ANATOMY OF THE OPTIC NERVE
Intraocular course
Intraorbital course
Intracanalicular course
Intracranial course
CLINICAL EXPRESSION OF DISEASE
Pallor and cupping
Optic disc swelling (edema)
Accompanying signs
ISCHEMIC OPTIC NEUROPATHIES
Nonarteritic anterior ischemic optic neuropathy
Arteritic anterior ischemic optic neuropathy
Diabetic papillopathy
Papillophlebitis
Radiation optic neuropathy
OPTIC NEURITIS
OPTIC PERINEURITIS
PAPILLEDEMA
Mechanism of papilledema
Idiopathic intracranial hypertension (pseudotumor cerebri)
Other causes of papilledema
COMPRESSIVE OPTIC NEUROPATHIES
Optic nerve sheath meningiomas
Other causes of optic nerve compression
INTRINSIC NEOPLASMS
Optic nerve glioma
Lymphoproliferative disorders
Other intrinsic neoplasms
INFLAMMATORY OPTIC NEUROPATHIES
INFECTIOUS OPTIC NEUROPATHIES
Optic disc edema with a macular star
Cat-scratch neuroretinitis
Other infectious neuropathies
TOXIC AND NUTRITIONAL OPTIC NEUROPATHIES
HEREDITARY OPTIC NEUROPATHIES
Autosomal dominant (Kjer) optic atrophy
Leber hereditary optic neuropathy
TRAUMATIC OPTIC NEUROPATHY
GLAUCOMA
OPTIC DISC DRUSEN
ANOMALOUS OPTIC DISCS
Crowded optic discs
Elevated discs without drusen
Tilted optic discs
Myelinated retinal nerve fibers
Hypoplasia
Aplasia
Coloboma
KEY POINTS
In Chapter 3, the organization of axons in the sensory visual system provided the basis for understanding the patterns of visual field defects. In this chapter, the neuroanatomy of the anterior sensory visual system forms the foundation for understanding optic nerve disorders and their clinical expression.
The optic nerve originates at the confluence of retinal ganglion cell axons as they traverse the scleral canal to exit the globe, and ends anatomically as these axons merge with the axons of the fellow optic nerve at the chiasm. Anatomic divisions of the optic nerve include intraocular, intraorbital, intracanalicular, and intracranial portions (Figure 4–1).
The short intraocular course of the optic nerve is often referred to as the optic nerve head, and the portion that can be seen with the ophthalmoscope is called the optic disc. The optic disc is usually oval, measuring about 1.5 by 1.75 mm, with its long axis typically oriented vertically. In most subjects, the optic cup, devoid of axons, is seen centrally, surrounded by the pink, doughnut-shaped neuroretinal rim (Figure 4–2A). The rim consists of axons seen end-on, as they pass from the nerve fiber layer and make a right-angled turn into the scleral canal. Although the number of axons in normal subjects is relatively constant, the diameter of the scleral canal varies among individuals. When the scleral opening is small, the axons are crowded into a small space (Figure 4–2B). These small, cupless discs are often referred to as “discs at risk,” as they are frequently associated with optic disc infarction (anterior ischemic optic neuropathy [AION]). Individuals with large scleral openings may have large disks with large central cups, which may be mistaken for the pathological cupping characteristic of glaucoma.
Figure 4–2.
Ophthalmoscopic Appearance of the Optic Disc.
(A) The normal left optic disc has a cup-to-disc ratio of 0.5. Note the pink color of the neuroretinal rim surrounding the cup, the central position of the retinal artery and vein, and the branching pattern of the retinal vasculature. (B) A normal left optic disc with a small cup-to-disc ratio, less than 0.1. This optic disc configuration is associated with an increased risk of developing anterior ischemic optic neuropathy.

Optic nerve axons congregate into bundles as they pass through the lamina cribrosa. This fibrous diaphragm is contiguous with the sclera and has approximately 200 openings. The lamina cribrosa further divides the intraocular optic nerve head into prelaminar, laminar, and postlaminar portions. Optic disc swelling occurs when the prelaminar axons swell due to failure of orthograde axoplasmic flow at the level of the lamina cribrosa. Axoplasmic stasis and disc swelling can be caused by compression, ischemia, toxins, or inflammatory processes, and is not disease specific.
Behind the globe, two to six short posterior ciliary arteries (branches of the ophthalmic artery) penetrate the sclera in a circumferential fashion around the exiting optic nerve. These vessels form an incomplete anastomotic ring at the level of the choroid (arterial circle of Zinn-Haller), supplying both the high-flow vasculature of the choroidal circulation and the optic nerve head. Although the central retinal vessels pass through the optic nerve head, their contribution to its vascular supply is negligible (Figure 4–3). Insufficient blood flow through the posterior ciliary arteries from thrombosis, hypotension, vasculitic occlusion, or other disease causes ischemic optic neuropathy.
Figure 4–3.
Anterior Optic Nerve Anatomy and Blood Supply.
Observe the abundant blood supply to the retrobulbar optic nerve from the pial plexus on the surface of the nerve and some contribution from the central retinal artery. In contrast, the prelaminar and laminar portions of the optic nerve head do not receive any significant contribution from the central retinal artery, but rely almost entirely on the short posterior ciliary arteries, which also supply the high-flow choroid. (Reproduced with permission from Weinstein JM. The pupil. In Slamovits TL, Burde R, associate editors. Neuro-ophthalmology, vol 6. In Podos SM, Yanoff M, editors: Textbook of ophthalmology. St Louis, 1991, Mosby.)

There are high concentrations of mitochondria in the axons of the prelaminar and laminar regions of the optic nerve. This is thought to be due to the much higher energy requirements for conduction in this unmyelinated portion of the optic nerve. This may also explain the vulnerability of the prelaminar optic nerve to processes that affect mitochondria, including heritable, toxic, and nutritional disorders, but also possibly anterior ischemic optic neuropathy and glaucoma.
After passing through the lamina cribrosa, the retinal ganglion cell axons acquire myelin sheathing, doubling the diameter of the optic nerve to more than 3 millimeters. The myelin sheath is produced by oligodendrocytes, the same cell type found in the white matter tracts of the central nervous system. Peripheral nerves are myelinated by Schwann cells. Thus, the optic nerve is histologically a white matter tract of the brain, rather than a peripheral nerve. This is evident clinically in several ways: The optic nerve does not usually regenerate like other cranial nerves, and optic neuritis is a frequent occurrence in multiple sclerosis (MS), a disease affecting white matter in the brain and spinal cord.
The orbital portion of the optic nerve is approximately 25 mm in length from the posterior aspect of the globe to the orbital apex. Because the globe is only 15 mm anterior to the orbital apex, the optic nerve describes a gently curved path; the extra length allows full movement of the globe without being tethered by the optic nerve (see Figure 4–1). In the orbit, the optic nerve is surrounded by the optic nerve sheath, which is continuous with the intracranial dura through the optic canal posteriorly, and bounded by the sclera anteriorly. The sheath encloses an extension of the intracranial meninges, with pia, arachnoid, and cerebrospinal fluid continuous with the intracranial subarachnoid space. Elevated intracranial pressure can be transmitted through the optic canal directly to the optic nerve head, causing bilateral optic disc edema (papilledema). Meningiomas can arise within the orbit from the dural optic nerve sheath, just as they develop from the intracranial meninges.
At the orbital apex, the nerve sheath fuses with a fibrous ring (annulus of Zinn) that forms the insertion of the superior oblique and the four rectus muscles. This connection explains why eye movement can cause pain when the optic nerve is inflamed in retrobulbar optic neuritis.
The vascular supply of the intraorbital optic nerve is more robust and redundant than that of the optic nerve head. Branches of the ophthalmic artery provide numerous longitudinal pial vessels to the surface of the optic nerve, which in turn yield penetrating vessels that extend toward the center of the nerve. The central retinal artery enters the nerve approximately 10 mm behind the globe, also contributing to the vascular supply of this segment of the intraorbital optic nerve (see Figure 4–3). Given such a hardy blood supply, ischemic insults to the retrobulbar optic nerve are rare, in contrast to the relatively frequent occurrence of optic disc infarction (ie, AION).
The intracanalicular portion of the optic nerve is approximately 10 mm long, beginning where the optic nerve enters the optic foramen in the lesser wing of the sphenoid, and ending where the optic nerve exits the optic canal and enters the intracranial cavity. From the orbit, the optic canal is directed medially and superiorly to enter the intracranial cavity. The optic canal is separated from the sphenoid sinus by very thin papyraceous bone, and the course of the optic nerve can be seen as a convexity in the lateral wall of the sinus. In addition to the optic nerve, the optic canal also contains the ophthalmic artery (and some accompanying sympathetic fibers).
Space-occupying lesions within the bony confines of the optic canal (such as an intracanalicular meningioma) do not have to be large to compress the intracanalicular optic nerve and cause visual loss, and may not be easily seen on neuroimaging. Blunt accelerating or decelerating trauma to the orbital rim can transmit forces to the optic canal, causing optic nerve tears, contusion, and shearing injury to the optic nerve, with or without canal fractures. Hemorrhage or edema within this confined space may produce additional ischemic injury.
The intracranial portion of the optic nerve extends from the nerve’s entrance into the intracranial space to the chiasm. Its length is approximately 10 mm, but varies greatly between individuals (from 3 to 18 mm). The optic nerves angle superiorly at approximately 45° from the skull base and converge toward the midsagittal plane to form the chiasm. The anterior clinoid bone is superior and lateral to the optic nerve as the nerve emerges from the optic foramen. The inferior frontal lobes and olfactory tracts are located above the nerve. The vascular supply of the optic nerve in this location includes the carotid arteries, located laterally, as well as the anterior cerebral arteries and the anterior communicating arteries, located superiorly. As the carotid artery emerges from the cavernous sinus, the ophthalmic artery is its first intracranial branch, travelling on the inferior surface of the optic nerve to enter the optic foramen. Carotid-ophthalmic artery aneurysms (commonly large and bilateral) or dolichoectatic enlargement and displacement of the carotid artery can cause compression of the intracranial optic nerve.
Acquired optic nerve disorders commonly result in pallor, pathological cupping, or swelling of the optic disc. Congenital optic disc anomalies, discussed at the end of this chapter, produce a broad spectrum of optic disc appearances that can easily be confused with acquired disease.
The axons that make up the optic nerve may be affected by disease anywhere along their course, from their origin in the inner retina to their synaptic endpoint in the lateral geniculate nucleus. Fatal injury to an axon results in retrograde and orthograde degeneration of the axon, and eventual death of the retinal ganglion cell of origin. Axonal injury remote from the optic disc may cause optic nerve dysfunction without any acutely observable optic disc abnormality, but over time optic disc atrophy and nerve fiber layer dropout in the inner retina becomes visible, revealing the extent of the damage. In the majority of neuro-ophthalmic disorders, axonal loss manifests as pallor of the normally pink neuroretinal rim, without apparent loss of neuroretinal rim mass. Diffuse disc pallor is a final common pathway for many optic neuropathies, and is nondiagnostic. However, the location of segmental pallor is often instructive, such as the sectoral or altitudinal pallor in AION, unilateral “bow-tie” atrophy with optic tract lesions (bilateral with chiasmal lesions), or temporal pallor characteristic of the toxic/nutritional or hereditary optic neuropathies (see Figures 4–10D; 4–34A, C; 5–4B; 5–9B). Loss of axons may also manifest as optic nerve cupping, as the central cup enlarges from axonal dropout. Optic disc cupping is characteristic of glaucoma, but can occur in other optic neuropathies. Cupping with pallor of the remaining neuroretinal rim suggests a cause other than glaucoma (see Figure 4–39B).
The term “optic disc edema” implies that optic disc swelling is the result of extracellular fluid from vascular transudation, which is usually not the case. “Optic disc swelling” is a better term, but commonly the two terms are used interchangeably (and we succumb to the popular usage of “edema” in this book—but only occasionally).
Swelling of the prelaminar axons causes elevation and expansion of the optic nerve head. Axons swell when the normal process of anterograde axoplasmic flow is dammed up by mechanical, ischemic, or toxic-metabolic processes occurring in the mitochondria-rich region of the axons anterior to the lamina cribrosa (Figure 4–4). The normally distinct border of the optic disc and surrounding retina become blurred as swollen peripapillary axons become elevated and opaque. Transudation of fluids from injured axons and disc vessels also contribute a small amount to the disc’s swollen appearance. A number of insults to the optic nerve can result in optic disc swelling (Table 4–1). Elevated intracranial pressure transmitted to the optic nerve head within the confines of the optic nerve sheath can cause stasis of axoplasmic flow at the level of the lamina because of regional pressure differentials. Anterior ischemic optic neuropathy produces optic disc swelling as a result of ischemia. Inflammation from infection, demyelination, or scleritis can also produce disc swelling. Because optic disc swelling can be caused by many disease processes, the history, examination, ancillary testing (such as neuroimaging), and clinical course are the keys to determining the diagnosis. Important features in developing a differential diagnosis are listed in Figure 4–5, with individual disorders covered in detail in this chapter.
Figure 4–4.
Prelaminar swelling in optic disc edema.
(A) Cross section of optic disc through normal optic nerve. (B) Optic disc with papilledema. Observe the swelling anterior to the lamina cribrosa from axoplasmic stasis in papilledema. (Reproduced with permission from Liu GT, Volpe NJ, Galetta SL: Neuro-ophthalmology: Diagnosis and Management, Book with DVD-ROM: 2nd edition. Saunders/Elsevier, 2010.)

CAUSES OF OPTIC DISC ELEVATION
|
Figure 4–5.
Common causes of optic disc swelling.
Although there are many causes of optic disc swelling, a thorough history and examination helps to narrow the possibilities. The more common diagnostic possibilities can often be distinguished by whether one or both optic discs are involved, the age of the patient, and the degree and time course of visual loss. Abbreviations: AION, anterior ischemic optic neuropathy; GCA, giant cell arteritis; MRI, magnetic resonance imaging; NAION, nonarteritic anterior ischemic optic neuropathy.

Infiltration of the optic nerve head by cancer cells, inflammatory cells, and/or infectious organisms is another mechanism of optic nerve head elevation. Generally, these processes also incite concomitant optic disc swelling.
Some anomalous, but otherwise normal optic discs may be elevated, giving the false appearance of optic disc edema (Table 4–2; see Figure 4–43B).
DISTINGUISHING TRUE OPTIC DISC SWELLING FROM ANOMALOUS OPTIC DISCS (PSEUDOPAPILLEDEMA)
|
Rapid expansion of the optic disc from axonal swelling can cause damage to the optic disc and peripapillary vasculature, resulting in hemorrhages at many levels: deep, dark peripapillary subretinal hemorrhages; dot/blot intraretinal hemorrhages; nerve fiber layer hemorrhages (usually flame shaped); and (rarely) preretinal or vitreous hemorrhage (Figures 4–6 and 4–7). Cotton-wool spots are areas of nerve fiber layer infarction and can be seen on the optic disc as well as in the nerve fiber layer of the retina (Figure 4–8). Vascular changes can include telangiectasia on the disc surface (increased capillarity) or venous stasis (increased venous caliber). Telangiectasia on the disc and within the peripapillary retina can accompany Leber hereditary optic neuropathy (LHON; see Figure 4–35A). Collateral veno-venous vessels on the disc can be seen with optic nerve sheath meningioma, chronic papilledema, or compensated retinal vein occlusion. An increase in the diameter of the intraocular portion of the optic nerve from axonal swelling produces concentric peripapillary chorioretinal folds, called Paton lines (see Figure 4–6). Macular choroidal folds, oriented radially to the disc, frequently occur as a result of indentation of the posterior globe by an engorged optic nerve sheath expanded by high cerebrospinal fluid pressure, but can also occur in orbital Graves disease or from any intraorbital mass (Figure 4–9). Macular edema can occur with massive disc swelling of any cause (see Figure 4–8) but is most frequently seen accompanying optic disc edema in infectious or postinfectious autoimmune processes (neuroretinitis) (see Figure 4–32A).
Figure 4–6.
Severe optic disc edema.
Massive optic disc edema is present in this patient with intracranial hypertension and systemic hypertension. The optic nerve is elevated and enlarged. Hemorrhage is present in the nerve fiber layer (flame-shaped hemorrhages) and within the retina (dot/blot hemorrhages). The swollen nerve elevates and laterally displaces the choroid and retina, creating concentric chorioretinal folds called Paton lines.

Figure 4–7.
Optic disc edema with retinal hemorrhages.
This patient with papilledema has hemorrhages in the nerve fiber layer (NFL) of the retina, producing flame-shaped hemorrhages, as seen in the right eye. The shape of the hemorrhages reflects the anatomic arrangement of the nerve fiber layer in the inner retina. The left eye has preretinal hemorrhages (arrows) in addition to NFL hemorrhages. Preretinal blood can flow over the surface of the retina, constrained only by the vitreous (hyaloid). This type of hemorrhage is called subhyaloid. Preretinal blood can also break through into the vitreous and create a diffuse vitreous hemorrhage, obscuring the view of the retina.

Figure 4–8.
Optic disc edema with cotton-wool spots.
The white patches on the surface of this disc with grade 5 papilledema are infarcts of the nerve fiber layer, called cotton-wool spots. Their presence suggests ischemia. Though more common in ischemic conditions such as malignant hypertension, they can be present in severe optic disc edema from any cause. Also note the macular edema residues forming a partial macular star.

Figure 4–9.
Choroidal folds and optic disc edema in idiopathic intracranial hypertension.
This clinical photograph shows linear macular striations (arrows) that can occur when the expanded optic nerve sheath indents the posterior globe, shortening the axial length of the eye (which induces a hyperopic refractive shift).

Anterior ischemic optic neuropathy (AION), as its name implies, is the result of ischemia. The designation of anterior indicates that the ischemic insult causes damage to the optic nerve head and is visible with the ophthalmoscope. AION is thought to be caused by hypoperfusion of one or more of the short posterior ciliary arteries that supply the optic nerve head. Nonarteritic AION (NAION) is very common, and is most likely the result of vascular insufficiency from thrombosis and/or hypotension in patients with atherosclerotic disease. Arteritic AION occurs less often (5–10% of AION), and is caused by vasculitic occlusion of the arterial supply to the optic nerve head due to giant cell arteritis (GCA). This more devastating form of AION can cause bilateral blindness, as well as significant systemic vascular morbidity.
The term posterior ischemic optic neuropathy (PION) indicates an infarct of the optic nerve posterior to the optic disc, with a normal-appearing optic disc initially. PION is rare (due to the robust vascular supply of the retrobulbar optic nerve as discussed earlier), and occurs almost exclusively in two settings: vasculitis (mainly GCA), or profound blood loss and /or hypotension that occurs during surgery or as a result of trauma.
NAION is a common cause of sudden, painless, monocular visual loss in adults typically 50 to 70 years old (Figure 4–10). Systemic hypertension (50%) and/or diabetes mellitus (25%) are commonly present. Frequently, patients have other systemic manifestations of atherosclerotic disease such as angina pectoris, previous myocardial infarctions, or history of stroke. Sleep apnea, migraine, renal dialysis, and smoking are other potential associated conditions.
Figure 4–10.
Nonarteritic anterior ischemic optic neuropathy.
A 62-year-old man awoke with painless visual loss in his left eye. (A) A dense inferior altitudinal visual field defect was present in the left eye (the visual field in the right eye was normal). (B) The right disc was normal, but note the absence of a central cup, showing that this patient has the typical cupless “disc at risk” for AION. The left optic disc was swollen. The optic disc edema primarily involved the upper half of the optic disc, corresponding to the inferior visual field defect. (C) After 6 weeks, the upper pole of the left optic disc was less swollen, but pale. Paradoxically, the lower pole appeared more swollen, with telangiectatic vessels that may represent “luxury perfusion” to the remaining normal nerve. (D) After 6 months, the upper portion of the left optic disc became pale, and the lower half remained pink. (E) Time course of visual loss. The visual loss in this disorder is sudden, but may decline stepwise over several days. Some patients may have slight improvement in their central vision with time, but the visual field defect is typically permanent.

Visual loss in this condition ranges from profound to mild, and is frequently first noted by patients when they awaken from sleep. The visual loss is generally painless but can be associated with a mild ocular ache. The visual deficit is usually stable after onset, but some patients may have a stepwise decline in vision over several days. A subset of patients (5–10%) has a relentlessly progressive stepwise decline in vision for weeks following the initial event.
The visual field loss is commonly a mix of central and altitudinal visual field defects (Figure 4–10A), but any disc-related visual field defect can occur. Not uncommonly, patients are quite specific in their history, stating that they have lost the lower (or upper) half of their vision. Occasionally, central acuity is spared, but the vast majority of the time the visual field defect includes central vision.
The optic disc swelling is frequently ruddy or hyperemic and may be diffuse or segmental. For example, a dense inferior altitudinal visual field defect may be associated with segmental swelling of the superior pole of the optic disc, with relative preservation of the inferior pole (see Figure 4–10B). The discs are commonly small and cupless “disks at risk,” a finding that may only be appreciated by viewing the contralateral (unaffected) optic disc (see Figure 4–10B). This finding is so widespread that patients thought to have NAION who have a moderate or large cup-to-disc ratio should be considered to have some other process (such as arteritic AION) until proven otherwise. Peripapillary nerve fiber hemorrhages frequently accompany the disc edema. The remainder of the fundus is generally unremarkable, although retinal arterioles may reflect evidence of systemic hypertension, diabetes, or atherosclerotic disease. The disc swelling generally resolves within 3 to 6 weeks, leaving optic disc pallor (see Figure 4–10C, D).
NAION is thought to be a stroke of the optic nerve head, resulting from insufficient blood flow through the posterior ciliary arteries. Atherosclerosis that accompanies aging, or that is accelerated by systemic vascular diseases such as hypertension and diabetes, narrows the lumen to a critical level where thrombosis or even mild hypotension results in ischemia. The crowded, cupless optic discs commonly identified in these patients may initiate and perpetuate a cascade: ischemia causing edema, edema in the narrow confines of the crowded disc causing further ischemia from increased tissue pressure, with a downward spiral of increasing edema and ischemia.
The timing of this ischemic event, characteristically occurring at night and in the early morning, may be explained by nocturnal hypotension, a concept first described by Hayreh et al (1994). Systemic blood pressure is normally lower at night when patients are sleeping. Hypertension (and other vascular disorders) can damage arterial compliance and local autoregulation, such that nocturnal hypotensive swings reach a critical level that precipitates NAION. Long-acting antihypertensive medications may further accentuate nocturnal hypotension. Not infrequently, patients who present with AION report having been changed to stronger or longer-acting antihypertensive medication in the weeks or months before the event. Obviously, withdrawal of the patient’s antihypertensive medications is not the answer, but it is reasonable to suggest to the patient’s physician that long-acting antihypertensives be taken in the morning, or that shorter-acting drugs be considered to avoid nocturnal hypotension.
The most important consideration in the differential diagnosis is distinguishing NAION from arteritic AION caused by GCA (see Figure 4–5). In every case of AION, the physician should ask specific questions regarding the systemic symptoms of vasculitis (discussed below). AION may be difficult to distinguish from optic neuritis in adults aged 35 to 45 years, and the patients may need both possibilities investigated (Table 4–3). Table 4–1 lists other causes of optic disc elevation.
OVERLAPPING PROFILES: COMPARISON OF ARTERITIC AND NONARTERITIC AION, AND NONARTERITIC AION AND OPTIC NEURITIS
Arteritic and nonarteritic AION may be difficult to distinguish in older patients | |||
---|---|---|---|
Nonarteritic AION and optic neuritis may be difficult to distinguish in younger patients | |||
Arteritic AION (GCA) 5–10% of AION | Nonarteritic AION 90–95% of AION | Optic Neuritis | |
Age | >50 years (very rare <50 years) | 45–70 years | <45 years |
Sex | F > M | F = M | F > M |
Optic disc appearance | Usually pale edema, occasionally disc may be normal (in PION). Any cup/disc configuration. | Sectoral or diffuse hyperemic optic disc edema with peripapillary hemorrhage and cotton-wool spots. Cup/disc ratio small. | Only 1/3 have optic disc edema (2/3 have a normal disc). Usually disc edema is mild, without hemorrhage or cotton-wool spots. |
Eventual optic atrophy | Diffuse pallor, but can have cupping. | Sectoral or diffuse pallor (no cupping). | Diffuse pallor. |
Vision loss | Acute, severe. | Acute, less severe. | Subacute (declines over days/week). |
Other eye | 75% involved acutely if untreated. | Rarely involved acutely; 30% lifelong risk, 15% 5-year risk. | Unusual for adults to present with bilateral symptoms (but subclinical involvement of opposite eye by visual field in 50%). |
Pain | Scalp tenderness and headache. | Unusual. | Often have pain with eye movement that precedes vision loss. |
Other eye symptoms | TVL preceding AION, diplopia. | None. | Phosphenes acutely, and Uhthoff phenomenon after recovery. |
Systemic symptoms or findings | GCA symptoms: headache, scalp tenderness, jaw or tongue claudication, fever, night sweats, malaise, weight loss | Risk factors include hypercholesterolemia, hypertension, diabetes, other vascular disease. | MS symptoms: numbness, imbalance, diplopia, paraparesis, incontinence, others. |
Vision prognosis | Some can improve initially with IV steroids. | Visual field rarely improves (but visual acuity can improve some). | Dramatic improvement is the rule: over weeks to months. |
Immediate treatment considerations | Immediate institution of high-dose steroids to salvage vision and reduce risk to the other eye. | No proven treatment. Acute-phase corticosteroids may help Hayreh (2008). | Consider IV methylprednisolone as per ONTT; do not use low-dose oral corticosteroids alone. |
Labs | Elevated ESR and CRP (but not always). | Normal ESR and CRP. | Normal ESR and CRP. |
MRI | Only helpful to exclude other entities; optic nerve enhancement possible. | Only helpful to exclude other entities; optic nerve enhancement very rarely. | Helpful; may show optic nerve enhancement or white matter changes suggestive of MS. |
IVFA | IVFA can show delayed filling of and staining of optic disc, and choroidal hypoperfusion. | Delay in filling and staining of optic disc, but usually no choroidal hyperperfusion. | No delay in filling. Disc will stain if swollen. |
A sedimentation rate, C-reactive protein, and complete blood count should be drawn in most cases to address the possibility of an arteritic cause. Patients without known diabetes or hypertension should be assessed for these two diseases, and it is reasonable to send patients to their medical physician for a thorough examination. Patients younger than 45 years without known risk factors should have a serological evaluation for hyperlipidemia, vasculitis (rheumatological studies), hypercoagulable states, and syphilis (Table 4–4). Patients with symptoms suggestive of sleep apnea may need a sleep study.
HEMATOLOGIC ABNORMALITIES THAT CAN CAUSE VASCULAR OCCLUSIONS
Hypercoagulable States
|
Erythrocyte Disorders
|
Leukemias |
Neuroimaging is generally not indicated as long as the history is convincing for AION (sudden onset, stable course), and the examination is consistent (optic disc swelling). If optic neuritis is a consideration, magnetic resonance imaging (MRI) may be needed to assess the possibility of MS. Patients who present with recent visual loss and optic nerve pallor may require neuroimaging to evaluate for possible compressive neuropathy (such as a meningioma, parasellar or sellar tumor, or compressive orbital Graves disease).
No proven, effective treatment is known for NAION. However, oral or intravenous corticosteroids in the acute phase are thought to be effective by some authorities, such as Hayreh and Zimmerman (2008). The use of optic nerve sheath fenestration in this condition was explored by the ischemic optic neuropathy decompression trial (IONDT). The study showed that this surgery was not beneficial and may even be harmful. The use of a daily aspirin may decrease the risk of an event in the contralateral eye, as discussed below. Obviously, underlying precipitating factors should be addressed, such as blood loss, anemia, sleep apnea, or factors inducing hypotension. As discussed above, a patient’s medications for systemic hypertension should be reviewed. A potential association of NAION with drugs for erectile dysfunction (phosphodiesterase-5 inhibitors) has been proposed, but a causative link is not convincing. It is reasonable to caution patients who have had one eye affected regarding the potential risk of these drugs.
Patients should have repeat visual fields after several weeks and after several months, to ensure that the visual field loss is not progressive. Once NAION has finished its course and optic pallor ensues, this process is unlikely to occur again in the same eye. One proposed rationale is that the death of axons from an ischemic event frees up space for the remaining axons, effectively reversing the crowding of the optic disc. About one-half of patients with NAION may experience minimal improvement in visual function with time, usually manifest as slight improvement in visual acuity (see Figure 4–10E). The incidence of occurrence in the fellow eye is 20% to 40%, although it generally occurs years later. Simultaneous or sequential events in both eyes should raise strong suspicions of a vasculitic (eg, GCA) or other cause (eg, amiodarone toxic optic neuropathy). Unlike retinal vascular events, NAION has not been shown to be associated with an increased risk of cerebrovascular or cardiovascular events.
Arteritic anterior ischemic optic neuropathy is caused by vasculitic closure of the posterior ciliary arteries from GCA. The visual loss is usually more profound than nonarteritic AION, and may occur in both eyes at the same time or in rapid succession. Patients with GCA are usually older than 60 years, and the disease becomes more common with each decade of life. Arteritic AION is unusual before 60 years of age, and only a small number of patients with this diagnosis in their 40s have been documented. Among patients with GCA, women outnumber men 3 to 1, and it is much less common in African-Americans.
GCA is an idiopathic systemic vasculitis consisting of inflammation in the wall of small and medium-sized arteries, usually involving the extracranial arteries of the head. The inflammatory process can greatly expand the wall thickness, obliterating the lumen of the vessel and obstructing blood flow, with resultant ischemic consequences (Figure 4–11).
Figure 4–11.
Arteritic anterior ischemic optic neuropathy (giant cell arteritis).
A 74-year-old woman described brief episodes of visual loss in her right eye for several days, followed by a “skim” over the right eye 2 days before her evaluation, and awoke the next day with “no vision” in the right eye. She reported general malaise and tenderness of her scalp over the temples for two weeks. The examination showed light perception vision only in the right eye, with pale optic disc swelling. Although the erythrocyte sedimentation rate (ESR) was relatively normal for her age (41 mm/hr), giant cell arteritis (GCA) was suspected on the basis of the history and examination. The patient was admitted for high-dose intravenous steroids, and a temporal artery biopsy confirmed the diagnosis of GCA. (A) Pale optic disc edema was present in the right eye. (B) Temporal artery exposed at biopsy. The artery appeared large and pale. (C) Temporal artery cross section. Hematoxylin and eosin (H&E) stain, x100. The lumen is obliterated by massive thickening of the arterial wall. Fracture of the internal elastic lamina is seen (arrow). (D) A multinucleated giant cell is seen at higher magnification (circled).

Patients with GCA may present with rapid and profound blindness from ischemic optic neuropathy in one or both eyes. Permanent visual loss is frequently preceded by transient visual loss lasting seconds to minutes, similar to amaurosis fugax (see Table 1–3). Although the visual loss itself is painless, patients commonly complain of a new (more or less continuous) headache, scalp tenderness, and jaw claudication. Scalp tenderness may be caused by inflamed arteries in the scalp, or be secondary to scalp ischemia. Scalp tenderness may be so intense that patients complain of pain when lying on a pillow, combing their hair, or wearing a hat. Jaw pain with chewing, pain on swallowing, and talking-induced tongue pain can result from claudication of the involved muscles. Additional manifestations of this systemic vasculitis include weight loss, poor appetite, generalized malaise, myalgia, arthralgia, and low-grade fever.
Polymyalgia rheumatica (PMR) is a chronic rheumatological disorder characterized by proximal shoulder and buttock pain without tenderness. PMR may be a precursor or concomitant accompaniment of GCA.
Visual field defects from arteritic AION may be similar to those found in nonarteritic AION, though the visual acuity and field are frequently much more severely affected. Not uncommonly, patients present with NLP (no light perception) vision. The optic disc appearance may be indistinguishable from nonarteritic AION, but more commonly it is diffusely edematous and chalky pallid (see Figure 4–11A). On occasion, arteritis can cause a posterior ischemic optic neuropathy (PION), in which the optic disc appears normal or is only minimally affected acutely. Acute, profound visual loss in a patient older than 55 with an unimpressive disc and retina, or in whom visual loss exceeds the observed optic disc edema, should be considered GCA until proven otherwise (Table 4–5). Bilateral or rapidly sequential AION also requires immediate consideration of GCA. In contrast to nonarteritic AION, glaucomatous-like optic disc cupping may develop following arteritic AION.
ACQUIRED CAUSES OF PROFOUND VISUAL LOSS WITH A RELATIVELY UNREMARKABLE FUNDUS EXAMINATION
Retrobulbar Optic Neuritis
|
Neuromyelitis Optica (Devic Disease)
|
Compressive Optic Neuropathy
|
Posterior Ischemic Optic Neuropathy
|
Acute Traumatic Optic Neuropathy
|
Disorders of the chiasm
|
Retrochiasmal Lesions
|
Retrobulbar Inflammatory or Infiltrative Disorder
|
Retinal Artery Occlusion
|
Paraneoplastic Optic Neuropathy and Retinopathy
|
Toxic and Nutritional Optic Neuropathies (and Toxic Maculopathies)
|
The occasional occurrence of a retinal infarct in the distribution of a cilioretinal artery in the setting of AION is highly suggestive of arteritis. This association is not surprising because the optic disc and peripapillary choroid share a common blood supply—the short posterior ciliary arteries. Ophthalmic artery involvement can cause simultaneous ischemic optic neuropathy and central retinal artery occlusion, a rare combination that can be caused by the profound ischemia possible from GCA.
Inflamed temporal arteries (or other scalp arteries) can frequently be palpated as a firm “cord” with a poor or absent pulse, explaining this disorder’s alternate name: temporal arteritis. Fortunately, these frequently involved arteries are also easily surgically accessible for biopsy.
Visual loss occurs in 30% to 40% patients with GCA. If the disorder is unrecognized and untreated, visual loss in the second eye can occur in up to three-quarters of patients with arteritic AION, usually within several weeks. Early recognition of this process is vital to prevent bilateral blindness. Other vascular consequences of GCA are listed in Table 4–6.
ISCHEMIC MANIFESTATIONS OF GIANT CELL ARTERITIS (GCA)
Ophthalmic
|
Systemic
|
The evaluation of patients presenting as AION is directed at determining whether the process is arteritic or nonarteritic, as discussed in previously (see Figure 4–5). Fluorescein angiography has been advocated by some experts as a potential way of distinguishing these two entities. Patients who have profound insufficiency of the posterior ciliary arteries resulting from GCA may demonstrate areas of choroidal hypoperfusion on the early sequences of intravenous fluorescein angiography (IVFA), with a marked delay in choroidal filling (Figure 4–12).
Patients with prominent bilateral optic disc edema and minimal visual loss are more likely to have papilledema from elevated intracranial pressure than bilateral arteritic AION, as the visual loss is typically profound in arteritic AION.
Patients older than 55 with acute, profound visual loss but minimal optic disc or retinal findings may have posterior ischemic optic neuropathy (PION) from GCA; other entities in the differential diagnosis are listed in Table 4–5.
Although no pathognomonic blood test is available for identifying GCA, an elevated Westergren sedimentation rate (usually >50) and elevated C-reactive protein (CRP) add weight to the clinical suspicion of this disorder. Thrombocytosis, anemia of chronic disease, and mild liver enzyme abnormalities may be present as well.
The erythrocyte sedimentation rate (ESR) is a nonspecific indicator of inflammation, and is also elevated in patients with infectious, collagen-vascular, renal, or neoplastic disorders, and in diabetic patients. The normal sedimentation rate increases with age. The upper limit of normal for a given age can be estimated by dividing the age by two in men, and adding 10 before dividing by 2 in women. Even with this criterion, some patients with an elevated ESR have no demonstrable disease. On the other hand, the ESR may be normal in up to 20% of patients who have GCA. Therefore, this important clinical test must be interpreted in the context of the patient’s other signs and symptoms. An elevated CRP has proven to be more sensitive (100% in one study), and the combination of an elevated CRP and ESR yields a specificity of 97% for GCA.
A definitive diagnosis can be made only by identifying characteristic pathological features in a biopsy of an affected artery (see Figure 4–11C, D). A temporal artery biopsy should be performed on all patients suspected of having GCA, even those patients in whom the clinical diagnosis seems certain. A positive biopsy may not change the short-term treatment plan, but it is invaluable in managing the patient in the months and years following the diagnosis, particularly if patients develop significant systemic morbidity related to steroid therapy.
The vasculitis in GCA is not continuous, and may be found in patches along an artery with normal or healing intervening areas. Therefore, temporal artery biopsies should be at least 2 to 3 cm in length, because a short biopsy specimen, or one that is not completely serially sectioned, may miss the pathological area. A single arterial specimen is diagnostic in 80% to 90% of patients who have GCA. Most physicians advocate a biopsy of the contralateral temporal artery or other symptomatic scalp artery if the initial temporal artery biopsy is negative in a patient with compelling clinical findings. Biopsy of two sites increases the sensitivity to greater than 90%. Some physicians biopsy the temporal artery on both sides during the same surgery.
Thus, a patient suspected of having GCA should have a sedimentation rate and C-reactive protein drawn, and steroids should be immediately administered (described in more detail below). A temporal artery biopsy should be performed within 1 to 2 weeks of beginning steroids if possible, as the rate of a definitively positive biopsy (showing diagnostic giant cells) will decrease after several weeks of steroid therapy. However, even after months of treatment, discontinuities of internal elastic lamina may still be identified, suggesting “healed” arteritis.
The clinical presentation, ESR, CRP, and temporal artery biopsy are usually sufficient to establish a definitive diagnosis. In atypical cases, neuroimaging may be required, and the differential diagnoses outlined in Figure 4–5 and Table 4–5 will need to be addressed. GCA is by far the most common vasculitis causing ischemic optic neuropathy in patients older than 50 years, but occasionally laboratory studies may be needed to address other vasculitides such as systemic lupus erythematosus, polyarteritis nodosa, herpes zoster, or Churg-Strauss disease.
Immediate administration of steroids may prevent bilateral blindness—an outcome that is not uncommon when the diagnosis or treatment is delayed. In patients with acute visual loss, high-dose intravenous steroids may actually recover some vision. A common regimen is 250 mg of intravenous methylprednisolone every six hours over several days. This treatment often requires hospitalization based on the age and potential for medical complications in these patients. Patients without acute visual loss but with clinical findings suggestive of GCA should be started immediately on 60 to 80 mg of oral prednisone daily, until a biopsy can be performed to determine further management.
Patients with GCA frequently require oral corticosteroids for 1 year or more. Most patients can be tapered to lower doses over a period of months by careful monitoring of the patient’s sedimentation rate, C-reactive protein, and symptoms. Collaboration with the patient’s medical physician is of paramount importance, given the potential systemic complications of corticosteroid therapy. Occasionally, immunosuppressive therapies such as methotrexate may be added as a steroid-sparing agent, to permit a more rapid taper of prednisone (particularly in diabetic patients).
Diabetic patients with optic disc edema and visual loss may have diabetic papillopathy (also called diabetic papillitis). This condition has features that suggest it is clinically different from typical nonarteritic AION: (1) the patients are younger (aged 15–40 years), (2) visual field defects and visual acuity loss is much less severe, (3) the condition is often bilateral, and (4) the visual deficits are more likely to improve with time (Figure 4–13). Though more common in patients with type I diabetes, this condition can also occur in patients with type II diabetes. The optic disc edema is usually diffuse rather than focal, and the discs display a fine, diffuse telangiectasia that may be difficult to distinguish from neovascularization. When it is bilateral, diabetic papillitis may mimic papilledema (often referred to as “diabetic pseudopapilledema”). Diabetic papillitis and AION may be indistinguishable in some cases. The precise etiology of diabetic papillitis is uncertain, but likely represents a form of optic nerve head ischemia. There is no proven effective treatment, though oral, periocular, and intravitreal steroids have been advocated.
Figure 4–13.
Diabetic papillopathy (papillitis).
A 37-year-old insulin-dependent diabetic patient described a sudden change in the vision of his left eye. (A) The right optic disc is not swollen, but diabetic retinopathy is evident in both eyes. The left optic disc is diffusely swollen with disc and peripapillary hemorrhages. (B) Automated perimetry of left eye showed only a small inferior nasal step, far less than expected from the degree of optic disc swelling. The visual acuity was 20/80. The visual field in the right eye was normal. (C) Three months later, the optic disc swelling in the left eye was nearly resolved, with mild pallor remaining. The central acuity improved to 20/40, but the visual field defect remained unchanged.

Papillophlebitis is a poorly defined cause of unilateral optic disc swelling that occurs most commonly in healthy patients aged 20 to 30 years. Patients present with painless, minimal visual loss. The optic disc shows diffuse hyperemic disc swelling, with dilated veins and flame-shaped peripapillary hemorrhages. The optic disc appearance suggests a central retinal vein occlusion (CRVO), but the hemorrhages do not extend far from the disc, and are not found in the retinal periphery (Figure 4–14). This entity is most likely a variant of central retinal vein occlusion, and the clinical evaluation should be the same as a CRVO in a young person: assessment for hypercoagulable states (see Table 4–4).
Figure 4–14.
Papillophlebitis.
A 35-year-old man described a “smudge” in the vision of his right eye. The best corrected visual acuity was 20/40 in the right eye and 20/20 in the left eye. (A) Optic disc edema with peripapillary hemorrhages, cotton-wool spots, and venous engorgement was present in the right eye. Unlike a central retinal vein occlusion, no hemorrhages were present in the retinal periphery. The fundus abnormalities and symptoms gradually cleared over 4 months. (B) Automated perimetry of the right eye shows minimal diffuse depression with a mean deviation of −4.43. The left eye had a normal visual field (mean deviation of −2 decibels).

Radiation optic neuropathy (RON) is a cause of subacute, painless visual loss, typically occurring years after radiation therapy (external beam and gamma knife) for ocular or central nervous system (CNS) tumors. It can occur with optic disc edema, similar to AION, or as a retrobulbar or chiasmal process with normal-appearing optic discs initially. The vision loss is usually stepwise progressive and is severe. Patients may have evidence of concomitant radiation retinopathy or CNS radionecrosis.
The clinical scenario is unusual in that RON occurs as a sudden event 1 to 2 years (or more) after the radiation treatment. This acute event occurs as an ischemic process from an occlusive microvasculopathy, likely as a culminating event set in motion by initial damage to arteriolar and capillary endothelial cells.
Evaluation of patients with RON must address the possibility of recurrence of the original tumor or other processes. Corticosteroids, hyperbaric oxygen, and anticoagulation have been used with limited success.
Optic neuritis typically causes acute monocular visual loss in young adults aged 15 to 45 years, associated with pain induced or worsened by eye movement (Figure 4–15). Three-quarters of patients with optic neuritis are women. The term optic neuritis means inflammation of the optic nerve, and therefore could be used to describe any condition that causes optic nerve inflammation. However, most physicians reserve this term for primary demyelinating events such as the optic neuropathy associated with MS or idiopathic conditions that have a similar clinical course.
Figure 4–15.
Optic neuritis and multiple sclerosis.
A 32-year-old woman had pain with eye movement and decreased vision in her right eye. (A) The initial visual field of the right eye showed dense visual field loss in three quadrants. The visual acuity was 20/80 and a 1.2 log unit relative afferent pupillary defect (RAPD) was present. The left eye was normal. (B) Mild diffuse disc edema was present in the right eye; the left optic disc was normal. (C) Magnetic resonance imaging (MRI) of the orbits (T1-weighted image with contrast, coronal view) revealed enhancement of the right optic nerve (arrow). (D) MRI of the brain (T2-weighted image, axial view) revealed periventricular white matter plaques characteristic of multiple sclerosis (MS) (arrows). Additional T2 signal abnormalities were seen in the right pons (not shown). As recommended by the Optic Neuritis Treatment Trial (ONTT), three days of high-dose intravenous methylprednisolone were administered. (E) Within 3 weeks of onset, the visual field demonstrated dramatic improvement. A shallow central scotoma remained, with a visual acuity of 20/40. Eventually the visual field and acuity normalized, but a small RAPD was still present 2 years later. (F) Time course of visual loss. Optic neuritis typically causes a decline in vision over several days to a week, followed by a slow but steady recovery over several months. Patients often achieve near-normal visual acuities and visual fields, but may indefinitely note a subjective difference in the brightness or quality of vision in the affected eye.

Much of the clinical knowledge regarding optic neuritis comes from the Optic Neuritis Treatment Trial (ONTT), a National Institutes of Health (NIH)-funded, national, prospective, randomized study that evaluated the treatment of acute optic neuritis. This important study provides information regarding not only the treatment of optic neuritis, but also the presentation characteristics, visual outcome, and risk of MS in patients with optic neuritis.
Optic neuritis usually causes monocular visual loss, described by patients as a haze, cloud, or dimness with poor color vision. The visual loss may progress over 2 to 7 days, at which point the vision begins to slowly improve, with normal or near-normal visual function usually achieved over several weeks or months (see Figure 4–15F). Retrobulbar pain, made worse with eye movement, usually precedes visual symptoms and continues during the phase of visual decline. As discussed above, the pain likely originates from extraocular muscle traction on an inflamed optic nerve at the annulus of Zinn. Occasionally, patients report seeing brief spots of light (phosphenes, photopsias) induced by eye movements or loud sounds. A neurological history and review of symptoms may reveal previous or concomitant neurological events that suggest a diagnosis of MS, such as transient episodes of numbness, weakness, loss of bowel or bladder control, or imbalance.
Many patients with active or recovered optic neuritis may note dimming of vision in the affected eye when their body temperature is elevated, such as after exercise, after a shower or sauna, or with a fever. This transient visual loss is called Uhthoff phenomenon and results from temporary impairment of optic nerve fiber conduction, which may be caused by elevated body temperatures or changes in the pH or ion content of the blood.
Visual acuity is usually affected. Color vision and contrast sensitivity deficiencies may be more severe than would be predicted from visual acuity alone (see Figure 2–7C). Potential visual field defects include any optic disc–related visual field abnormality. Although a central scotoma is the classic finding, altitudinal visual field defects are also at least as common (and were more common in the ONTT than central scotomas), making the distinction between this disorder and AION difficult in some cases. It is not unusual to find slight visual field abnormalities in the asymptomatic eye. The RAPD is frequently measurably larger than expected from the visual field defect. However, patients who have had optic neuritis in the fellow eye may have a minimal or no RAPD. Even after a patient’s visual acuity, visual field, and color vision have recovered, the RAPD tends to persist, as does the brightness sense disparity between an affected and unaffected eye.
The prolonged conduction velocity of an affected optic nerve can be demonstrated as a delay (increased latency) in the signal generated with visually evoked potential (VEP) testing. This test is not useful in the diagnosis of optic neuritis in a symptomatic eye, as common clinical tests of visual function present more compelling evidence of a previous or current optic neuritis. However, VEP testing is employed frequently by neurologists seeking evidence of demyelination in multiple sites in non-ocular, mono-symptomatic patients suspected of having MS. The Pulfrich phenomenon (see Box 2–4) may be observed by patients with unilateral optic neuritis, as a direct consequence of delayed signal conduction in an affected optic nerve.
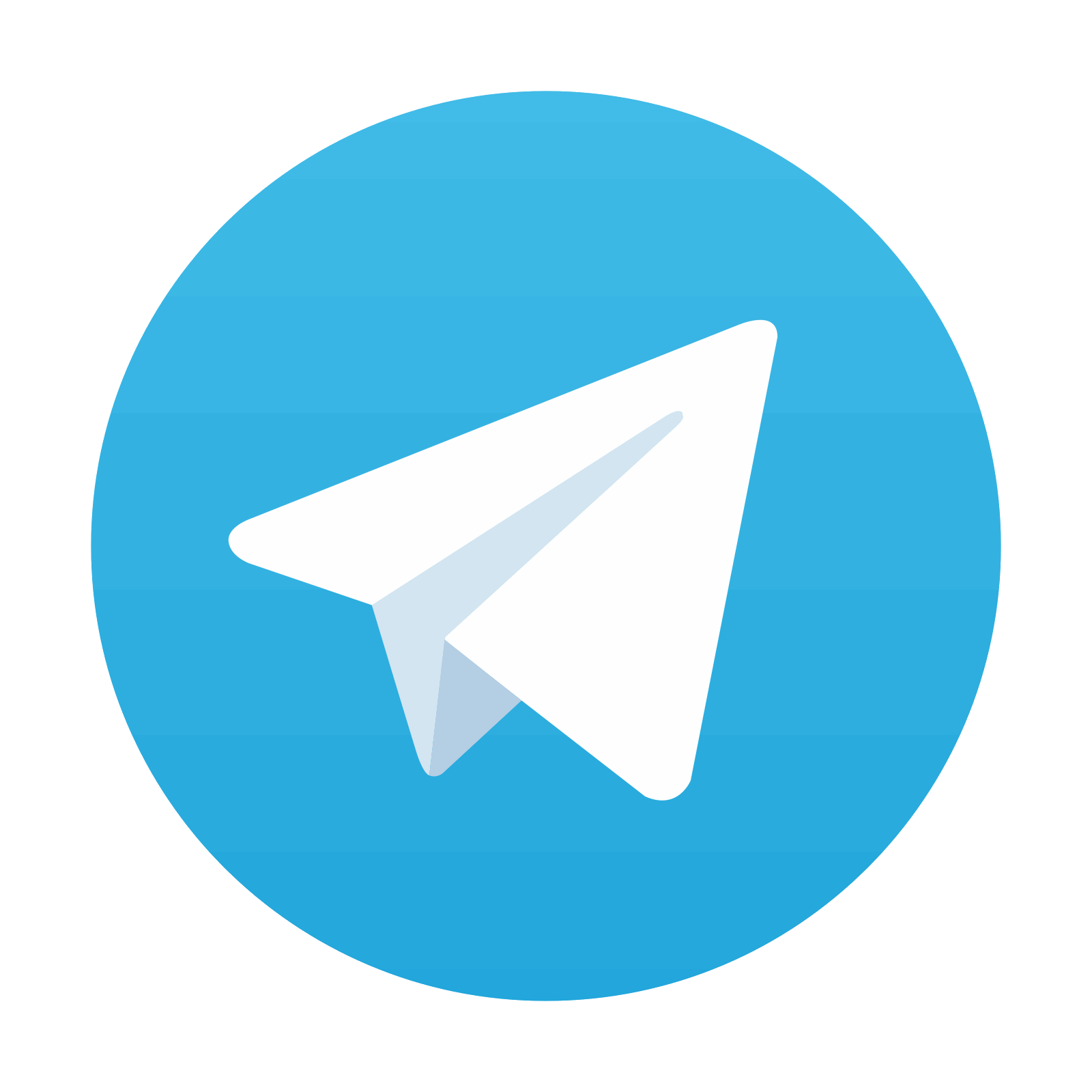
Stay updated, free articles. Join our Telegram channel

Full access? Get Clinical Tree
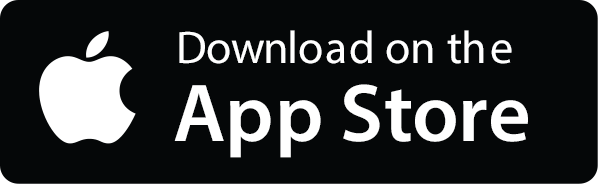
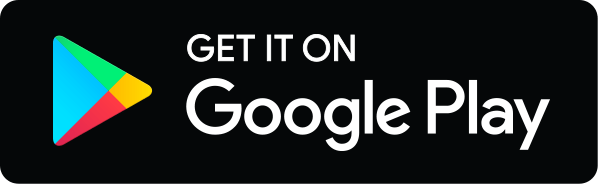