal OCT angiogram of the macula, the foveal avascular zone (FAZ) produces a normal avascular area. On some OCTA systems it is also referred to as nonflow area.
Nonperfusion Area/Capillary Dropout Area
Nonperfusion or capillary dropout area refers to an avascular area that should normally be vascular. For example, on an OCT angiogram of the macula, any retinal avascular area outside the FAZ is considered a retinal nonperfusion (capillary dropout) area.
Flow Impairment
A region suffers from flow impairment if it has low or no flow signal, but the flow signal increases if the interscan time is increased via VISTA. Increasing the interscan time improves the sensitivity of OCTA and lowers the saturation limit to allow for the detection of slower flow. This allows for the separation of regions that truly have lost vasculature from those regions with vasculature with reduced flow velocity.
Quantification of Neovascularization
A number of metrics can be used to describe and quantify neovascularization. In proliferative diabetic retinopathy, abnormal vessel growth extends into the pre-retinal vitreous above the ILM. In age-related macular degeneration, neovascularization occurs in the outer retina (the region between the outer boundary of the OPL and BM). On the en face angiogram, the lesion or membrane area is a metric to quantify the area occupied by the neovascular complex. The neovascular vessel area is the area of the pathologic neovascular net within the membrane area, not including the space between vessels. Following skeletonization of the vessel area, vessel length can be computed as the length of the skeletonized neovascular net. Vessel area density and vessel length density can be determined by dividing the vessel area or vessel length by the membrane area. Some authors use the term flow area instead of vessel area. We do not recommend this practice as the word flow should be reserved for parameters having to do with volumetric flow rate, and not parameters that simply indicate the presence of vessels without quantifying the flow within them.
REFERENCES
1. Barton J, Stromski S. Flow measurement without phase information in optical coherence tomography images. Opt Express. 2005;13(14):5234-5239.
2. Mariampillai A, Standish BA, Moriyama EH, et al. Speckle variance detection of microvasculature using swept-source optical coherence tomography. Opt Lett. 2008;33(13):1530-1532.
3. Tokayer J, Jia Y, Dhalla AH, Huang D. Blood flow velocity quantification using split-spectrum amplitude-decorrelation angiography with optical coherence tomography. Biomed Opt Express. 2013;4(10):1909-1924.
4. Fingler J, Zawadzki RJ, Werner JS, Schwartz D, Fraser SE. Volumetric microvascular imaging of human retina using optical coherence tomography with a novel motion contrast technique. Opt Express. 2009;17(24):22190-22200.
5. Makita S, Hong Y, Yamanari M, Yatagai T, Yasuno Y. Optical coherence angiography. Opt Express. 2006;14(17):7821-7840.
6. Liu G, Lin AJ, Tromberg BJ, Chen Z. A comparison of Doppler optical coherence tomography methods. Biomed Opt Express. 2012;3(10):2669-2680.
7. An L, Wang RK. In vivo volumetric imaging of vascular perfusion within human retina and choroids with optical micro-angiography. Opt Express. 2008;16(15):11438-11452.
8. Jia Y, Tan O, Tokayer J, et al. Split-spectrum amplitude-decorrelation angiography with optical coherence tomography. Opt Express. 2012;20(4):4710-4725.
9. Choi W, Moult EM, Waheed NK, et al. Ultrahigh-speed, swept-source optical coherence tomography angiography in nonexudative age-related macular degeneration with geographic atrophy. Ophthalmology. 2015;122(12):2532-2544.
10. Agemy SA, Scripsema NK, Shah CM, et al. Retinal vascular perfusion density mapping using optical coherence tomography angiography in normals and diabetic retinopathy patients. Retina. 2015;35(11):2353-2363.
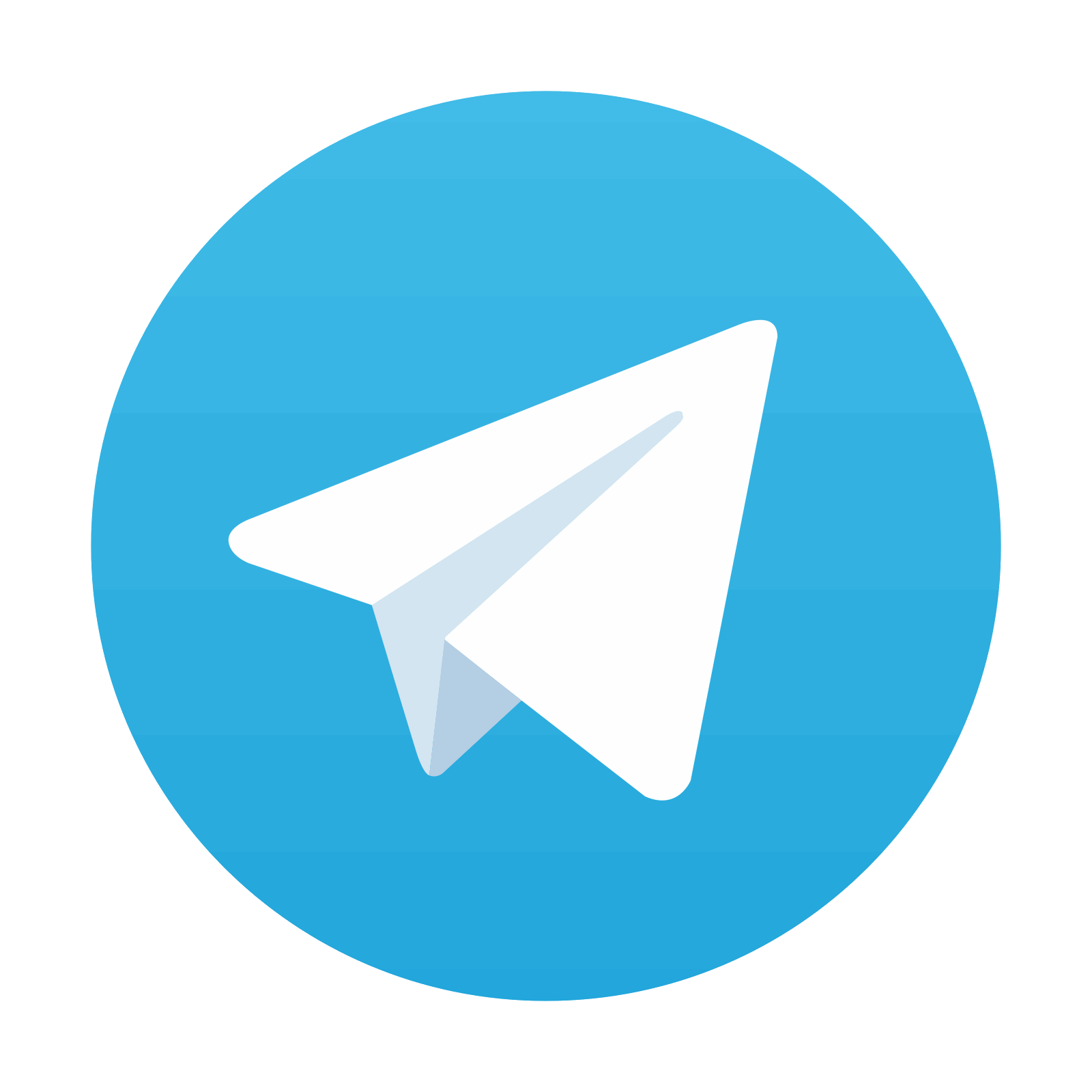
Stay updated, free articles. Join our Telegram channel

Full access? Get Clinical Tree
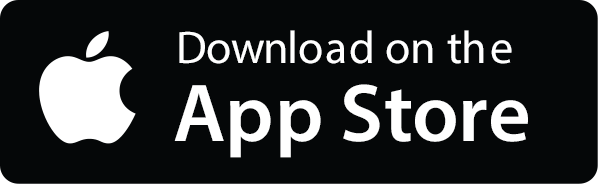
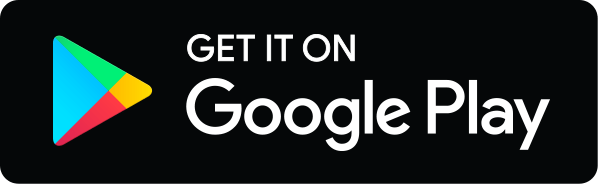