4.1 Diagnostic/Technology Overview
Optical coherence tomography angiography (OCTA) has recently emerged as a noninvasive technique for imaging the vasculature of the eye. OCTA relies on the rapid acquisition of multiple OCT scans at the same location on the retina. Because all of the structures in the back of the eye, except for the blood flowing through the vessels, are static, the only feature changing between the sequential scans would be blood flow. Utilizing unique computer algorithms to look for the difference between sequential, rapidly acquired B-scans (a process called decorrelation) results in a retinal vasculature map. Because the retina is transparent and OCT is a 3D imaging modality, point by point decorrelation can be applied in three dimensions, generating a depth-resolved map of the retinal vasculature. Depending on the penetration of the OCT beam past the retinal pigment epithelium, a map of the choriocapillaris may also be generated.
Two technologic advancements have been critical to the evolution of OCTA. The first is high scanning speeds to minimize interscan movement to enable decorrelation. The second is a resolution high enough to be able to visualize motion within the smaller blood vessels. Both of these conditions were met with the advent of spectral domain OCT (SD-OCT) machines and are also present in the next generation of swept source OCT (SS-OCT).
Moreover, since the angiographic data are acquired by decorrelation of structural OCT data, the OCTA comes coregistered with the structural OCT data. This allows for the overlay of the angiographic data with the simultaneously acquired structural OCT data, creating a volumetric cube that can be scrolled through or segmented into layers by automated or manual segmentation algorithms. Angiographic data is generated by analyzing changes in amplitude and/or phase of vascular flow, created by the movement of erythrocytes, which produce a decorrelation signal between consecutive scans. Numerous different algorithms can be used to do this: amplitude decorrelation, such as in the Split-Spectrum Amplitude-Decorrelation Angiography, or a combination of amplitude and phase based algorithms, such as OCT-based microangiography, which are used in commercially available OCTA devices.
There are two major ways of analyzing OCTA data. Volumetric data consisting of structural and angiographic images can be scrolled through to assess pathological changes at various retinal layers. While this method highlights pathology at various levels, it is often more time consuming and difficult to analyze. The other approach is segmentation of an area of interest to generate an en face image. Various automated algorithms have been employed to create segmentation slabs. However, accurate segmentation is always a challenge, especially in eyes with pathology where boundaries between layers may be difficult to delineate. Manual segmentation, although more time consuming, allows for changes in the slab thickness and repositioning of the segmentation lines for more accurate visualization and analysis of pathological lesions. Currently, most OCTA machines will present segmented data in two ways: an en face angiographic image that delineates the lesion, and a structural B-scan with flow overlay that allows for structure–vasculature association (▶ Fig. 4.1).
Fig. 4.1 (a) A 3 × 3 mm spectral domain optical coherence tomography angiography (SD-OCTA) scan centered at the fovea showing areas of nonperfusion, pruning of vessels (arrow), microaneurysms, and retinal neovascularization. (b) Extraretinal extension of the membrane on the corresponding coregistered B-scan (arrow) confirmed proliferative diabetic retinopathy. Note the presence of motion artifact in the lower portion of the en face image. (c) A swept source OCTA 12 × 12 mm scan centered at the parafoveal right lower quadrant showing areas of nonperfusion and retinal neovascularization. (d) The corresponding coregistered B-scan confirms proliferative diabetic retinopathy (arrow). Motion artifacts, while present in both, are more discernable in the SD-OCTA image.
4.1.1 Artifacts
A major challenge of OCTA is its susceptibility to degradation by artifacts, predominantly projection and motion artifacts. Projection artifacts are created by the scattering of light by overlying blood vessels and subsequent reflection by the underlying layers, especially the highly reflective retinal pigment epithelium (RPE). This allows for the more superficial vessels to also be visualized in the deeper layers, hindering accurate analysis of the deeper layers. Projection artifact correction algorithms have been employed in commercial device software. While they do not completely remove projection artifacts, they are being improved upon.
Microsaccades, as well as patient and eye movement, may occur during image acquisition. These extraneous movements create motion artifacts that appear as white lines on an OCTA image. Nonvascular movement of reflective structures then generates a false decorrelation signal and gives the appearance of blood flow. Most devices employ some form of motion correction (software-based and/or eye-tracking-based). Motion-tracking software has significantly enhanced the quality of OCTA images but also increases scan time in patients with poor fixation.
Currently available OCTA scans have a field of view that is limited because of constraints on acquisition time; the eye can only hold still for so long. Thus, an increase in scanning area is likely to increase imaging time and compromise resolution. Since the principle application of OCTA is currently in macular and parafoveal pathologies, the 3 × 3 mm scan pattern helps visualize these areas with high resolution. However, 6 × 6 scans are also available on most SD-OCTA machines, and with higher speed SS-OCTA instruments larger scans can be visualized in a single acquisition.
4.2 Key Applications
Dye-based imaging modalities, such as fluorescein angiography (FA) and indocyanine green angiography (ICGA), have been the gold standard for the diagnosis of retinal and choroidal vascular pathologies. FA and ICGA offer certain advantages over OCTA, such as the ability to image a wider field, visualization of vessel leakage, and improved detection of aneurysms with slow flow. However, they also have disadvantages, including their invasive nature, which precludes frequent acquisition to follow pathology, and the fact that the angiographic scan does not come correlated to any structural scans, and therefore only approximate visual registration can be performed to compare structure (as seen on color photographs and OCT) to vasculature (as seen on the angiogram). Moreover, FA better visualizes larger and more superficial vessels. It has been shown not to visualize in any great detail the intermediate and deep capillary plexus of the retina. OCTA, on the other hand, is noninvasive, has minimal to no risk of complications, a shorter image acquisition time, and is independent of the circulation of dye. The 3D nature of the scan allows for independent visualization of the deeper retinal vasculature and the choriocapillaris. The resolution of vasculature seen on OCTA has been shown nearing the level of histological studies. Due to its noninvasive nature, discernible microvascular changes of the retina can be followed longitudinally by OCTA as often as clinically mandated.
4.2.1 Diabetic Retinopathy
OCTA is useful in identifying the characteristic lesions of diabetic retinopathy (e.g., capillary nonperfusion, retinal ischemia, neovascularization, and microaneurysms) and can be used to follow them longitudinally (▶ Fig. 4.2). Microvascular flow alterations such as capillary pruning, intraretinal microvascular abnormalities, and venous beading and looping may be detected at earlier stages with OCTA as compared to conventional clinical examination (▶ Fig. 4.1). Quantitative vessel density measurements may improve the staging of diabetic retinopathy.
Fig. 4.2 Swept source optical coherence tomography angiography 3 × 3 mm scan of an eye with severe nonproliferative diabetic retinopathy. Visible are prominent venous looping (arrow), venous beading (arrow), and areas of capillary nonperfusion.
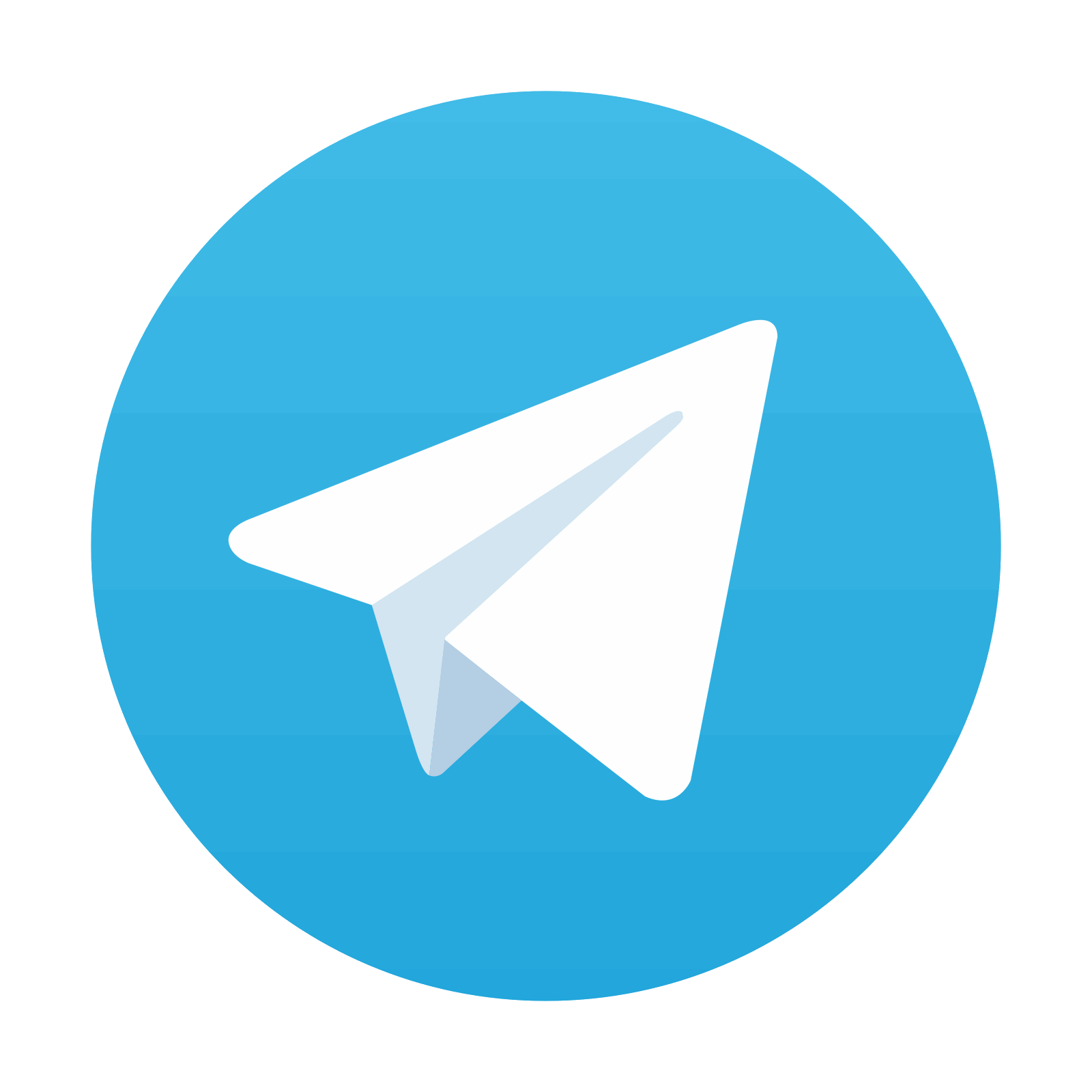
Stay updated, free articles. Join our Telegram channel

Full access? Get Clinical Tree
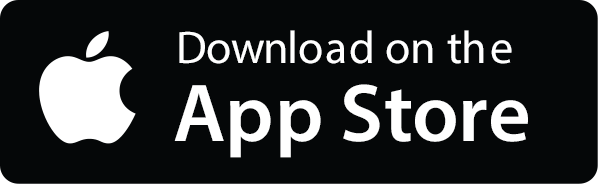
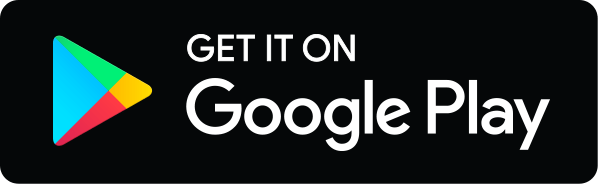