Brian C. J. Moore, Roy D. Patterson, Ian M. Winter, Robert P. Carlyon and Hedwig E Gockel (eds.)Advances in Experimental Medicine and BiologyBasic Aspects of Hearing2013Physiology and Perception10.1007/978-1-4614-1590-9_12© Springer Science+Business Media New York 2013
12. On the Limit of Neural Phase Locking to Fine Structure in Humans
(1)
Laboratory of Auditory Neurophysiology, University of Leuven, Herestraat 49 bus 1021, Leuven, B-3000, Belgium
Abstract
The frequency extent over which temporal fine structure is available in the human auditory system has recently become a topic of much discussion. It is common, in both the physiological and psychophysical literature, to encounter the assumption that fine structure is available to humans up to about 5 kHz or even higher. We argue from existing physiological, anatomical, and behavioral data in animals, combined with behavioral and anatomical data in humans, that it is unlikely that the human central nervous system has access to fine structure above a few kHz.
1 Introduction
The issue of temporal versus place coding has occupied hearing researchers for over a century. It is not just an academic issue as it is relevant for the remediation of hearing impairment, e.g., via cochlear implants. Unfortunately, it is at present not possible to apply the most incisive behavioral and physiological techniques within the same species. Thus, physiological findings in nonhuman species are typically invoked to interpret human hearing, which raises questions regarding the physiological similarity between laboratory and human species. We argue that coding of fine structure in human is limited to lower frequencies than in cat.
2 Neural Phase Locking to Fine Structure
Broadly defined, phase locking of sensory neurons refers to their ability to represent some temporal aspect of a stimulus waveform. By “representation” is usually meant an increased and/or decreased probability that the neuron fires action potentials (spikes) at specific time points of the stimulus waveform. In the context of auditory physiology, it is customary to distinguish phase locking to fine structure, envelope, and transients, which differ in their underlying physiological mechanisms. Here, we use the term phase locking in the restricted sense of a modulation of firing rate locked to stimulus fine structure, i.e., to instantaneous, cycle by cycle, pressure fluctuations. Phase locking to fine structure can in principle be studied with any sound, but in practice single tones have been the stimulus of choice. They indeed deserve their status of purity in this context because of their perfectly flat envelope. Ignoring onset or offset transients, the sustained part of the neuronal response allows a straightforward assessment of phase locking to fine structure. For reasons of space, we base our arguments mainly on pure tone responses in the cat – the animal which has been characterized most extensively – but the general trends we describe are, at least partly, observed in guinea pig, rabbit, chinchilla, gerbil, monkey, and barn owl.
3 Human Behavioral Limits Reflecting Phase Locking to Fine Structure
Many attributes of auditory perception have been proposed to involve or require neural phase locking to fine structure. Because changes in stimulus waveform are typically accompanied by changes in response dimensions other than fine structure (spatial pattern of excitation, temporal envelope), assessment of its role requires indirect arguments. The exception is binaural hearing, for which it is beyond doubt that neural coding and processing of fine structure are essential. Many studies have explored sensitivity to interaural differences in phase and time. Again, pure tones are purest: for any other stimulus, interactions between stimulus components may create envelope fluctuations underlying behavioral sensitivity.
Humans show exquisite sensitivity to interaural phase differences up to 1.4 kHz (Zwislocki and Feldman 1956). A similar limit on the use of fine structure is suggested by nontonal stimuli (Klumpp and Eady 1956; Schiano et al. 1986). Besides ITD jnds, other perceptual attributes that are necessarily based on a binaural comparison of fine structure show a similar upper frequency limit (Licklider et al. 1950; Perrott and Musicant 1977) of ∼1.5 kHz. Dunai and Hartmann (2011) recently traced the upper frequency limit for tonal ITD discrimination in fine steps: in all four subjects, the highest frequency at which ITD sensitivity could be measured was 1,400 or 1,450 Hz.
4 Physiological Limits of Phase Locking to Fine Structure
Early studies indicated that the existence range of pure-tone neural phase locking extends up to several kHz (Rose et al. 1967; Johnson 1980). The most used metric is the vector strength (Goldberg and Brown 1969). A plot across nerve fibers of maximal vector strength as a function of characteristic frequency (CF), in response to CF tones, suggests a low-pass filter (Johnson 1980). Likewise, a within-fiber plot of maximal vector strength as a function of frequency has a monotonically decreasing shape (Rose et al. 1968; Joris et al. 1994a). When other metrics are used, such as the temporal width of the period histogram (Joris et al. 1994b) or width and height of autocorrelograms (Louage et al. 2004), the low-pass filter characterization appears less adequate. For simplicity we here take the upper limit of phase locking as the highest frequency at which significant phase locking is found, but the exact definition is not critical to our argument. The upper limit of phase locking differs between species (Weiss and Rose 1988). In cat, vector strength starts to decrease at about 1 kHz and becomes insignificant at ∼5 kHz.
The auditory nerve projects to the cochlear nucleus. Here, the phase-locking limit clearly differs between subnuclei and cell types. In the anteroventral cochlear nucleus (AVCN), bushy cells show exquisite phase locking, but their upper cutoff of significant phase locking is somewhat lower than in the AN (∼4 kHz, Bourk 1976; Rhode and Smith 1986; Blackburn and Sachs 1989; Joris et al. 1994a). AVCN stellate cells can show excellent phase locking at very low frequencies (Joris et al. 1994b), but phase locking is clearly degraded in terms of upper frequency limit (Bourk 1976; Rhode and Smith 1986; Blackburn and Sachs 1989), and the cells are biased towards high CFs (Melcher 1993). The posteroventral cochlear nucleus contains neurons with exquisite phase locking to low-frequency tones (Godfrey et al. 1975; Rhode and Smith 1986; Smith et al. 2005). The nucleus is biased towards high CFs, and its output neurons receive highly convergent input from the auditory nerve and show wide tuning. Octopus cells show sustained phase-locked responses at low frequencies, but for tones above ∼2 kHz, it is rare to get more than a single pure onset response (Godfrey et al. 1975; Rhode and Smith 1986; Joris and Smith 2011). Multipolar cells do show sustained responses at all frequencies but have a lower upper limit of phase locking than the auditory nerve, as do octopus cells (Rhode and Smith 1986). Finally, the dorsal cochlear nucleus shows poor phase locking to fine structure (Goldberg and Brownell 1973) and is also biased towards high CFs (Spirou et al. 1993). In summary, it appears that in terms of upper limit, bushy cells best preserve the phase locking present in the AN. This is of little surprise since these cells receive a small number of powerful axosomatic terminals (the end bulbs and modified end bulbs of Held) from the auditory nerve (Ryugo and Rouiller 1988; Sento and Ryugo 1989) and have short membrane time constants by virtue of their intrinsic properties (reviewed in Young and Oertel 2004).
The main projection targets of bushy cells are the binaural circuits in the superior olivary complex. Spherical bushy cells give excitatory projections to the medial and lateral superior olive (LSO and MSO). Globular bushy cells give excitatory projections to glycinergic, inhibitory nuclei (the medial and lateral nuclei of the trapezoid body, MNTB, and LNTB) which in turn project to LSO and MSO. Both kinds of bushy cells show exquisite phase locking (reviewed by Joris and Smith 2008) and have a variety of specializations in their innervation, biophysical properties, and anatomy, including one of the largest synaptic terminals in the brain (the calyx of Held on the MNTB).
The use by the central nervous system of phase locking to fine structure in the peripheral auditory system is dramatically illustrated in the sensitivity, arising in the superior olivary complex, to interaural time differences (ITDs) to pure tones (equivalently referred to as sensitivity to interaural phase differences, IPDs). ITD sensitivity is generated both in the MSO and the LSO. Recordings in MSO show prominent ITD sensitivity to pure tones (Goldberg and Brown 1969; Yin and Chan 1990), but the upper limit has, unfortunately, not been characterized in cat. The LSO has only a small low-CF representation, and its ITD sensitivity to tones is weaker, in a number of respects, than in MSO (Joris and Yin 1995; Tollin and Yin 2005). Importantly, ITD sensitivity signifies a recoding from a temporal to a rate code. Put simplistically, phase locking to fine structure is “used” in MSO and LSO and is translated to a code of average rate. Thus, even though data at the level of the MSO are scarce, limits of ITD sensitivity in neurons at higher levels give insight in the highest frequency at which phase locking to fine structure affects the output rate of neurons.
The best studied projection target of the binaural nuclei in the superior olivary complex is the inferior colliculus (IC). In cat, the highest frequencies at which ITD sensitivity has been reported in IC are just below 3 kHz (2,860 Hz in Rose et al. 1966; 2.8 kHz in Yin and Kuwada 1983). This ITD sensitivity is thought to be largely inherited from the superior olivary complex. It is unclear whether this sensitivity is further modified by monaural phase-locked inputs at levels above MSO and LSO, e.g., through an interaction between monaural phase-locked input from the AVCN and binaural ITD-sensitive input from superior olivary complex. Phase locking in IC neurons is clearly limited to much lower frequencies than in auditory nerve or cochlear nucleus (Kuwada et al. 1984). Cochlear nucleus neurons that directly project to IC do not phase lock to the high frequencies to which bushy cells phase lock, so even if phase locking to fine structure would directly alter properties of ITD sensitivity in the IC, this could only be the case at low frequencies and would not affect the upper limit of ITD sensitivity.
Sensitivity to ITDs of fine structure is evident up to 3 kHz in cat thalamus (Ivarsson et al. 1988). Interestingly, significant IPD sensitivity in cat primary auditory cortex was found up to 2.5 kHz (Reale and Brugge 1990), though it is not clear from that report whether higher frequencies were tested. Thus, while the upper limit of phase locking to fine structure degrades with ascending anatomical levels, this does not appear to be the case for the upper frequency limit of ITD sensitivity, which is similar at different anatomical levels. This is not surprising. Once the temporal code is converted to a rate code, the sensitivity can simply be passed on to higher structures, and specializations such as large terminals and fast membranes are no longer required.
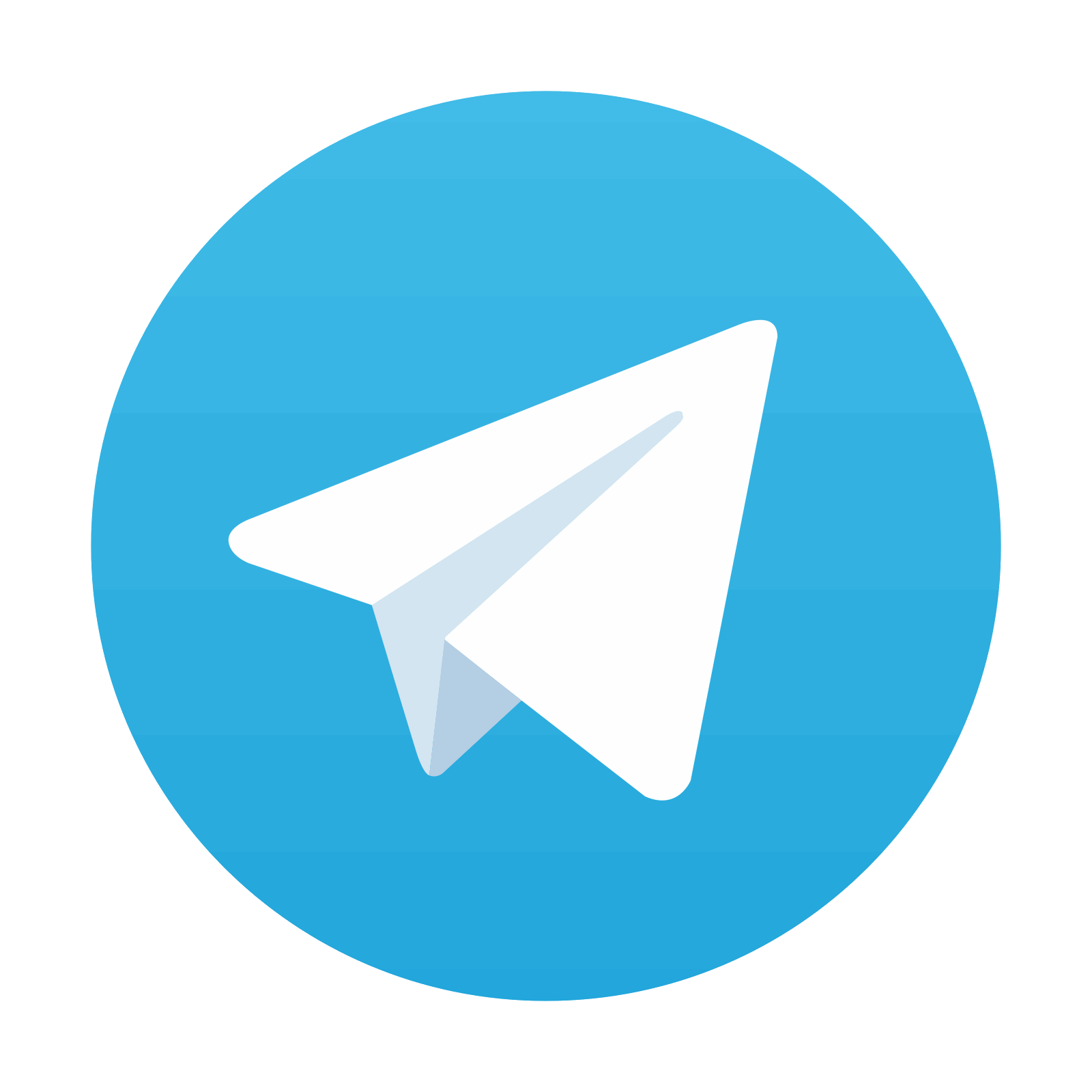
Stay updated, free articles. Join our Telegram channel

Full access? Get Clinical Tree
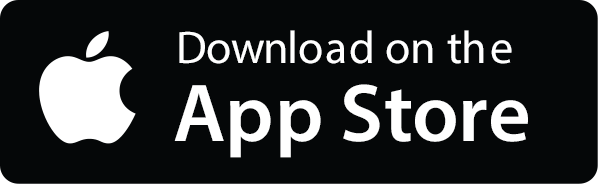
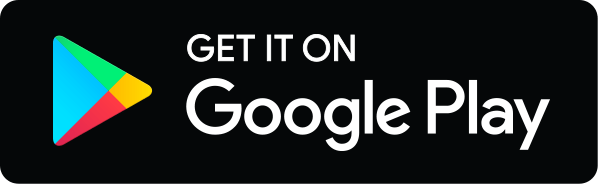