Tony Ko, PhD and Jay Wei, MS
INTRODUCTION TO ANGIOVUE OPTICAL COHERENCE TOMOGRAPHY ANGIOGRAPHY
Available since 2014, Optovue’s AngioVue system is the world’s first commercially available optical coherence tomography angiography (OCTA) system. Between 2015 and 2017, more than 260 journal papers on OCTA have been published using data from the AngioVue system. The AngioVue system is based on Optovue’s Avanti Widefield optical coherence tomography (OCT) platform, which performs OCT scanning at 70,000 axial scans (A-scans)/second and eye-motion tracking at 30 frames/second using the infrared fundus video images. The Avanti along with the AngioVue OCTA software can be used to visualize the microvasculature of the eye in a noninvasive manner. This chapter describes the core technologies behind the AngioVue system as well as its current acquisition, display, and analysis features.
ANGIOVUE TECHNOLOGIES
The core technologies used in the AngioVue system are split-spectrum amplitude-decorrelation angiography (SSADA)1 for OCT angiography image formation and DualTrac Motion Correction (Optovue Inc) for dealing with patient eye motion during OCTA data acquisition. In addition, standard OCT technologies such as segmentation of different retinal layer boundaries are used to provide depth-resolved en face visualization of both OCT intensity information (en face OCT) and OCTA microvasculature information (OCT angiograms).
OCTA algorithms compare changes in the back-reflected OCT signal between repeated cross-sectional B-scans in order to detect motion in the blood vessel. The SSADA algorithm is an amplitude-based method (ie, detecting amplitude changes in the returning OCT signal) with additional processing to further enhance motion contrast for flow detection and reduce noise arising from axial motion.1 The SSADA algorithm splits the OCT image into different spectral bands, thus producing multiple frames from a single OCT B-scan. Since the information content in each frame is different from each other, the split spectrum approach can increase the number of frames used, which leads to an improved motion contrast signal. Another advantage of splitting the spectrum is that each split frame has a lower axial resolution and is less susceptible to axial eye motion. Spectral domain (SD)-OCT axial resolutions typically range from 3 μm to 5 μm. Since OCTA algorithms are designed to detect any motion between repeated B-scans, small axial eye motion on the scale of a few microns will create a noisy background in the OCTA image. The typical approach to reduce background noise in the motion contrast image is to increase the number of repeated B-scans used to form a single OCTA image in order to use averaging to reduce the noise. By splitting the spectrum to lower the axial resolution, the SSADA algorithm can reduce the background noise from small axial motion without needing to increase the number of repeated B-scans. In AngioVue, the SSADA algorithm permits the reduction of repeated B-scans to the minimum number of 2 while still generating high-quality OCT angiograms, thereby reducing the total acquisition time of each OCTA volume to less than 3 seconds.2 Figure 8-1 demonstrates the concept of the SSADA algorithm as implemented in AngioVue.
Figure 8-1. SSADA algorithm working on AngioVue. (A) Two B-scans are repeated to calculate the OCTA information. (B) From the repeated B-scans, SSADA can generate 11 sets of OCTA calculation through splitting the spectrum. The resulting 11 images are then averaged to form the final OCTA B-scan. (C) Acquiring multiple OCTA B-scans in this manner leads to the generation of the 3-dimensional OCT angiogram.
Since OCTA algorithms are highly sensitive to any motion between repeated B-scans, patient eye motions during the data acquisition of an OCTA volume can lead to substantial motion artifacts in the OCTA data. The artifacts associated with saccades usually show up as straight bright lines in the OCT angiogram that can obscure useful clinical information. Optovue’s DualTrac Motion Correction enables high quality imaging by employing 2 levels of motion artifact removal. The first level, eye tracking, performs real-time OCT scan correction to account for eye blinks, saccades, and fixation drifts. The second level, motion correction technology (MCT), is a post-processing step that performs precise pixel-level registration in 3 dimensions to further improve the motion correction accuracy and the resulting image quality.
The real-time tracking system uses a high frame rate infrared fundus video to detect any blinks or fixation changes from the patient’s eye. If a blink or fixation change is detected, the tracking system monitors for the completion of the offending motion. When the eye has stopped moving, the OCT scan beam is steered to a new position to compensate for the detected eye motion. The data acquisition is then restarted from the point of the 3-dimensional (3D) volume before the start of the offending motion in order to reacquire the portion of the 3D volume that had been corrupted by motion artifacts. This real-time tracking can ensure the acquisition of OCTA volume free of frank eye motion or blink artifacts. However, real-time tracking systems almost always have tracking accuracies worse than the 10 μm to 15 μm transverse or sampling resolution used in most OCTA systems. The resolution mismatch between the tracking and OCTA systems can lead to “tracked” OCT angiograms in which some vessels appear to have small wiggles (ie, increased tortuosity) due to the lack of high-precision real-time eye tracking at the level of a few microns.
Optovue’s DualTrac Motion Correction employs a second-level correction called MCT to further improve on the performance of the real-time tracking system in removing motion artifacts. MCT is an orthogonal registration algorithm that uses information from 2 OCT image volumes in order to align the A-scans in their original anatomical position in the 3D (X, Y, and Z) space.3 The MCT algorithm requires the acquisition of 2 OCTA imaging volumes that are orthogonal in scan direction to each other. In AngioVue, the first volume is a horizontal raster (Fast-X) scan while the second volume is a vertical raster (Fast-Y) scan. The MCT algorithm combines the complementary information contained in the 2 orthogonal volumes to allow for the best registration of each A-scan in the 3D space. By combining the information from 2 volumes, MCT effectively doubles the amount of B-scans available for averaging, which also results in much better OCT and OCTA image quality.4 As shown in Figure 8-2, the MCT algorithm can be used following the completion of data acquisition to correct out any residual motion artifacts that real-time tracking was unable to remove. As shown in Figure 8-3, in cases where real-time tracking cannot be performed, the MCT algorithm can still be used to remove motion artifacts independent of having a tracked data volume.
Figure 8-2. MCT can correct residual motion artifacts after real-time tracking is applied. (A) An OCT angiogram acquired with real-time tracking. The patient was asked to perform a series of quick fixation changes and blinks during the data acquisition. Two small areas (red arrows) of vessel misalignment indicate the limits of tracking precision. (B) Following MCT, the residual motion artifacts and vessel misalignments are minimized, and the image quality is also much improved.
Figure 8-3. MCT can be applied even in situations where real-time tracking cannot be achieved. (A) A Fast-X OCT angiogram showing the bright and horizontal motion artifacts associated with saccades and fixation shifts. (B) A Fast-Y OCT angiogram showing the motion artifacts in the vertical direction. (C) The combined result following MCT correction showing minimal motion artifacts from the saccades of the original Fast-X and Fast-Y volumes.
ACQUIRING ANGIOVUE SCANS
Acquiring an AngioVue scan is as simple as acquiring a 3D OCT volume. Each AngioVue B-scan contains 304 A-scans with each scan location repeated at least once in order to perform OCTA. The time interval between repeated B-scans in AngioVue is approximately 5 ms, which is a sufficiently long time difference to detect the slow flows in the capillaries. The AngioVue scan volume is composed of 304 B-scans with the resulting OCT angiogram containing 304 × 304 pixels. Using the SSADA algorithm, the repeated B-scans at each scan location are reduced to the minimum number of 2 while still generating high-quality OCT angiograms. Including the flyback time to return the scan beam to the start of each B-scan, a total of approximately 209,000 A-scans are acquired in one AngioVue volume. The acquisition time for each imaging volume is therefore slightly less than 3 seconds at 70,000 A-scans/second.
AngioVue scans can be acquired in the macula region using the fovea fixation (Angio Retina) or the optic disc region using the disc fixation (Angio Disc). The scan sizes allowed in the Angio Retina scan are 3 × 3 mm, 6 × 6 mm, and 8 × 8 mm, while the scan sizes allowed in the Angio Disc scan are 3 × 3 mm and 4.5 × 4.5 mm. Currently, all scan sizes are acquired with 304 × 304 A-scans/volume. The smaller scan sizes will produce the highest-quality OCT angiograms while larger scan sizes allow wider area coverage to aid multimodality comparisons. By default, all OCTA scans use the Vitreoretinal (ie, non-Enhanced Depth Imaging) acquisition mode to place emphasis on the retinal vessels. Although it is not recommended, Chorioretinal (ie, Enhanced Depth Imaging) acquisition mode is possible and can be manually selected during the data acquisition step.
Since the real-time tracking system employs the infrared fundus video for motion tracking and correction, it is essential to obtain a high-quality infrared fundus image to ensure good tracking performance. The operator should optimize the distance between the AngioVue scanner and the front of the cornea (ie, the working distance) so the surface back-reflections from the cornea and lens are not present in the infrared fundus image. Optimal alignment to the center of the pupil is also desired to reduce any vignetting artifacts in the fundus image. After a high-quality infrared fundus image is obtained, the OCT image can then be optimized through the auto-optimization process. The automated process first brings the OCT image into the scanning window (Auto Z), then adjusts the optics in the scanner for the best focus of the OCT beam (Auto F), and finally adjusts the polarization states of the OCT beam to maximize the returning OCT signal (Auto P).
Figure 8-4. Flow projection artifact suppression in AngioVue. (A) An AngioVue image at the level of the superficial capillary layer. (B) The same image at the level of the outer retinal zone demonstrating the projection of inner retinal vessels onto the RPE layer. (C) Removal of the flow projection artifacts enhances the visualization of neovascular pathology in the outer retinal zone.
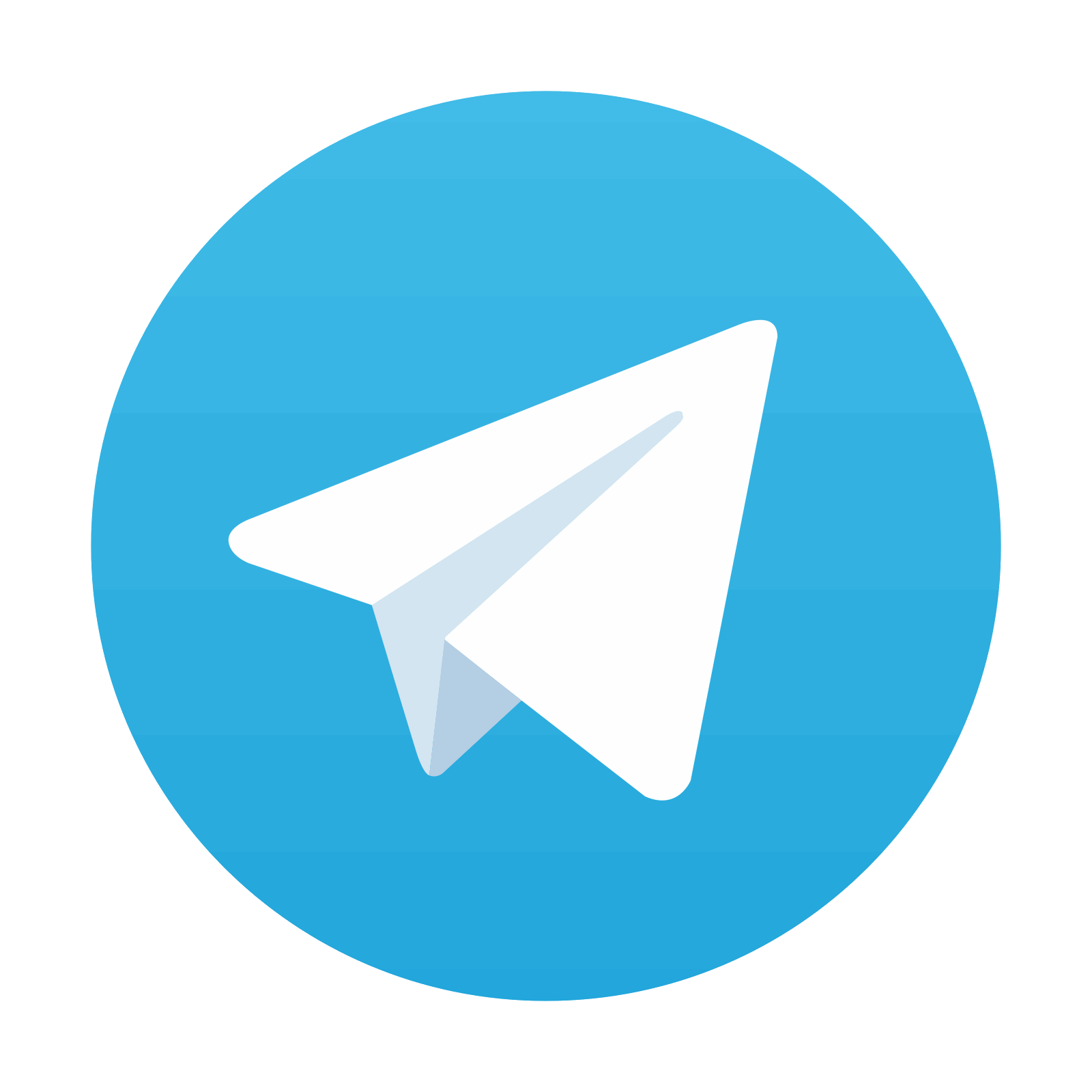
Stay updated, free articles. Join our Telegram channel

Full access? Get Clinical Tree
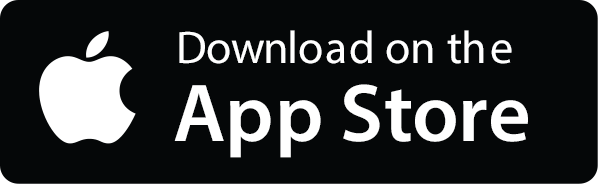
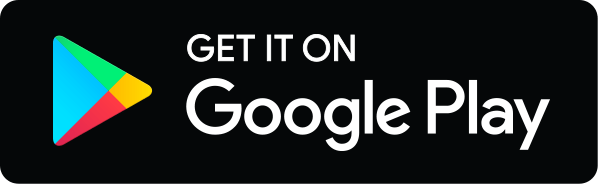