Laryngeal cartilaginous framework
Two additional structures provide structural support to the larynx: the quadrangular membrane and conus elasticus. The quadrangular membrane is an elastic structure that extends anteriorly to the lateral epiglottis, posteriorly to the arytenoids, superiorly to the aryepiglottic fold, and inferiorly to the medial wall of the pyriform sinus [6]. The conus elasticus is a fibroelastic structure that originates inferiorly along the cricoid cartilage and extends superiorly to the anterior commissure and vocal processes; it forms the vocal ligament medially and is continuous with the cricothyroid membrane anteriorly [6].
Muscles and Peripheral Innervation

Intrinsic muscles of the larynx
Extrinsic muscles of the larynx include infrahyoid and suprahyoid muscles and serve to maintain or alter the position of the larynx within the neck. This can include maintaining a stable laryngeal framework so that the intrinsic laryngeal muscles can work effectively in the setting of voice performance [8]. Infrahyoid muscles include the omohyoid, sternohyoid, sternothyroid, and thyrohyoid, with general function serving to lower position of the hyoid bone. Suprahyoid muscles include the stylohyoid, geniohyoid, mylohyoid, and digastric muscles, with contraction serving to raise the hyoid bone.
Laryngeal sensory innervation is from the internal branch of the SLN above the glottis and the RLN at and below the glottis.
Vocal Folds

Layered structure of the vocal fold
Hirano described the body-cover theory of the vocal fold, with the cover consisting of the surface epithelium, superficial lamina propria, and intermediate lamina propria, and the body consisting of the deep lamina propria and thyroarytenoid muscle [14].
Superior to the true vocal folds are false vocal folds, also known as the ventricular or vestibular folds. The ventricle lies between the true and false vocal folds. The false vocal folds aid in airway protection during swallow and can also contribute to normal voice production and timbre [15–17]. The false vocal folds also play a prominent role in Tibetan and Tuvan throat singing. Adduction of the false vocal folds is due to contraction of the ventricularis muscle, innervated by the RLN [16].
Blood Supply
Blood supply to the larynx is from the superior and inferior laryngeal arteries. The superior laryngeal artery arises from the superior thyroid artery, which arises from the external carotid artery. The inferior thyroid artery arises from the thyrocervical trunk, which arises from the subclavian artery. Venous drainage is via the superior and inferior laryngeal veins which arise from the thyroid veins.
Central Innervation
Nuclei for the motor neurons of the intrinsic muscles of the larynx are in the nucleus ambiguus [18]. Additional nuclei involved in control of voice production include the trigeminal motor nucleus in the pons, facial and hypoglossal nuclei in the medulla, and ventral horn of the spinal cord, with coordination accomplished via the ventrolateral parabrachial area, lateral pontine reticular formation, anterolateral and caudal medullary reticular formation, and nucleus retroambiguus [19]. Voluntary voice control requires input from the cerebral cortex, including Broca’s area, the supplementary motor area, and the pre-supplementary motor area [19].
Interestingly, multiple forebrain systems appear to innervate the thyroarytenoid muscles [20]. This includes the anterior cingulate, periaqueductal gray, and ventral respiratory group, which serve as the center for vocalization in primates [19]. The second system consists of the central nucleus of the amygdala, basal ganglia, thalamus, and hypothalamus, which contribute to swallowing, vocalization in response to aversive stimuli, and emotional vocalizations such as laughing or crying [21]. The ventrolateral preoptic area of the hypothalamus provides neural input to the thyroarytenoid during sleep [20].
Changes in Anatomy with Aging
The newborn larynx sits more superiorly in the neck at the level of the C4 vertebra compared to the level of C6–7 in adults. This leads to a shorter vocal tract and altered formant frequencies. The epiglottis has a more tightly curled contour, the vocal folds are about 2.5–8 mm long [22, 23], and the vocal fold lacks the layered architecture found in adults [24]. There is no well-defined vocal ligament, but this emerges beginning after age 1 and is observable after age 4 [23, 25]. As the child ages, cartilages also increase in size and change shape. The vocal folds develop a layered structure [24, 26]. By age 7, the depth of the superficial lamina propria is approximately that seen in the adult [27]. In terms of distribution of elastin and collagen and orientation of these fibers, the vocal fold layers do not approximate those of an adult until around age 13 [28]. Interestingly, hyaluronic acid (HA) in infant vocal folds has been found to be evenly distributed throughout, in contrast with the distribution in adult vocal folds, in which distribution of HA, elastin, and collagen vary by layer [29]. This has been hypothesized to have a protective effect against phonotraumatic damage from prolonged crying. The ratio of the membranous/cartilaginous glottis also changes with aging, from a ratio of approximately 1.5:1.0 in the newborn to a ratio of 4.0:1.0 in the adult female and 5.5:1.0 in the adult male [30, 31]. The altered ratio with effectively shortened membranous vocal fold may play a role in changing relative impact stresses and the high rate of nodules in children.
Fundamental frequency is 400–600 Hz with crying [32]. This decreases during the first few years of life in both males and females [4]. Further decreases in fundamental frequency occur from age 10 to 18 [33], with a downward shift of 12 semitones in males and 3–4 semitones in females [34]. Other pubertal changes in voice can include a reduction and then expansion in range, more difficulty negotiating register transitions in singing, increased breathiness, and transient difficulties with pitch control [35–37].
Vocal Fold Vibration


During phonation, the larynx acts as an energy transducer, transforming aerodynamic energy into acoustic energy. Image is courtesy of Dr. Erin Devine
Although the above description can explain how vocal fold oscillation is initiated, it does not completely explain how it is sustained. For that, consideration of changes in pressure above the glottis is required. When the vocal folds open, air accelerates through the glottis, and an air column of positive pressure moves superiorly into the vocal tract, which aids further in vocal fold opening. As the vocal folds close, the momentum of the air column continues, creating negative pressure which aids further in vocal fold closing. Vocal tract inertance with time-delayed changes in the supraglottal air column facilitates vocal fold opening and closing and allows for sustained oscillation [4].
Factors Affecting Vocal Fold Vibration
Subglottal Pressure
Subglottal pressure is the driving force of voice production. Two of the primary descriptors of vocal output, fundamental frequency (measured in Hz) and vocal intensity (typically measured as sound pressure level and measured in decibels (dB)), are dictated by subglottal pressure and activity of the intrinsic laryngeal muscles [40]. Increasing subglottal pressure while holding fundamental frequency constant causes an increase in intensity, and increasing subglottal pressure while holding intensity constant causes an increase in fundamental frequency [41]. The amount of change to be expected has been calculated in modeling studies and found to be 0.5–6 Hz increase in frequency per cmH2O increase in subglottal pressure [42] or 8–9 dB increase in intensity per doubling of the phonation threshold pressure [40, 43]. Children have been found to have higher subglottic pressure than adults even when frequency and sound pressure level are controlled for [44–46].
Vocal Fold Length and Tension
Fundamental frequency is determined by mass and stiffness, which are related to vocal fold length and tension, and controlled by the relative contractions of the cricothyroid and thyroarytenoid muscles. These two muscles are innervated by different nerves (the cricothyroid by the SLN and the thyroarytenoid by the RLN), which allows for precise control of differential contraction and, thus, fundamental frequency. Stiffness is regulated by vocal fold length, proportional to vocal fold tension, and serves as the effective restoring force in vocal fold vibration [4]. Contraction of the cricothyroid muscle causes increased tension in the cover and body and consequent increase in fundamental frequency. Contraction of the thyroarytenoid muscle causes increased tension of the body which, depending on the state of cricothyroid muscle contraction, can either increase or decrease fundamental frequency [47, 48]. Isolated thyroarytenoid contraction causes a decrease in vocal fold length and cover stiffness with an increase in body stiffness, resulting in a decreased fundamental frequency. Combined contraction of the thyroarytenoid and cricothyroid muscles causes an increase in fundamental frequency, with isolated cricothyroid contraction causing the greatest increase in frequency.
Vocal Fold Contour

Glottic configurations, including convergent, divergent, and rectangular configurations. Image is courtesy of Dr. Erin Devine
Vocal Fold Adduction
Changes in prephonatory glottal width affect vocal aerodynamics and resultant voice quality, with increases in prephonatory glottal width leading to increased breathiness and decreased vocal efficiency. Increased prephonatory glottal width, as in vocal fold paresis or paralysis, leads to increases in phonation threshold flow and pressure [39, 51]. Flow tends to vary more than pressure with changes in glottal gap [52].
Hydration

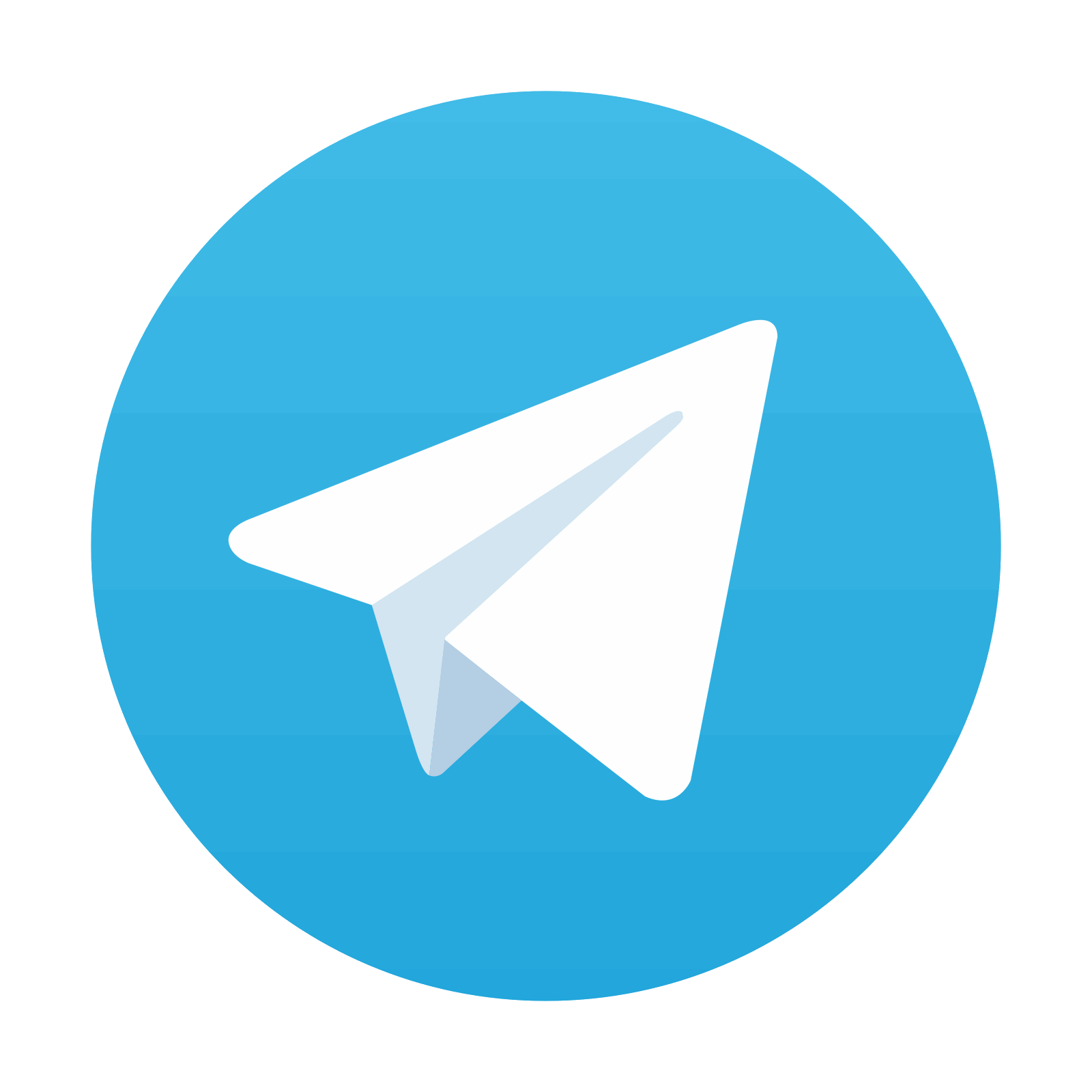
Stay updated, free articles. Join our Telegram channel

Full access? Get Clinical Tree
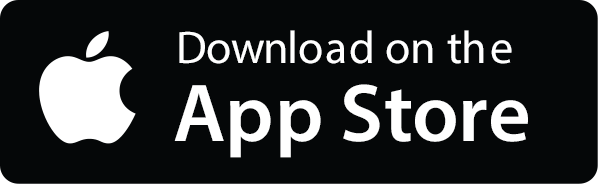
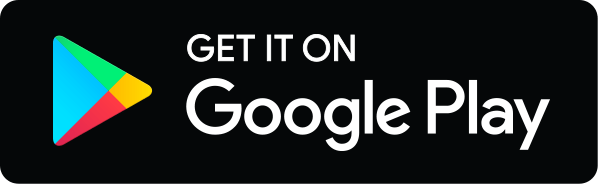