Fig. 12.1
Visualization of age-related and inflammatory changes in the vitreous using spectral domain optical coherence tomography (OCT). (a) OCT B-scan obtained from 74-year-old woman without uveitis demonstrating detachment of the posterior hyaloid face (arrows) and age-related vitreous condensation (arrowhead). (b) OCT B-scan obtained from a patient with birdshot chorioretinopathy demonstrating vitreous cells with minimal vitreous haze (clinical vitreous haze score +0.5, vitreous (VIT)/retinal pigment epithelium (RPE)-relative intensity 0.0783). (c) OCT B-scan obtained from a patient with sarcoid-related panuveitis demonstrating vitreous cells in combination with moderate vitreous haze (clinical vitreous haze score +1.0, VIT/RPE-relative intensity 0.2222). (d) OCT B-scan obtained from a patient with idiopathic panuveitis demonstrating vitreous cells in combination with moderate to severe vitreous haze (clinical vitreous haze score +2.0, VIT/RPE-relative intensity 0.2701). (e) OCT B-scan obtained from a patient with idiopathic panuveitis demonstrating vitreous cells in combination with more severe vitreous haze (clinical vitreous haze score +3.0, VIT/RPE-relative intensity 0.3818). Requires permission (from Keane et al., Ophthalmology 2014)

Fig. 12.2
Quantitative assessment of the vitreous using spectral domain optical coherence tomography (OCT) at initial presentation in a 57-year-old patient with idiopathic panuveitis (clinical vitreous haze score 2+). (a) OCT B-scan revealing widespread intravitreal cellular infiltrates and diffusely increased vitreous signal intensity. (b) OCT B-scan following manual segmentation of the vitreous compartment (boundaries: vitreous top to internal limiting membrane (ILM)) and the retinal pigment epithelium (RPE) (boundaries: RPE-inner to RPE-outer), with a resulting vitreous (VIT)/RPE-relative intensity of 0.3550. Requires permission (from Keane et al., Ophthalmology 2014)
Subsequent studies have validated this technique in a larger cohort using a different OCT device (Cirrus, Carl Zeiss Meditech) and have developed an automated version of the technique as part of its pathway to potential clinical adoption [17, 18].
Retinal and Choroidal Infiltrates
In many forms of uveitis, disease activity is characterized by features other than vitreous haze. For most posterior uveitis, the signs of active inflammation are to be found in the retina and choroid, being marked by new or growing inflammatory lesions which are presumed to be cellular infiltrates. Conventional fundus photography can be valuable in the documentation of these lesions and should not be underestimated. This is being extended through ultrawidefield imaging such as those provided by Optos (Optos Panoramic 200MA; Optos PLC, Dunfermline, Scotland, UK) [19]. This is of particular value in documenting lesions beyond the posterior pole such as acute retinal necrosis (ARN), retinal vasculitis , or intermediate uveitis [20, 21]. These types of imaging are useful in providing a permanent record of the extent of disease but are not routinely quantified and provide little information on the depth of the lesion or the tissue layers involved; such images also do not routinely provide quantitative data.
OCT can provide the three-dimensional imaging that enables accurate localization of depth of inflammation and its extent and is amenable to quantitative analysis. OCT does not need to be limited to the posterior pole. Extramacular OCT has been used in a targeted way for imaging extramacular lesions in infectious uveitis, notably toxoplasma retinochoroiditis and viral retinitis [22–25]. Keane et al. were the first to describe a systematic approach to extramacular enhanced depth OCT (EMEDOCT) which was utilized in a cohort of patients with birdshot chorioretinopathy [26]. This study depended on a five-position protocol where the patient was required to fixate in different directions; however, advances in technology will expand the field of view with greater speed and convenience to the patient. For example, with the Heidelberg Spectralis imaging system , it is now possible to obtain simultaneous widefield OCT images (up to 16.5 mm in diameter) and 55° fundus imaging, using a “widefield-imaging” module. The advent of the next generation of swept-source OCT devices also offers considerable promise in this regard [5]. Swept source OCT devices use a tunable laser as their light source and thus offer the potential for greatly increased image acquisition speed. The first commercially available swept-source OCT device for retinal applications is the DRI OCT-1 (Topcon, Japan). This has an image acquisition speed of 100,000 A-scans per second. As a result, its standard volume scan covers an area of 12 × 9 mm in seconds (by comparison, the standard volume scan area for its predecessor, Topcon 3D-OCT, covers an area of only 6 × 6 mm). Prototype swept-source OCT devices have recently been described which offer image acquisition speeds an order of magnitude greater than this (e.g., >1 million A-scans per second) and covering up to a 100° retinal viewing angle [27, 28]. The implications of these impending technologies for the management of patients with uveitis are clear.
Retinovascular Leakage
The FDA/NEI Working Group on Clinical Endpoints in Ocular Inflammatory Disease identified “retinal vascular leakage involving vessels posterior to the equator” as one of the key features of inflammation in uveitis. It is important to note however that much that is of significance in uveitis may occur outside of the posterior pole [19]. Conventional fluorescein angiography is limited to a 60° field even with the use of photomontage techniques. More recently, the Staurenghi contact lens combined with the confocal scanning laser ophthalmoscope (SLO) enabled 150′ visualization in the hands of a skilled operator, but the major advance has been ultrawidefield fluorescein angiography (FA) with the Optos system which provides a much larger field of view—up to 200°—with relative ease [29, 30].
Ultrawidefield FA permits accurate assessment of peripheral vasculitis and ischemia permitting the documentation of peripheral retinal changes which may often be underestimated or even completely missed on clinical assessment and which are outside the range of conventional FA. A number of reports have demonstrated the value of qualitative assessment of uveitis by ultrawidefield FA, but more recently a quantitative approach was proposed by Karampelas et al. who provided numerical indices for capillary nonperfusion, vessel leakage, and active vasculitis in a series of 82 patients with uveitis [31–33]. This study also confirmed that macular edema could be reliably detected on ultrawidefield FA and that its correlation with foveal subfield thickness on OCT was similar to that seen with standard FA in other studies [34–36]. Peripheral ischemia correlated with neovascularization-related leakage, a finding also seen in ultrawidefield FA studies of non-uveitic retinovascular diseases such as central retinal vein occlusion (CRVO) and diabetic eye disease [37, 38]. Peripheral vascular leakage correlated with macular edema (Fig. 12.3), possibly indicating the widespread hyperpermeability effects of inflammatory molecules such as vascular endothelial growth factor. Other studies have also shown the value of ultrawidefield FA in uveitis, with some studies noting that access to peripheral images led to a management alteration in 10–48% of cases [21, 39].


Fig. 12.3
Ultrawidefield fluorescein angiography (FA) (Optos PLC, Dunfermline, Scotland, UK) in a patient with intermediate uveitis. Evidence of both peripheral retinal nonperfusion (especially temporally) and cystoid macular edema (CME) can clearly be seen. The peripheral retina is less clearly visualized at the superior and inferior poles, where the patient’s eyelashes can be seen to impinge on the image
One of the challenges of ultrawidefield FA is the dramatic distortion that occurs in peripheral elements of the image, leading to the potential for gross overestimation of areas of retinal pathology. For example, in montage ultrawidefield images, area measurements will have an error of ≥5-fold if one assumes each pixel to have an equal size on the retina [40]. Fortunately however, techniques have recently been developed to correct for these errors. These involve ray tracing with model optical systems, with the resulting information used to stereographically project ultrawidefield images (thus preserving shape and area measurements) [40, 41]. These models are theoretical, however, and it is not possible to assess their accuracy as retinal landmarks have no standard dimensions. This issue has recently been overcome in an innovative manner: Sagong et al. used the known dimensions of an implanted intraocular retinal prosthesis to act as an “intraocular ruler” [42]. They also compensated for variance produced by the axial length of the eye. Their resulting algorithm allows accurate measurements of peripheral retinal distances and areas obtained using ultrawidefield imaging. Furthermore, this approach is likely to be incorporated into the software of commercially available Optos ultrawidefield instruments in the near future.
Anterior Chamber Cells
The first applications of anterior segment OCT were primarily as an alternative method of imaging major structures, but higher resolution systems are enabling identification and even quantification of signs both of disease activity and damage, including anterior chamber cells and flare, keratic precipitates, iris granulomata, peripheral anterior synechiae, and other angle pathology. The application of anterior segment OCT to uveitis is discussed more fully elsewhere (see Chap. 7), but the key issue relevant to this discussion is around the detection and quantification of AC cells. Improvements in hardware, acquisition technique, and image processing have enabled increasingly convincing studies that demonstrate OCT-derived estimates of cell count in the anterior chamber [43] and may in the future even be able to define cell type [6, 44].
Cystoid Macular Edema
The detection of CME and its subsequent quantification were the earliest uses of OCT in uveitis. Although not accepted as a primary outcome measure by the FDA, trials where it is included alongside the “accepted” endpoints of vitreous haze and visual acuity demonstrate its greater sensitivity to detect an effect than these traditional endpoints [45, 46]. Its value in clinical practice is well established, but there are a number of caveats. First, unlike angiography, OCT records static structure not dynamic leakage. A number of studies have shown that although most cases of uveitic macular edema will have changes on both imaging techniques, it is possible to have macular leakage on FA without macular thickening on OCT and vice versa [35, 36, 47, 48]. In the Multicenter Uveitis Steroid Treatment (MUST) Trial, the agreement in diagnosis was moderate (k = 0.44), with macular leakage on FA present in 40% of cases free of macular thickening on OCT and thickening present in 34% of cases without leakage. The second caution regarding the use of OCT-measured macular edema as an endpoint is that there is considerable variation in how it is recorded and reported.
There is also considerable variation in methods for OCT quantification and reporting, including reporting as a binary outcome, i.e., present/absent based on whether central macular thickness (CMT) is greater or less than a prespecified threshold (which varies according to the trial) [45, 49]; the absolute change in CMT [50]; the percentage change in CMT [51]; the change in CMT as a log score (unpublished; clinicaltrials.gov reference NCT01314417); change in cystoid area and height [52]; and estimation of retained area of retinal tissue at the plexiform layer [53]. In addition there are many studies that assess the pattern of uveitic CME including the presence of cystoid spaces, presence of diffuse thickening, and presence of subretinal fluid [54–56]. As with the vitreous, studies have also begun to look at the OCT signal intensity of cystoid spaces, and subretinal fluid, from various etiologies [8–13]. Although this heterogeneity is frustrating when it comes to evidence synthesis, it is a reminder that there is far more information on an OCT scan than will be captured by a single number.
Clinical Significance and Future Directions
The development of objective imaging-based measures of inflammation will revolutionize the field of uveitis, both in routine clinical care and for clinical trials. Just as the availability of sequential CMT measurements enables easy assessment of macular edema over a period of time, so new applications of OCT will open up an era of reliable assessment of the other key parameters of inflammation.
There is no doubt as to the potential of these techniques to replace our current subjective clinical assessments. The challenge is to continue to optimize and validate them for routine clinical adoption. This includes testing the additional benefit of increased width, depth, and resolution provided by newer OCT devices or sampling strategies. Validation is essential both to demonstrate the reliability of these techniques and also to show that the measures obtained correlate with a clinically meaningful impact, whether of visual acuity or some other measure of visual function.
Clinical validation may be challenging, as clinically “obvious” worsening of disease (e.g., enlargement of a unifocal area of retinochoroiditis due to ocular toxoplasmosis) may not produce a detectable change in any measured aspect of visual function (visual acuity, visual field, contrast sensitivity, color vision, etc.). Such demonstration of a visual function correlate is less likely to be an issue for clinicians who are used to assessing and treating asymptomatic worsening of disease based on clinical appearance even in the absence of a direct functional correlate. Ophthalmologists are therefore likely to be willing to adopt these novel OCT techniques for many reasons: first, the technology is already in clinic and available; second, clinicians are familiar with it; third, the measurements may be cross-referenced with the images, enabling clinicians to visualize these changes and see that they are not artifactual; and fourth, clinicians have already made the transition from biomicroscopic “guesstimate” to OCT-derived measurement in one domain (macular edema).
One potential barrier to adoption is the need for automation of some of these techniques. With our collaborators, we have gone on to develop a pilot automated system, VITAN, which can achieve these measurements in a matter of seconds [17]. We predict that within the next 5 years, these commercial systems will also include automated quantification of vitreous, individual retinal and choroidal lesions (macular and extramacular) and retinovascular pathology (for FA/indocyanine green angiography and “OCT angiography”).
This pathway—development, optimization, validation, and adoption—requires the partnership of experts and stakeholders from across a range of fields. For this purpose, in 2013, EQUATOR—Extended OCT Quantification of Uveitis Activity for Trial Outcomes and Reporting—an international collaboration of experts with skills from the fields of clinical uveitis, OCT, image analysis, modeling, outcome methodology, trial design, and diagnostic device evaluation was founded (www.equator.vison).
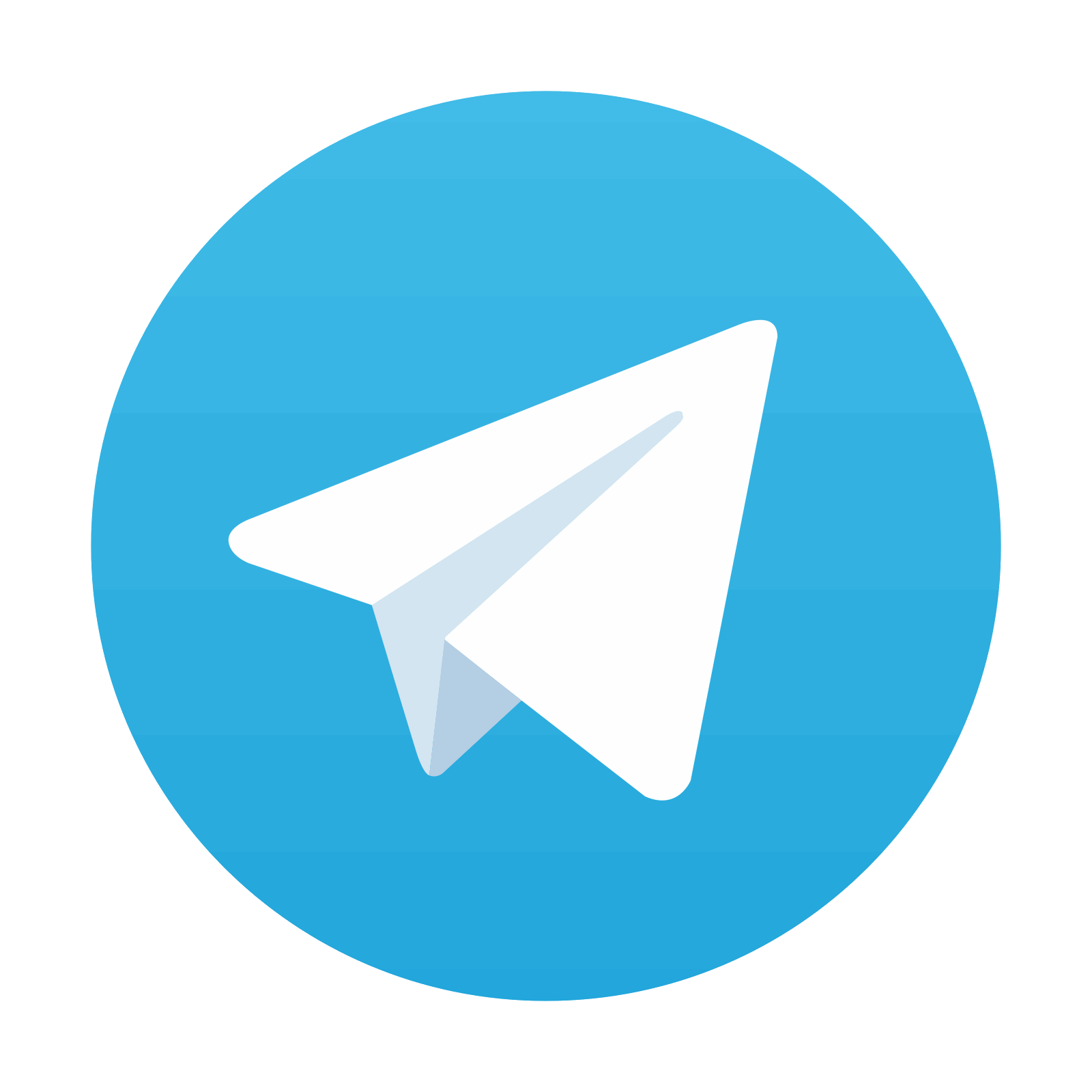
Stay updated, free articles. Join our Telegram channel

Full access? Get Clinical Tree
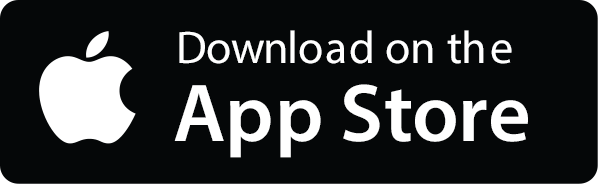
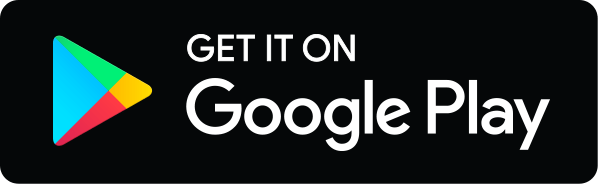