Migraine headache and Raynaud’s phenomenon
Vasospasm
Endothelins
C-reactive protein
Platelet aggregation, etc.
Vascular disease—carotid disease, ASCVD, MI, blood vessel, cholesterol, inflammation
Nocturnal hypotension
Vascular dysregulation
Autoimmunity
Shock syndromes/hypotension
Anemias
Diabetes mellitus
Obstructive sleep apnea syndrome
Definition
NTG, as discussed throughout this chapter, is a primary optic neuropathy with the following characteristics:
1.
Statistically normal range IOP < 22 mmHg on diurnal or multiple day measurements (some studies allow one IOP ≥22 and ≤24 mmHg).
2.
Optic disc cupping and associated retinal nerve fiber layer loss, often with flame-shaped, nerve fiber layer hemorrhages, similar to primary open-angle glaucoma with elevated intraocular pressures.
3.
Visual field defects consistent with the appearance of the optic nerve damage, typically nerve fiber bundle defects such as arcuate scotomas, nasal steps, or paracentral scotomas.
4.
An open anterior chamber angle without evidence of other causes of possible previously elevated IOP, such as peripheral anterior synechiae from prior uveitis or angle closure, angle recession from trauma, mid-peripheral, radial transillumination defects to suggest burned-out pigmentary glaucoma, or known history of steroid use that might have caused past elevated IOPs.
5.
Absence of other causes of visual field loss or optic nerve damage that could mimic glaucoma, such as congenital disc anomalies, a myopic tilted disc, optic nerve or pituitary/chiasmal tumors, previous arteritic or non-arteritic ischemic optic neuropathy, cardiogenic shock, or other non-reocurring vascular insult, where disc damage is typically nonprogressive.
Epidemiology
Population Studies
The IOP level at which the diagnosis of glaucomatous optic neuropathy has been made varies from the single digits to more than 50 mmHg. This modern understanding of the importance of IOP reduction for the treatment of all glaucomas is supported by many multicenter, randomized, prospective trials [4–10].
Many studies of NTG have concluded that the prevalence of NTG among glaucoma patients is high but variable, depending on the population studied (see Table 13.1). These wide variations in observed prevalence are undoubtedly due, in part, to varying definitions of NTG based on optic nerve and visual field criteria. Variations in prevalence may also not take diurnal IOP fluctuations or masqueraders of NTG fully into account.
Clinical Studies
In a large population-based study of almost 33,000 individuals in Malmo, Sweden (2002), NTG was identified as the most frequent glaucoma diagnosis (52.9 %) in newly diagnosed patients [11]. The detection of NTG was four times more common among unselected screened individuals (62 % of whom had previously seen an ophthalmologist) compared to self-selected patients identified from routine clinical practice. The authors concluded that NTG is easily overlooked.
NTG prevalence varies widely among different ethnic populations. In the Baltimore Eye Survey (1991), the prevalence of POAG ranged from 1.3 % with IOP 16–18 mmHg and 1.8 % with IOP from 19 to 21 mmHg up to as high as 26 % for IOPs ≥35 mmHg [12]. Half of white and black patients with POAG had a screening IOP less than 21 mmHg, decreasing to 25 % after one follow-up IOP and 15 % after multiple follow-up IOPs. The incidence of POAG increased steadily with IOP elevation in both groups, but 16.7 % of patients were ultimately diagnosed with NTG. In the recent Barbados Eye Study (2007) among Caribbean blacks of African ancestry, incident POAG was diagnosed in 4.4 % of all followed patients and incident NTG was seen in one-third of all newly diagnosed cases over a 9-year period [13].
In the Rotterdam Eye Study in Holland (1994) among whites, POAG prevalence was 1.1 % overall with 38.9 % of these diagnosed with NTG [14]. In the Egna-Neumarkt Study (1998) of mainly white individuals in Northern Italy, the prevalence of POAG was 1.4 % and NTG was 0.6 % [15]. In the Blue Mountains Eye Study (1996) in Australia among white individuals, the prevalence of POAG increased from 0.9 % with IOP 12–13 mmHg to 5.7 % with IOP 22–23 mmHg rising to 39 % for IOP ≥28 mmHg [16]. In the recent Thessaloniki Eye Study in Greece (2007) among all white individuals, the prevalence of POAG varied from 2.7 to 3.8 % depending upon the definition, but NTG with IOP ≤21 mmHg represented 59 % of those patients identified [17].
In the Los Angeles Latino Eye Study (2004) among Latinos of Mexican ancestry, the incidence of POAG was 4.4 % [18]. IOP ≤21 mmHg was seen in 82 % of these individuals. In the Tajimi Study in Japan (2004), overall POAG prevalence was 3.9 %, comprised of NTG with IOP ≤21 mmHg in 3.6 % and POAG with IOP ≥21 mmHg in 0.3 % [19]. In rural India (2005), prevalence of POAG was 1.6 % with NTG diagnosed in 67 % of identified patients [20]. Clearly, NTG is a very common condition worldwide.
Risk Factors for NTG
IOP-Related
Diurnal-Nocturnal Variation and Fluctuation of IOP
The Collaborative Normal-Tension Glaucoma Study (CNTGS) (1998) was a randomized trial of treatment comparing reduction of IOP by 30 % vs. observation in progressive NTG patients. It showed that aggressive IOP reduction could reduce progression of glaucomatous disease from 35 to 12 % during approximately 5 years of follow-up [5]. Also, we know that IOP fluctuates during the waking day (diurnal variation) and asleep at night (nocturnal variation) and from office visit to office visit (usually between 8 AM to 5 PM). So, is it possible that some patients with “apparent” NTG may have IOPs that are much higher outside clinic exam hours or have increased IOP in the supine position during sleep? These “high” IOPs that occur outside the office visit time frame need to be considered when deciding a patient meets the criteria for NTG vs. traditional HTG.
Several studies have demonstrated that IOP may peak when measured at times other than the usual time of office visits. Yamagami et al. showed in NTG patients that mean diurnal IOP variation was 4.9 mmHg and peak IOPs most often occurred at 10 AM, but that 55 % of peak IOPs occurred between 6 PM and 8 AM outside usual clinic hours [21]. In another study, however, these researchers found that peak 24-h IOPs could be predicted from an average of six clinic IOPs, suggesting that there may be value in checking the IOP in low-tension patients relatively often [22]. A third 24-h IOP study concluded that IOP variation in NTG patients was similar to normal subjects and that sleep had little influence on 24-h IOP variation [23]. Sequential, daylong, office IOP measurements have recently been shown useful to help identify selected NTG patients demonstrating visual field deterioration with supposedly “controlled” IOP. IOPs were all highest in the early morning hours in NTG, POAG, and glaucoma suspect patients. IOP variations >5 mmHg were seen in 35 % of subjects, and in the NTG group there was a significant correlation between visual field deterioration and IOP peak and range, suggesting the importance of these factors to predict progression in NTG [24]. Whether or not it is useful to check patients at night or in a recumbent position remains to be determined.
Patients with diurnal IOPs ≤15 mmHg have been more frequently associated with having retinal nerve fiber layer (RNFL) defects closer to fixation than in eyes with diurnal IOPs >15 mmHg [25]. The width of RNFL defects seen in this study, however, was not different between the two groups. These findings were interpreted by the authors to indirectly support an alternative pathogenesis for optic nerve damage other than direct IOP-related causation in NTG, such as optic nerve ischemia due to sectoral vascular insufficiency from small branches of the short posterior ciliary arteries supplying the laminar and prelaminar optic nerve.
Diurnal 24-h IOPs in NTG patients have also been observed to show a general decline overtime or in relation to patient age. In one prospective Japanese study, one-ninth of patients with NTG were observed to have declining untreated IOPs over 3 years [26]. Yet in another cross-sectional study of NTG patients after 4 weeks of medication washout, the mean, maximum, minimum, and IOP variation over 24 h did not differ between two patient groups divided by age <60 years vs. >60 years. But, 24-h IOP in this study correlated negatively with patient age >60 years [27].
Asymmetry of Disease and IOP
A number of studies during the past 25 years have demonstrated asymmetric optic nerve and visual field damage in some NTG patients, that is, the most affected eye has a higher IOP [28–31]. Note, however, that 24-h IOP in HTG eyes showed striking concordance of IOP variation between fellow eyes of 1.6–2.0 mmHg and the estimated probability of a 2-mmHg difference was 68–90 % and a 3-mmHg difference was 78–95 % for all time intervals studied [32]. More recently, these same researchers looked at the diurnal IOP fluctuation in untreated glaucoma suspect (GS) and NTG eyes [33]. They took 6 IOP measurements from 7 AM to 10 PM to evaluate the concordance of IOP between fellow eyes. The mean absolute IOP difference between eyes was 1.4–1.9 mmHg in the GS eyes and 1.3–1.5 mmHg for the NTG eyes (correlation between fellow NTG eyes, r = 0.81). The probability that the difference in IOP between fellow NTG eyes ranged within 3 mmHg was 86–93 %. These more recent studies bring into question the likelihood that true 24-h IOP asymmetry, which was not measured in the early studies, was the cause of the observed inter-eye disease asymmetry. As a result, one might postulate non-IOP-related factors, which were incorrectly attributed to the difference in measured daytime IOPs, to explain the observed asymmetry between fellow eyes of those NTG patients studied earlier.
Nocturnal, supine IOP rise may play a significant role in optic nerve damage seen clinically as “daytime” NTG. Intraocular pressure in the recumbent position is frequently elevated compared to the upright position. Even though we may never practically know how much our individual patient’s IOPs may rise during sleep, we can and should lower their daytime office IOPs with the most effective agents or interventions. We now know that prostaglandin analogues, laser trabeculoplasty, and surgical IOP lowering are the most effective interventions to lower daytime IOP and reduce 24-h fluctuations in IOP measured in the laboratory setting (see sections below on medical, laser, and surgical therapy for details). We should choose the best IOP-lowering therapy for each patient individually based on the stage of disease, demonstrated rate of progression, life expectancy, and willingness of the patient to engage in joint management of his or her own disease.
A number of studies have confirmed a thinner CCT (measured in μm by ultrasonic pachymetry) in NTG eyes vs. normal eyes vs. HTG eyes vs. OHT eyes, respectively (see Table 13.2). Aghaian et al. [34] found that CCT did not differ in normal eyes of Chinese, Caucasian, Hispanic, and Filipino individuals (548–555 μm), yet CCT was thinner in normal Japanese eyes (531 μm), eyes of HTG and NTG patients (538 and 529 μm), and normal African-Americans (521 μm) [35]. African-American patients have similarly been found with relatively thin CCTs in other population studies. For example, investigators found a similar CCT in normal eyes of Caucasians, Asians, and Hispanics (~550 μm), but thinner corneas in African-Americans (535 μm) [36]. Finally, a recent study of 1,699 normal Latino eyes in Los Angeles found a normal CCT of 547 μm in the same range as normal Caucasian, Chinese, Hispanic, and Filipino eyes studied elsewhere [37].
Table 13.2
Elements of the medical and ocular exam for NTG
Medical/ocular history |
Migraine, Raynaud’s syndrome, cold hands and feet, Prinzmetal’s angina |
Patient age and associated morbidity/longevity |
Systemic nocturnal hypotension—overtreatment of HBP |
Visual acuity |
Snellen acuity |
Color vision testing |
Refractive error |
Intraocular pressure |
Techniques—GAT, dynamic contour tonometry, ocular response analyzer, pneumatonometer, Tono-Pen, rebound tonometer |
Confounding of CCT |
Positional changes—supine and yoga head stands in Sirsasana |
Diurnal and multiple day measurements, nocturnal |
Pachymetry for CCT |
Gonioscopy to R/O uveitis/PAS, trauma/angle recession, PG/pigment dispersion syndrome |
Iris/lens appearance to R/O PG, trauma, pseudoexfoliation |
Optic nerve appearance |
Size |
Cupping patterns—APON/focal ischemic, senile sclerotic/age-related atrophic, myopic glaucomatous, notching, sloping, excavation, laminar dots/pore visibility, pallor, vessel patterns |
Hemorrhages |
RNFL |
PPA |
Tilting document appearance—stereo photos, spectral domain OCT, GDx VCC, HRT3 |
Assess ON blood flow?—color Doppler, velocimetry, flowmetry, FA |
Risk factors for progression of ON damage—IOP fluctuation, ODH, migraine, female sex, age? |
Visual field |
SITA SAP—size III vs. V stimulus, 24–2/30-2 vs. 10–2 pattern |
Establish a good baseline |
Frequent follow-up, q 3–6 mos. |
Use 10–2 program if visual field defect (VFD) close to fixation |
Use 10–2/size V program if very advanced damage |
SITA SWAP |
FDT Matrix |
Assess risk factors for progression of VF damage |
MRI/CT ± contrast—indications |
Loss of central acuity not explained by clinical exam |
Disc pallor out of proportion to disc cupping |
Young age |
Hemianopic VFD not respecting the horizontal meridian but respecting the vertical midline, particularly in the contralateral eye |
Unilateral progression despite equal IOPs in both eyes |
Unilateral or asymmetrical dyschromatopsia |
Other possible tests, as indicated |
Cold challenge—nail bed capillaroscopy for vasospasm assessment |
24-h. BP monitoring |
Blood tests—CBC, VDRL, FTA, ANA, ESR, Chem 12, viscosity, CRP, complement C3 and 4, cholesterol, platelet aggregation, endothelins, B12, folate |
Carotid artery studies—Doppler U/S, MRA, angiogram |
General systemic medical examination for: |
Monoclonal gammopathy of undetermined significance |
Autoimmune disease |
Blood pressure regulation to avoid nocturnal hypotension |
Treatment of cardiovascular disease |
Treatment of anemia |
Treatment of arrhythmia |
Treatment of congestive heart failure |
Systemic immunosuppression therapy in selected individuals |
Follow-up |
Risk factor assessment |
Diurnal vs. repeated IOP measurements for baseline IOP and fluctuation |
Role of 24-h IOPs |
Good VF baseline |
Frequent disc exams for ODH |
Baseline ON photos and computerized imaging with periodic re-imaging |
Target IOP determination derived from studies with periodic reassessment |
Thin CCT was associated with localized retinal nerve fiber defects in NTG eyes [38], with increased visual field defects at initial diagnosis but not with progression in POAG eyes, with visual field progression in POAG eyes (529 vs. 547 μm for progressing vs. non-progressing eyes) [39], and with a smaller IOP reduction in response to topical ocular hypotensive medications in OHT eyes [40]. From all evidence, consideration of CCT and its role in the diagnosis and progression of glaucoma is very important, with a thin CCT definitely associated with glaucomatous optic nerve and visual field damage and even response to IOP-lowering therapy.
In summary, in my practice I measure CCT in all glaucoma suspects and patients. If CCT is very thin (e.g., less than 500 μm), with an IOP <22 mmHg after multiple office-hour IOP measurements, and I suspect or diagnose NTG based on disc damage and/or visual field defects, then I typically aim for a goal of at least a 30 % IOP lowering (as supported by the CNTGS findings) with perhaps 5–10 % more lowering tacked on as a clinical “safety factor” for the unknown underestimation of true IOP.
Much more work needs to be done to sort out the actual contribution of corneal factors as represented by CCT or eye tissue biomechanics to IOP estimation and optic nerve susceptibility to glaucoma damage.
To summarize the above studies:
1.
CCT does appear to be significantly reduced in many eyes with NTG.
2.
A thin CCT is a risk factor for the diagnosis and progression of glaucomatous disc and visual field damage.
Eyes with a thin CCT may have higher IOPs than presumed by GAT and not be true NTG eyes (but HTG eyes). It is also logically possible that a thin CCT represents an inherent structural abnormality associated with the damage seen in glaucomatous optic discs and reflects an underlying structural weakness of the supportive collagen in the lamina cribrosa. Thus, an inherent susceptibility to IOP-related damage occurs, even at normal IOP levels, which leads to loss of retinal ganglion cell axons over time. This of course then results in classic glaucomatous optic nerve cupping and visual field loss as seen in NTG [41, 42].
Different Measurement Techniques for a More “Accurate” IOP
A number of devices have been developed as alternatives to the GAT (see Table 13.3) to attempt to measure IOP in settings of abnormal, irregular, scarred, pathologic, and postsurgical corneas (e.g., nystagmus, corneal edema, posttraumatic keratoconus, Fuchs endothelial dystrophy, after corneal transplant, post-PRK, or post-LASIK). A more detailed discussion of these newer IOP measuring devices is found elsewhere in this book. Briefly, Pascal dynamic contour tonometry (DCT) has generally been found to be less affected by CCT than GAT, although not in all studies, and tends to measure IOP slightly higher than GAT. IOP measurements by pneumatonometer, Tono-Pen, and non-contact tonometry (NCT) all appear to be dependent on CCT, with the NCT as most dependent and the Tono-Pen least dependent on CCT. The ocular response analyzer (ORA) seems to measure IOP independent of the effect of CCT and higher than GAT, while rebound tonometry (RBT) measurements correlate with GAT and appear to be affected by CCT. The pressure phosphene tonometer has a wide variability in its ability to measure IOP accurately due to its very subjective end point in IOP determination. For the time being, the GAT still remains the IOP measurement device standard, and it remains to be seen if future work may replace it with a newer device. As a practical matter, clinicians should check the calibration of their Goldmann tonometers monthly to avoid introducing additional measurement variability into inherent physiological variability of IOP [47, 48].
Table 13.3
Summary of results from the CNTGS reports
1. | 1992: 30 % IOP reduction from a mean of 16.9 mmHg was achieved in 57 % of NTG patients without surgery [43] |
2. | 1994: Early errors occurred in diagnosis of VF progression in NTG—more testing to confirm progression (in 4/5 repeated VF tests) reduced false-positive rate from 57 to 2 % [44] |
3. | 1998: Comparison of VF progression between groups—reduction in rate for treated (12 %) vs. untreated (35 %) NTG patients when corrected for cataract (mostly seen in trabeculectomy eyes, 16/34) [4] |
4. | 1998: Effectiveness of IOP reduction in NTG—VF progression seen in 20 % of treated patients vs. 60 % of untreated patients [5] |
5. | 2001: Natural history of untreated NTG—30 % show small, localized progression by 3 years and 50 % show small, localized progression by 7 years, not affecting the MD, but some progress faster [131] |
6. | 2001: Factors increasing relative risk for progression of VFDs in NTG—migraine = 2.58, disc hemorrhage = 2.72, female gender = 1.85, Asians < Whites [45] |
7. | 2003: Factors that predict the benefit of IOP lowering in NTG—no ODH, female gender, family history of glaucoma, no family history of stroke, no personal history of CVD, mild disc excavation, females (but not all) with migraine [46] |
Effect of LASIK/PRK on IOP
The recent popularity of corneal refractive surgery such as photorefractive keratectomy (PRK) and laser-assisted in situ keratomileusis (LASIK) has impacted the ophthalmologist caring for glaucoma patients. The corneal thinning resulting from these procedures results in underestimates of true, manometric IOP when using GAT. This may result in a generation of the overdiagnosis of NTG or underdiagnosis of true HTG in many patients if the examiner is either unaware of the corneal surgery or does not account for it when measuring IOP. Generally, IOP measurements by GAT and NCT [49] are 2–5 mmHg lower after PRK/LASIK, depending on the amount of refractive correction achieved [50–52]. IOPs measured by the Tono-Pen [53] and pneumatonometer [54, 55] appear less affected by refractive thinning. Measurement of IOP on the nasal, non-affected cornea has been advocated to lessen the measurement inaccuracy [56]. Recent studies have suggested that IOP readings by the Pascal DCT are not affected by PRK/LASIK corneal thinning [57–59]. However, IOP measurements by the ORA are significantly reduced, with associated decreases in corneal hysteresis and corneal resistance factor, suggesting that LASIK permanently alters the viscoelastic properties of the cornea [59]. Finally, several correction algorithms for IOP following LASIK have been derived. These formulas may be considered when preoperative information about an individual patient is missing [60–64].
In summary, the best and most pragmatic advice at this time is to obtain good baseline, office-hour IOP measurements before anticipated refractive surgery and then again, several months after surgery, when the patient is off all topical steroids. The difference in the average pre- and postoperative IOPs would be the patient’s individual IOP correction factor. In the absence of preoperative data, perhaps one of the previously cited correction algorithms could be utilized.
Optic Nerve Related
Optic Disc Size
The optic nerve transmits to the brain the retinal ganglion cell axons gathered from a total retinal area of more than 1,000 mm2 concentrated down into an area of 2–3 mm2. Although anatomically small in size, the optic disc is the key intraocular structure we study to help discriminate normal from abnormal with respect to diagnosing and following glaucoma patients [65] (Fig. 13.1).The normal optic nerve has about 970,000 ± 240,000 axons with a normal rate of age-related loss of about 4,900 axons per year [66]. The number of axons in the optic nerve is fairly constant from person to person despite varying sizes of the scleral canal outlet. There is no significant correlation between axon count and size of the scleral canal, which ranges from 1.6 to 2.9 mm2 in pathology specimens of normal eyes [67]. Clinically, optic disc area can range in extremes from 0.8 to 6 mm2 [68]. Some have suggested that a larger optic disc is more susceptible to IOP-induced damage.
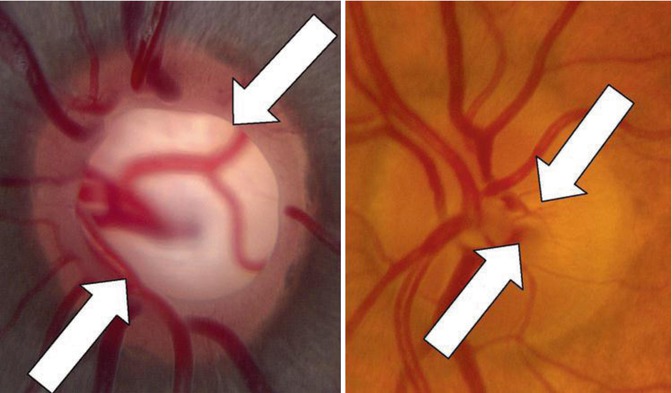
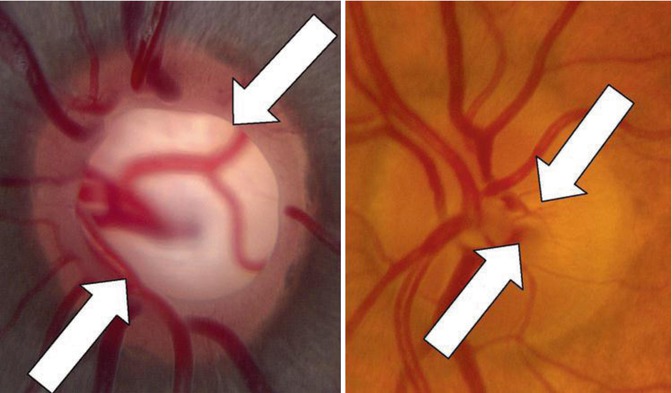
Fig. 13.1
Two normal but differently sized optic discs with different cup-to-disc ratios (between the white arrows in Fig. 13.2). Note the large disc on the left with C/D ~0.6 in a 14-year-old African–American male, and the small disc with C/D ~0.2 in a 47-year-old Caucasian female. Both patients had normal RNFL thicknesses by OCT and normal visual fields
Are myopia and optic disc size associated with NTG? In 1972, Tomlinson and Leighton compared the eyes of 11 NTG patients matched with respect to sex, age, and refractive with non-glaucomatous persons and HTG patients [68]. They found NTG eyes to have a longer overall axial length, with an increased cup-to-disc ratio (C/D), compared to normal and HTG eyes, although axial lengths and C/Ds did not differ between groups. Tomita et al. used computerized image analysis to study the optic nerves in 26 NTG patients [69]. They found a larger optic disc area and a higher mean IOP, associated with greater visual field loss, in 8 of 22 patients with disc size and IOP asymmetry, suggesting a greater vulnerability to IOP in eyes with larger discs. However, in 1995, Jonas et al. did not find an association between disc size, measured by morphometry, and visual field loss in NTG patients but noted more cupping and visual field loss in eyes with the smaller disc, suggesting a selection bias in the earlier studies [70].
Comparisons of High and Normal-Tension Glaucoma Discs
In his comprehensive article on NTG in 1980, Levene reviewed a total of 767 NTG cases [3]. He concluded that certain characteristics were more common in NTG than HTG. These features were:
1.
Early, dense visual field defects extending to within 5° of fixation
2.
Sudden visual field loss
3.
Early involvement of fixation
4.
Slow progression of the field defect
5.
A disproportion between the marked disc cupping noted and the limited but centrally located, detectable visual field defects
6.
High frequency of monocular cases
7.
Female preponderance
8.
Limited “sensitivity” of the field defect to even a marked reduction in IOP
Have these findings held up in studies subsequent to Levene’s review? The finding of greater disc cupping associated with less generalized loss of visual field mean sensitivity, but a deeper central visual field loss was confirmed by Gramer et al. [71]. but not by Lewis et al. [72]. Caprioli and Spaeth examined eyes with early to moderate visual field loss and found the optic disc rim in NTG eyes to be significantly thinner inferiorly and inferotemporally than in HTG eyes with an average IOP 15 mmHg higher than the NTG eyes [73]. But they did not find comparative differences in disc pallor, peripapillary atrophy, disc hemorrhage, nasal cupping vessel overpass, and baring of the circumlinear vessels between groups. Cup contour was judged to be more gradually sloped toward the rim in NTG than HTG eyes, but HTG eyes had a steeper cup wall farther from the disc edge than in NTG. They also found initial visual field defects to be closer to fixation in NTG and more peripheral, but progressing centrally, in HTG. They felt their findings implied a different pathogenesis of optic nerve damage in these two groups, with NTG eyes more susceptible to vascular optic nerve injury and HTG eyes more susceptible to barotrauma, rather than vascular injury. These findings were confirmed by Heidelberg retinal tomography (HRT) in a study by Eid et al. who found larger cup areas and C/D ratios, smaller inferior neuroretinal rim area, and a greater mean deviation of the superior rather than inferior arcuate visual field zone in NTG eyes, compared with HTG eyes [74]. They felt this indicated more vulnerability of the optic nerve to focal damage in NTG eyes. By contrast, Lester and Mikelberg [75] and Lester [76] did not find any differences in the optic nerve parameters measured by HRT between NTG and HTG eyes.
Others have compared the optic nerves of NTG and HTG patients. Tezel et al. found only increased disc hemorrhages and arteriolar narrowing in NTG eyes [77]. Wang et al. [78] found only an increase in peripapillary atrophy in NTG eyes with IOP ≤15 mmHg, while Miller and Quigley [79] found no differences between the two groups, except that an “hour glass appearance” of connective tissue bundles within the scleral lamina cribrosa was less common in NTG eyes. Miller and Quigley proposed that inherent, underlying differences in optic nerve head architecture of the lamina cribrosa that they observed might explain the variable sensitivity to IOP between HTG and NTG eyes.
Patterns of Optic Nerve Damage Observed in NTG Eyes
Attempts have been made to discriminate NTG from HTG based on observed patterns of optic disc cupping (focal vs. diffuse enlargement), disc hemorrhage occurrence, pattern of loss of the neuroretinal rim, amount of peripapillary atrophy, amount and appearance of retinal nerve fiber layer atrophy (localized vs. diffuse), and myopic tilting of the nerve (Figs. 13.2, 13.3, 13.4, 13.5, and 13.6). Spaeth has written that “LTG is not a valid clinical entity, just as HTG is not a valid clinical entity. The two overlap. The factor distinguishing LTG from HTG appears to be, paradoxically, not IOP but disk resistance.” [80] Yet, he and others have studied the glaucomatous disc with a view toward disease classification in an attempt to explain the pathogenesis of glaucoma by the various appearances of the disc seen on clinical exam. These researchers have carefully evaluated many glaucomatous optic nerves and discriminated four types of disc appearance, although none is exclusively associated with NTG:
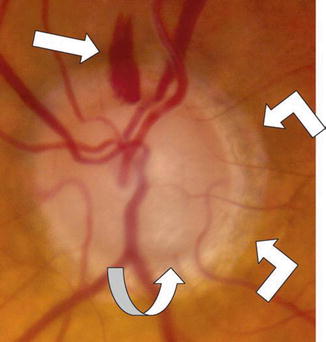
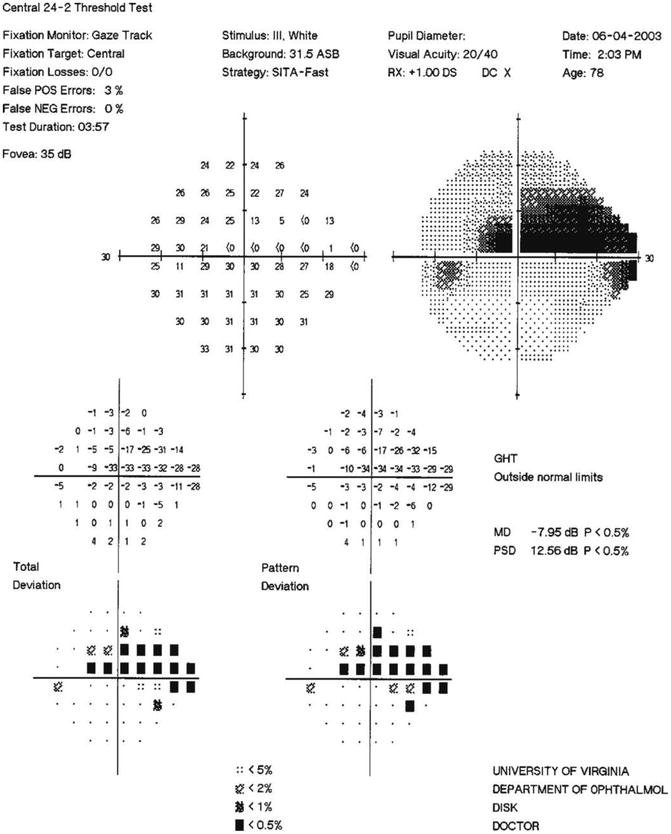
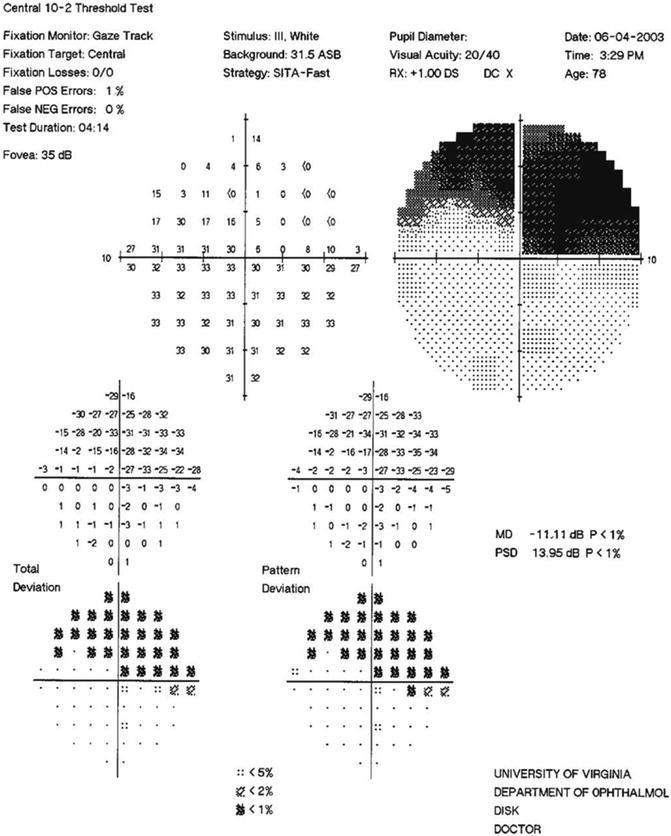
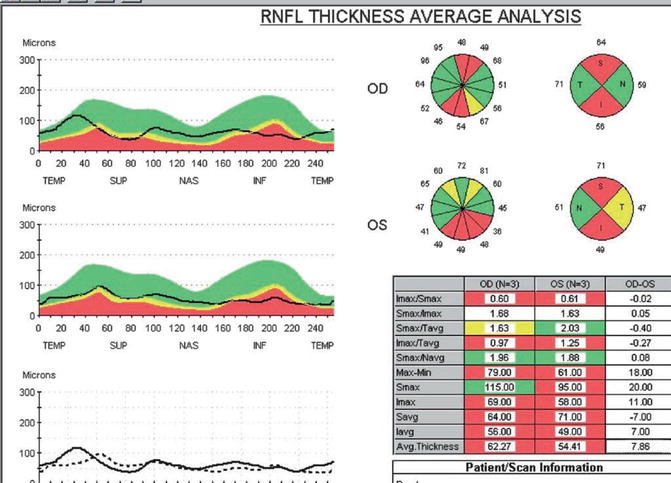
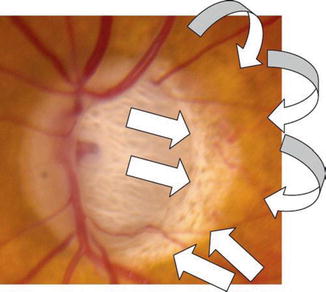
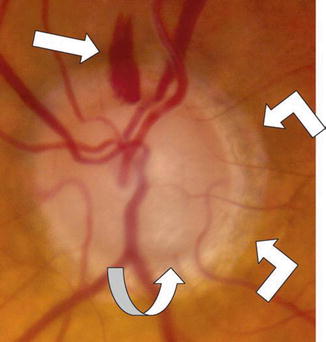
Fig. 13.2
Color disc photograph of an optic disc hemorrhage, acquired pit of the optic nerve, and peripapillary atrophy in the left eye of an 84-year-old Caucasian male with very advanced NTG. A typical, flame-shaped, optic disc hemorrhage (ODH, straight white arrow) seen at the 12 o’clock position in the left eye. Note that this hemorrhage has occurred in an area of thinned, but relatively retained, neural rim at the superior pole of the disc (vertical cup/disc = 0.9). By contrast, note the loss of the inferior neural rim at the 5–6 o’clock position, with focal deep excavation (an acquired pit of the optic nerve, APON, curved white arrow), associated focal pallor and visible lamina cribrosa pores deep in the cup. Note also typical, mild, β-zone peripapillary atrophy (angled white arrows) temporally and inferiorly beyond the disc margin
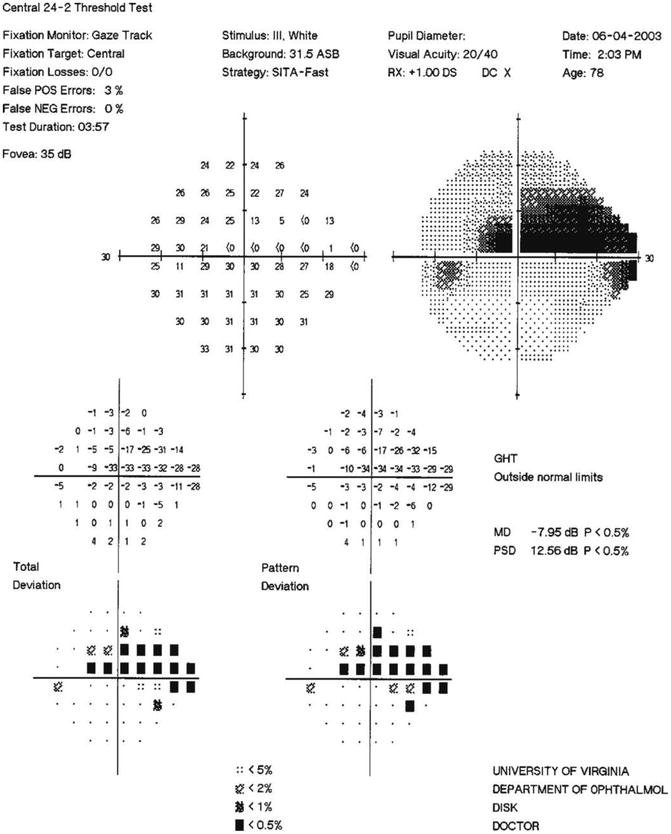
Fig. 13.3
SITA standard 24–2 visual field of the NTG patient in Fig. 13.2. Note the dense superior arcuate scotoma (overall MD = −7.95), encroaching on the point 3° superonasal to fixation (−34 dB), in this 24–2 visual field with 6° spacing of test points. This defect corresponds to the extensive loss of the inferior nerve rim. Note also that despite the significant cupping of the optic disc seen in Fig. 13.2, the inferior visual field only has an inferonasal scotoma, corresponding to the thin superior neural rim area where the dense ODH is located. Future follow-up will need to look carefully for changes in this region. The single, dense paracentral defect is expanded in the10–2 field, with 2° spacing of test points, shown in Fig. 13.4
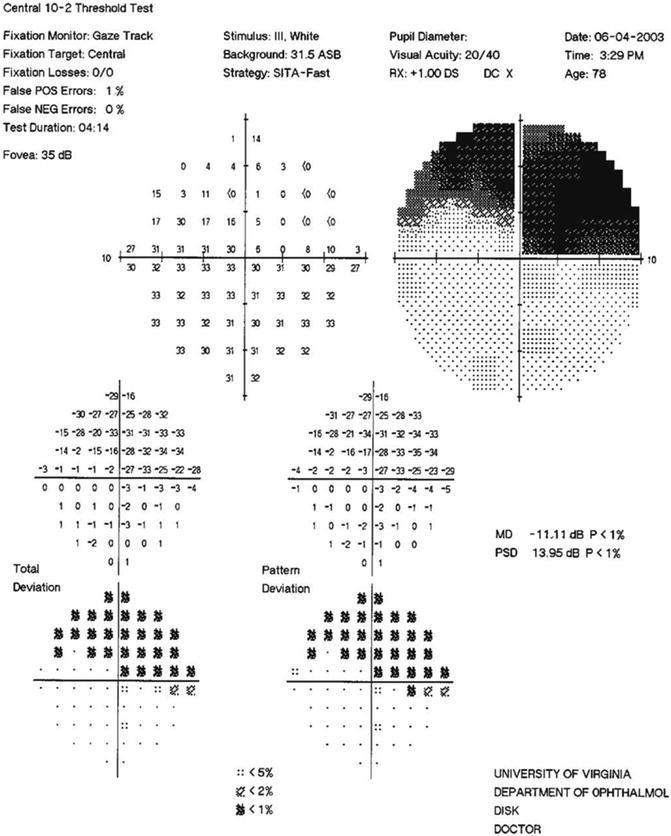
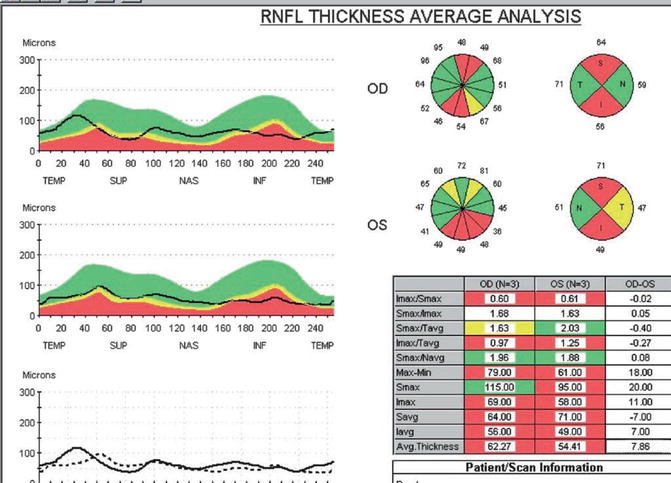
Fig. 13.5
Optical coherence tomography of the peripapillary RNFL of the NTG patient in Figs. 13.2, 13.3, 13.4, and 13.5. The 12 o’clock sector OCT NFL thickness OS is still normal at 72 μm, compared to a markedly thinned NFL of 49 μm at the 6 o’clock sector OS (seen in the 12 o’clock-hour pie pictograph, second from the top in the middle of the OCT printout). The patient’s superior OCT NFL thickness OS (represented by the black line in the second TSNIT graph on the center left) is still in the green normal zone at 12 o’clock, but dips into the borderline yellow zone in the neighboring 11 and 1 o’clock sectors (seen in both the TSNIT graph and the pie pictograph). OCT here appears to detect early NFL loss in the two sectors flanking the 12 o’clock ODH and suggests the need for careful follow-up for future progressive thinning of the NFL in this region
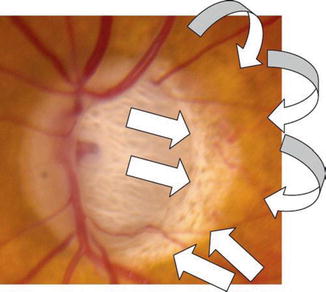
Fig. 13.6
Color disc photograph of typical β-zone (white crescent of atrophy adjacent to the temporal edge of the disc, straight arrows) and a-zone (mottled area outside the area of alpha atrophy, curved arrows) peripapillary atrophy in the left eye of an 88-year-old female patient with extremely advanced NTG (C/D 0.95+). Note also the marked disc cupping, laminar pores visible in the depth of the cup, and loss of both superior and inferior disc neural rims
2.
Senile sclerotic glaucoma (“age-related atrophy”) discs often associated with NTG, first described by Geijssen and Greve in 1987 [81]
4.
Generalized enlargement of the optic cup discs associated with diffuse retinal nerve fiber layer atrophy, usually not associated with NTG, and described by Spaeth [82] and many other authors
Focal Ischemic Discs
Spaeth first described “primary ischemic glaucoma,” later termed “focal ischemic glaucoma,” based on fluorescein angiography studies of the optic nerve in 16 patients with clinical NTG [82]. These eyes were characterized by inferotemporal disc hypofluorescence, shallow and eccentric disc cupping with more pallor than expected, associated disc hemorrhages, and dense, often absolute, superior, paracentral scotomas frequently noted by the patient. He did not comment on the amount of cupping compared to the visual field. In the NTG patients with this focally damaged disc appearance, he found a possible association with diabetes mellitus, syphilis, severe heart disease, “hemodynamic crises,” and platelet abnormalities, suggesting localized ischemia of the optic nerve head to explain the disc injury.
Subsequent studies [83–90] have all confirmed that the focal ischemic disc appearance, also termed “acquired pit of the optic nerve,” [85] is seen also in HTG but more frequently in NTG (~15 % vs. 75 %, respectively [85]). These studies have revealed a number of associations with the focal ischemic disc.
Table 13.4
Possible surgical procedures to consider in NTG eyes
Trabeculectomy with or without 5-FU or MMC |
Trabeculectomy with express shunt |
Glaucoma drainage implants |
Non-penetrating surgery |
Viscocanalostomy |
Non-penetrating deep sclerectomy with collagen implant |
Trabeculotomy |
Trabectome |
Canaloplasty/trabecular tightening |
IStent trabecular micro-bypass stent |
SOLX suprachoroidal shunt |
Cyclophotocoagulation |
Non-contact |
Contact—diode G probe |
Endocyclophotocoagulation with phaco |
Cataract surgery alone with potential post-op IOP decline |
In particular, the development of the optic pit has been speculated to arise from an underlying “laminar insufficiency” [85] due to a primary weakness of the optic nerve, whether from structural, vascular, or other etiologies. This theory is supported by pathologic findings from work by Quigley and coworkers who found a number of structural differences in the lamina cribrosa of patients with NTG compared to HTG [91, 92].
Senile Sclerotic Discs
Geijssen and Greve first described “senile sclerotic glaucoma” characterized by older age, relatively low IOP (or NTG), normal anterior chamber angles, “senile excavation” or a saucerized cup with gradual sloping of the optic nerve cup toward the rim, atrophy of the peripapillary retina, and choroidal sclerosis (also called “fundus tessellation”) giving the disc a “moth-eaten” appearance [81]. HTG eyes, by contrast, had younger age, high IOP, abnormal anterior chamber angles, deep and steep optic nerve excavation, little peripapillary atrophy, and no choroidal sclerosis. They found larger superior than inferior visual field defects in the NTG eyes compared with equal superior and inferior visual field defects in HTG eyes. They suggested a primary local vascular disease with chronic ischemia causing damage in these senile sclerotic discs, leading to the appearance of peripapillary atrophy and choroidal sclerosis, and a primary IOP mechanism for damage in the HTG eyes, with a secondary role of insufficient blood supply causing disc injury in HTG. Subsequent studies have also found similar and additional characteristics in patients with senile sclerotic discs [83, 87, 93, 94].
Myopic Glaucomatous Discs
Geijssen first proposed that myopic eyes might represent a distinct group of NTG with a characteristic disc appearance when damaged by glaucoma, separate from damage due to pathologic myopia [84]. In her study, she observed disc tilting with oblique insertion, shallow cupping, temporal crescents or peripapillary atrophy, and typical glaucomatous thinning and excavation of the superior and inferior poles of the neuroretinal rim. Subsequent studies have confirmed these and other findings in this type of myopic glaucomatous disc damage [83, 87, 95, 96].
Generalized Enlargement of the Optic Cup Discs
Spaeth first called this type of optic nerve damage “primary hyperbaric glaucoma” characterized by elevated IOP, a normal disc fluorescein pattern, more symmetric, round enlargement of the optic cup, and visual field loss more often peripheral than central [80]. He proposed that disc damage in this group of eyes was purely mechanical in etiology from the chronic effects of elevated IOP. Other studies have also found a number of characteristics in patients with generalized optic cup enlargement [83, 87, 95, 96].
In summary, while these four types of disc cupping are often typical of eyes with NTG, they are not generally predominant in this disease entity. However, their identification may help target disease management from an ocular (e.g., degree of necessary IOP lowering) and systemic (e.g., treatment of cardiovascular risk factors) point of view.
Neuroretinal Rim (NRR) Loss
Jonas et al. examined the pressure dependence of NRR loss in patients with NTG [97]. They found that optic disc size actually decreased with decreasing maximal IOP in eyes with the focal ischemic disc appearance, suggesting a vasogenic reason for the nerve fiber loss. This finding was also suggested by another study that found an abnormal retrobulbar circulation in NTG eyes [98]. Yet, Jonas et al. also found a correlation between a smaller NRR area in eyes with a relatively higher IOP compared to eyes with a lower IOP in all three of the focal ischemic, senile sclerotic, and myopic glaucomatous disc types seen in the NTG eyes he studied [97]. This suggests a barotraumatic mechanism for damage in all these disc types characteristic of NTG.
Tan et al. utilized the HRT to study NRR loss in OHT, OHT converters, NTG suspects, and NTG eyes [99]. These researchers found reproducible loss of NRR primarily at the superior and inferior poles of the OHT groups, especially inferiorly, consistent with prior studies showing vertical cup elongation in early glaucoma. NRR loss was detected in 11 % of OHT suspects and in 90 % of OHT eyes converting to glaucoma. NRR loss was detected in 58 % of NTG suspects but in only 54 % of NTG eyes and in 3 of 5 NTG converters in whom visual field defects developed. Temporal rim loss in these eyes correlated with dense paracentral visual field defects, as seen in other studies previously mentioned.
Optic Disc Hemorrhage (ODH)
Optic disc hemorrhages are a hallmark of progressive glaucomatous optic nerve damage. ODHs were characterized by Drance et al. in the 1970s as localized ischemic optic neuropathy, and they noted a 20 % prevalence in NTG patients [99–102]. Since then, many studies have examined patients with NTG and ODHs to elucidate their characteristics and associations and to correlate ODHs with disease state.
Optic disc hemorrhages may be linear, flame-shaped, splinter-shaped, or occasionally blot type and rounded. They usually are found in the prelaminar area of the optic nerve and peripapillary retina, and rarely in the depth of the optic disc cup. ODHs are found with a higher frequency (~3:1) in the inferior vs. the superior disc pole of the disc. ODHs in NTG eyes have recently been found to be associated with branch retinal vein occlusions in the contralateral eye, suggesting a possible common microvascular thrombotic mechanism [103, 104].
Jonas and Budde investigated glaucomatous eyes with very high IOP (juvenile open-angle glaucoma) and very low IOP (NTG), in order to increase the possibility to detect differences in optic nerve appearance between the groups. In contrast to earlier studies, these authors found that the optic nerves in these two groups were similar in number of detectable localized retinal nerve fiber layer defects (RNFLDs), size of peripapillary atrophy, optic cup depth, steepness of disc cupping, rim/disc area ratio, retinal arteriole diameter, and degree of focal arteriolar narrowing. However, NTG eyes did have broader localized RNFLDs, more frequent NRR notches, and more frequent and larger ODHs [105]. They speculated that the lower frequency of detected ODH and NRR notches in high-pressure JOAG eyes resulted directly from the larger transmural pressure gradient across the small vessels of the optic disc in NTG eyes. Therefore, a small vessel rupture on the optic nerve in eyes with NTG would lead to a larger disc hemorrhage that reabsorbed more slowly, and hence have a higher chance to be detected in disc photos than in eyes with HTG. An ODH due to a similar vessel rupture in an HTG eye would be smaller, more easily reabsorbed, more easily missed in a disc photo, and lead to a smaller resulting RNFLD seen on subsequent clinical exam. Thus, the relative paucity of ODH in HTG vs. NTG may be an artifact of the examination which may not reflect the true frequency of occurrence.
Based on their findings, these researchers further speculated that the pathogenic mechanisms of disc damage in NTG and HTG may rather be more similar than different. This conclusion also agrees with:
In summary, ODHs serve as an easily identifiable sign of disease progression and should always be carefully searched for on clinical exam and review of disc photos. Their occurrence should prompt more investigation within 2–6 weeks into the status of a patient’s disease state such as:
1.
Optic disc photos and computerized disc imaging at current visit to document the ODH
2.
VF testing with 24–2 or even central 10–2 programs, if a paracentral defect is suspected based on the ODH location, to look for change in or new visual field defects
3.
More frequent IOP monitoring at various times throughout the day to detect unsuspected IOP spikes
4.
Usually more aggressive IOP control to reduce IOP an additional 15–20 % from established current baseline
5.
Repeat optic nerve evaluation with dilation to look for ODH resolution, new and/or recurrent ODHs
6.
Subsequent careful disease state monitoring with VF testing and optic nerve exam to establish future stability
Peripapillary Atrophy (PPA)
Peripapillary atrophy is separated into two zones: zone α, a peripheral zone with irregular hyper- and hypopigmentation from loss of the RPE, and zone β, a central, whitish zone with visible choroidal vessels and sclera due to loss of both RPE and photoreceptors (Figs. 13.6, 13.7, and 13.8). This atrophy is felt to develop due to loss of the peripapillary choroidal branches from the short posterior ciliary arteries, which penetrate centripetally into the prelaminar portion of the optic nerve head in a sectoral fashion. These branches supply the optic nerve axons and collagenous laminar beams with blood. Absence or dysfunction of these branches would cause segmental ischemia and damage to the optic nerve head leading to axonal loss, focal disc notching, cupping, and acquired pits of the optic nerve with associated PPA.
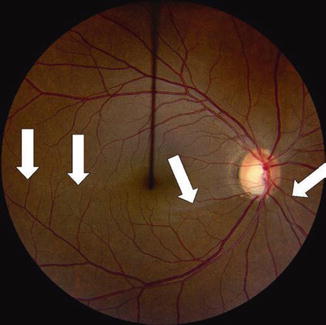
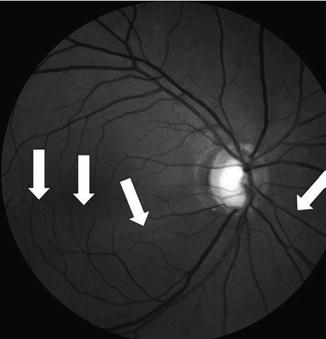
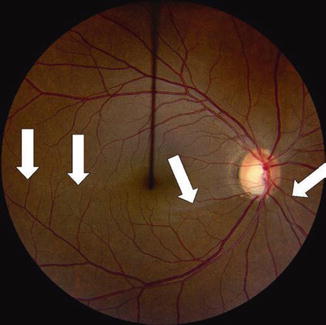
Fig. 13.7
Color disc photograph showing loss of the inferior NFL with focal cupping in the right eye of a 41-year-old Hispanic female with moderately advanced NTG, OD > OS. Classical, deep, inferior excavation/cupping and focal pallor of the right eye optic nerve rim with marked loss of the associated inferior arcuate NFL (vertical cup/disc = 0.75–0.8). Note the distinct loss of inferior NFL striations with absence of typical high NFL reflectance inferiorly and a resulting dull, dark, wedge-shaped defect from 4 to 8 o’clock, with clear and sharp visibility of the blood vessels and their margins in this region, as a result of the loss of the surrounding NFL (between white arrows). By contrast, these brightly reflected NFL striations are seen easily at the superior pole of the disc where the neural rim is well retained, with blurring of the blood vessel margins due to the normally thick NFL surrounding the vessels in this area
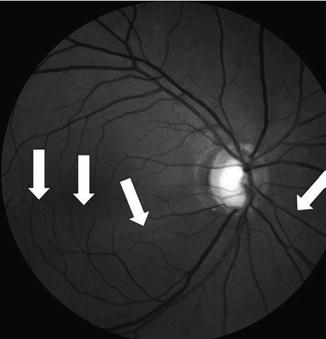
Fig. 13.8
Red-free NFL photograph of the right eye of the NTG patient in Fig. 13.7. Note the very dense loss of inferior NFL seen in the dark, wedge-shaped, extensive defect, radiating out from approximately the 4 o’clock to the 7:30–8 o’clock position on the nerve rim (between white arrows), seen also in Fig. 13.7
Anderson first suggested in 1983 that peripapillary halos or atrophy were more common in NTG than HTG eyes [106]. Geijssen and Greve in 1987 first described the association of PPA with larger superior than inferior visual field defects in the NTG senile sclerotic disc compared to little PPA and equal visual field defects in the superior and inferior regions in HTG discs [81]. They concluded that primary local optic disc microvascular insufficiency could explain the pathogenesis of disc damage in NTG eyes, while in HTG eyes elevated IOP played a primary role and vascular insufficiency a secondary role in the pathogenesis of disc damage. Their findings are similar to those by Kawano et al. in 2006, who found that only the inferior half of zone β PPA correlated with glaucomatous VF damage [107]. Further, inferior PPA also correlated with axial length, while superior PPA correlated only with axial length but not VF damage. They felt these findings suggested that the inferior region of the ONH is more vulnerable to damage in NTG than the superior region, and therefore, inferior PPA is more clinically significant in NTG than superior PPA.
Similarly, Buus and Anderson found the incidence of PPA to be 64 % in NTG discs and only 34 % in OHT discs [108]. This difference persisted even after exclusion of eyes with myopia greater than −4 diopters. Araie et al. also found that the extent of PPA in untreated NTG eyes correlated with VF progression [109]. But, Tezel et al. found that zone β PPA was detected more often in NTG than HTG eyes, presumably because the NTG eyes were seen at a more advanced stage of disease [77]. They felt that the final appearance of the optic nerve damage was similar in NTG and HTG eyes for the same degree of damage, regardless of the possible different mechanisms for the optic neuropathy observed in the two groups. This was confirmed by Jonas and Xu, who did not find larger areas of zone α or β PPA in NTG eyes compared to HTG eyes, after exclusion of eyes with myopia greater than -8 diopters, when eyes were matched for stage of disease by the amount of remaining NRR area and visual field loss [110].
In summary, β PPA is a sign associated with advancing glaucomatous optic neuropathy. Its presence should lead the clinician to look for other signs of related disc damage such as associated disc notching or rim thinning, disc hemorrhages, retinal nerve fiber layer loss, and prompt VF testing to detect possible early disease, where NTG has not necessarily been suspected previously.
Visual Field Defects (VFDs)
Patterns of Loss
Dense, Focal, and Central Versus Peripheral and Diffuse
As documented from review of the early classical literature on NTG and from his own retrospective case series, Levene [3] concluded that VFDs in NTG eyes tend to:
1.
Involve fixation early
2.
Be dense and to within 5° of fixation
3.
Occur suddenly
4.
Be disproportionately less extensive than expected from the advanced optic nerve damage observed
5.
Be monocular
6.
Progress slowly
In a retrospective review of NTG patients in 1976, Chumbley and Brubaker noted a nerve fiber bundle pattern of Goldmann VFDs in all eyes that could be evaluated [111]. Superior arcuate scotomas were seen in 84 % and inferior arcuate scotomas in 58 %, and both defects in 41 % of eyes (Figs. 13.3, 13.4, 13.9, 13.10, 13.11, 13.12, and 13.13). Interestingly, VFDs involved the macula in 25 % of eyes either at initial exam or progressed to involve the macula on follow-up in their study group.
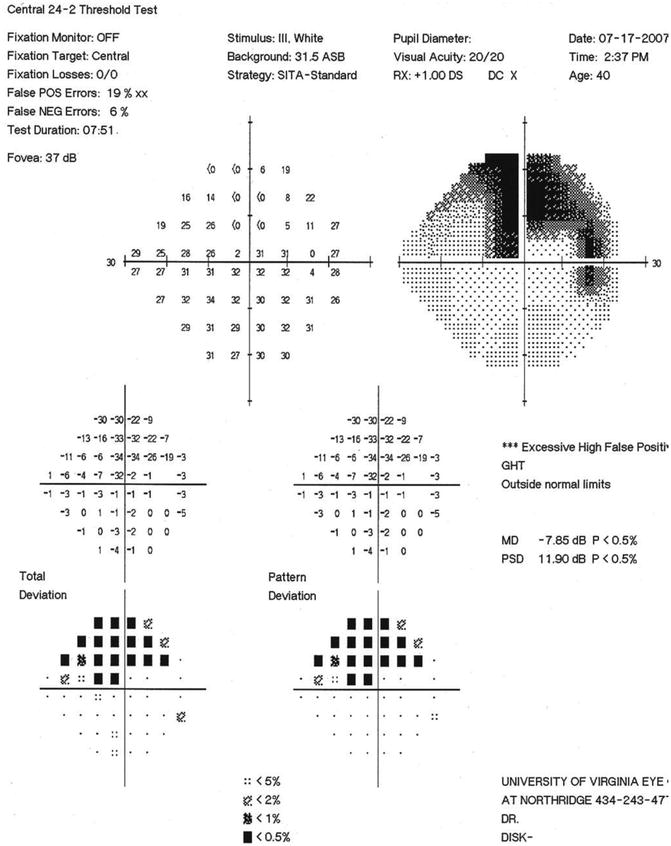
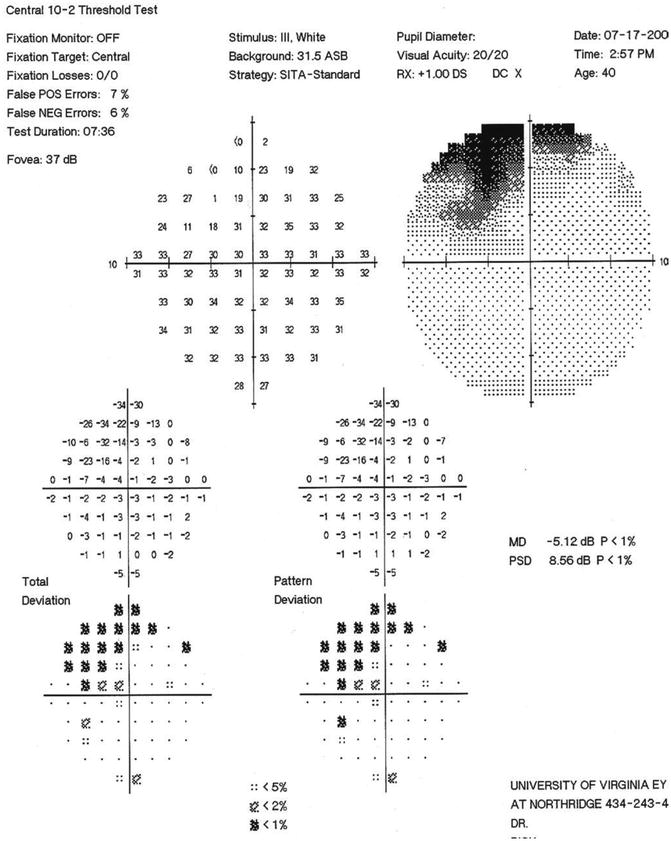
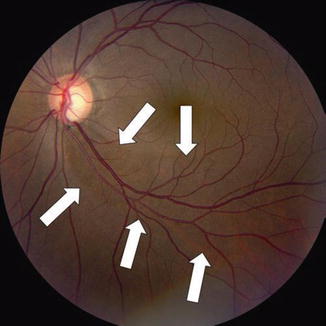
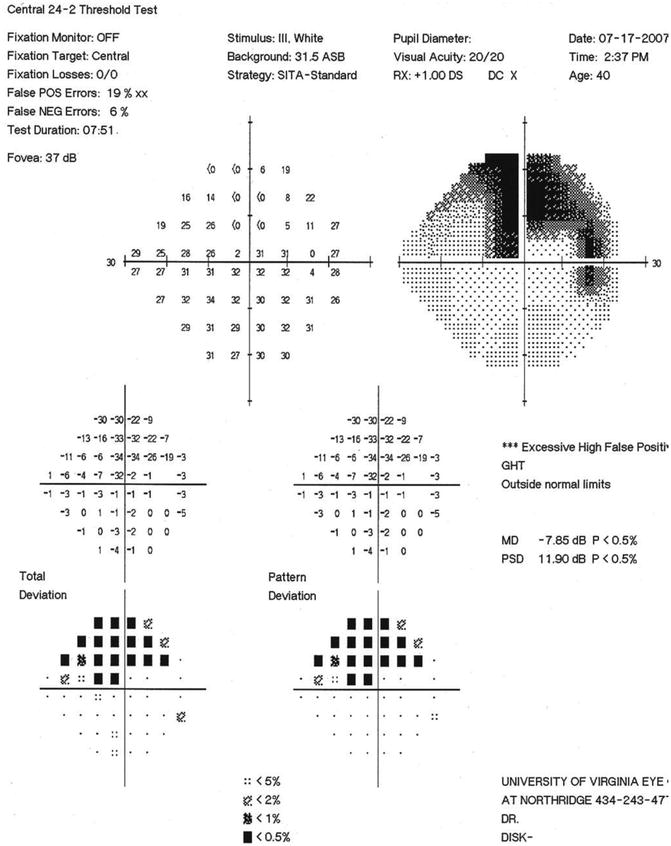
Fig. 13.9
SITA standard 24–2 visual field of the NTG patient in Figs. 13.7 and 13.8. Note the dense superior arcuate scotoma (overall MD = −7.85) and the dense superonasal paracentral defect (−32 dB) in the single point near fixation on this 24–2 field, with 6° spacing of test points. This single point defect is expanded in the 10–2 field, with 2° spacing of test points, shown in Fig. 13.10
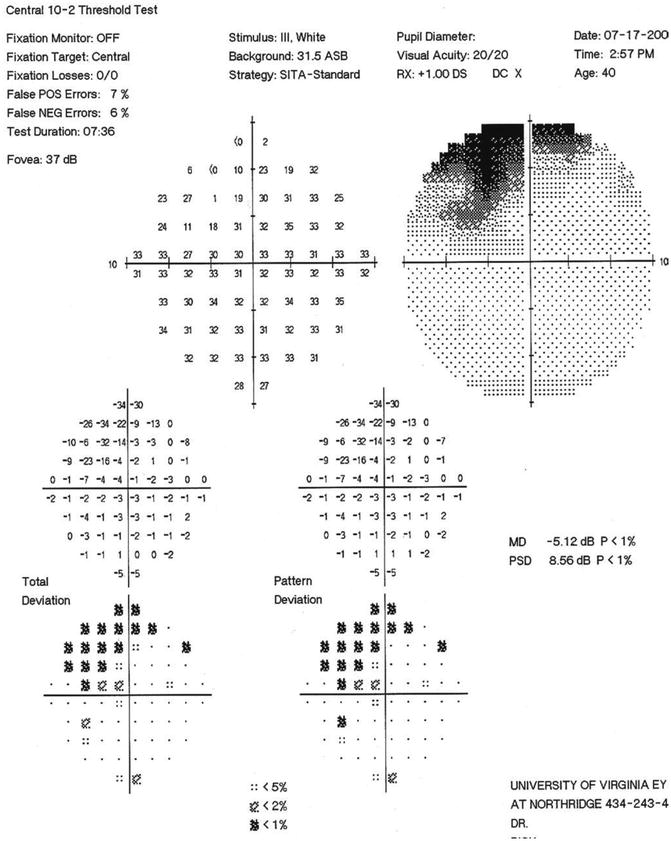
Fig. 13.10
SITA standard 10–2 visual field of the NTG patient in Figs. 13.7 and 13.8. Note the moderately dense superior arcuate scotoma extending to within 3° of fixation superonasally, with points of depression in this superonasal area ranging from −14 to −34 dB. This fairly extensive area of significant visual field involvement (overall MD = −5.12, involving 16 test points) is represented by only the single paracentral point with a MD = −32 in the 24–2 field in Fig. 13.9. Use of the 10–2 program can enhance detection of early paracentral visual field loss and can be used to effectively follow progression that might easily be missed on the 24–2 program
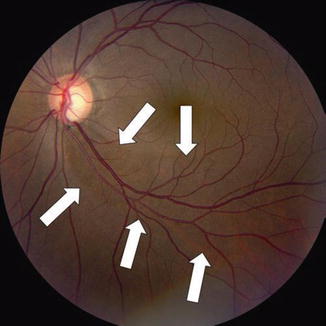
Fig. 13.11
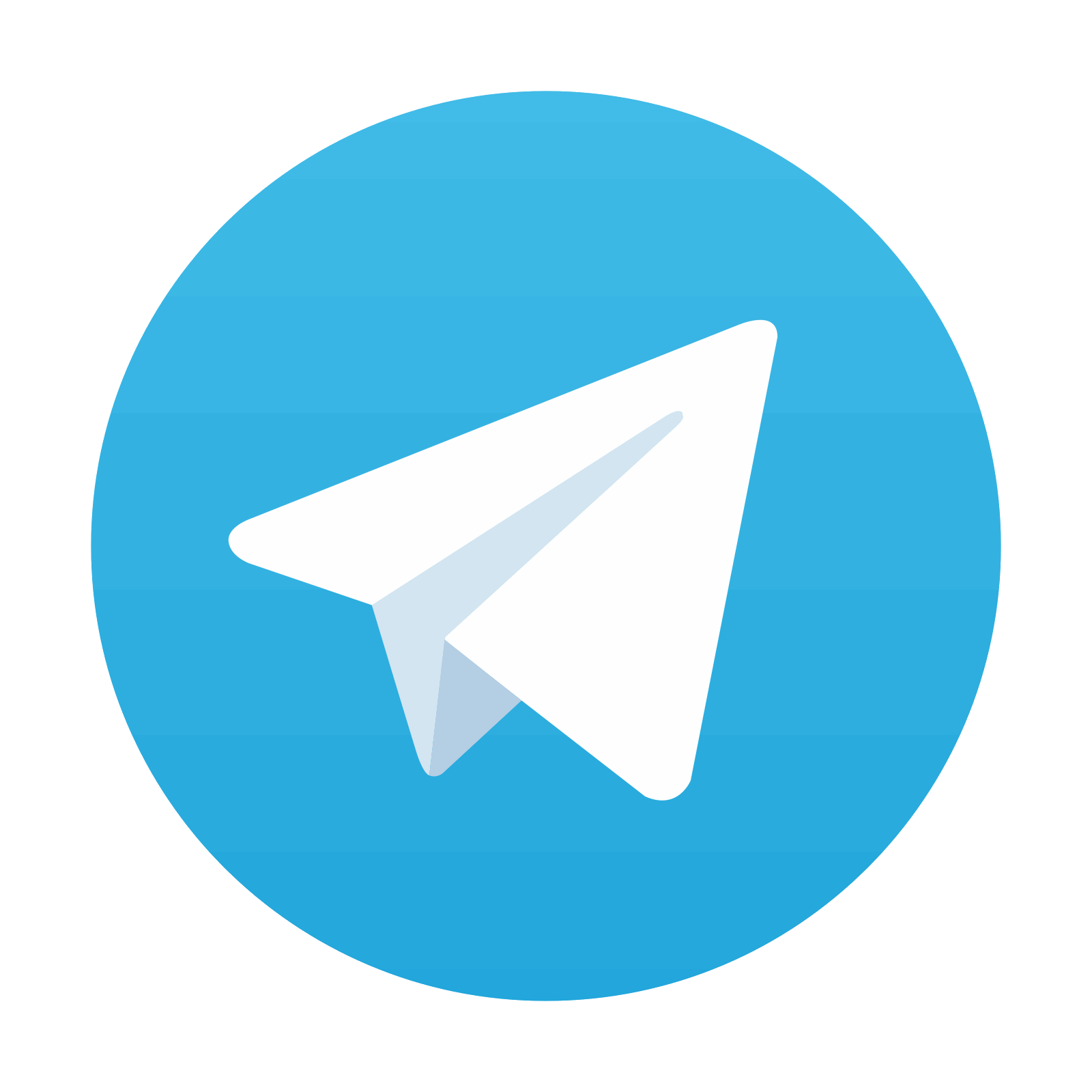
Color disc photograph showing loss of the inferior NFL with focal cupping in the left eye of the 41-year-old Hispanic female with moderately advanced NTG, OD > OS, seen in Figs. 13.7, 13.8, 13.9, and 13.10. Classical, deep, inferior excavation/cupping, and focal pallor of the left eye optic nerve rim with marked loss of the associated inferior arcuate NFL (vertical cup/disc = 0.75–0.8). Note the smaller, wedge-shaped, NFL defect radiating out from approximately 4:30–5 o’clock to the 6 o’clock position on the nerve rim (between white arrows), compared with the defect OD seen in Figs. 13.7 and 13.8. Note again the distinct loss of inferior NFL striations with absence of typical high NFL reflectance inferiorly and a resulting dull, dark, wedge-shaped defect from 4:30–5 o’clock to 6 o’clock. The blood vessels and their margins in this inferior region are clear and sharp, as a result of the loss of the surrounding NFL. By contrast, the brightly reflected NFL striations are seen easily at the superior pole of the disc, where the neural rim is well retained, resulting in blurring of the blood vessel margins due to the normally thick NFL surrounding the vessels in this superior area
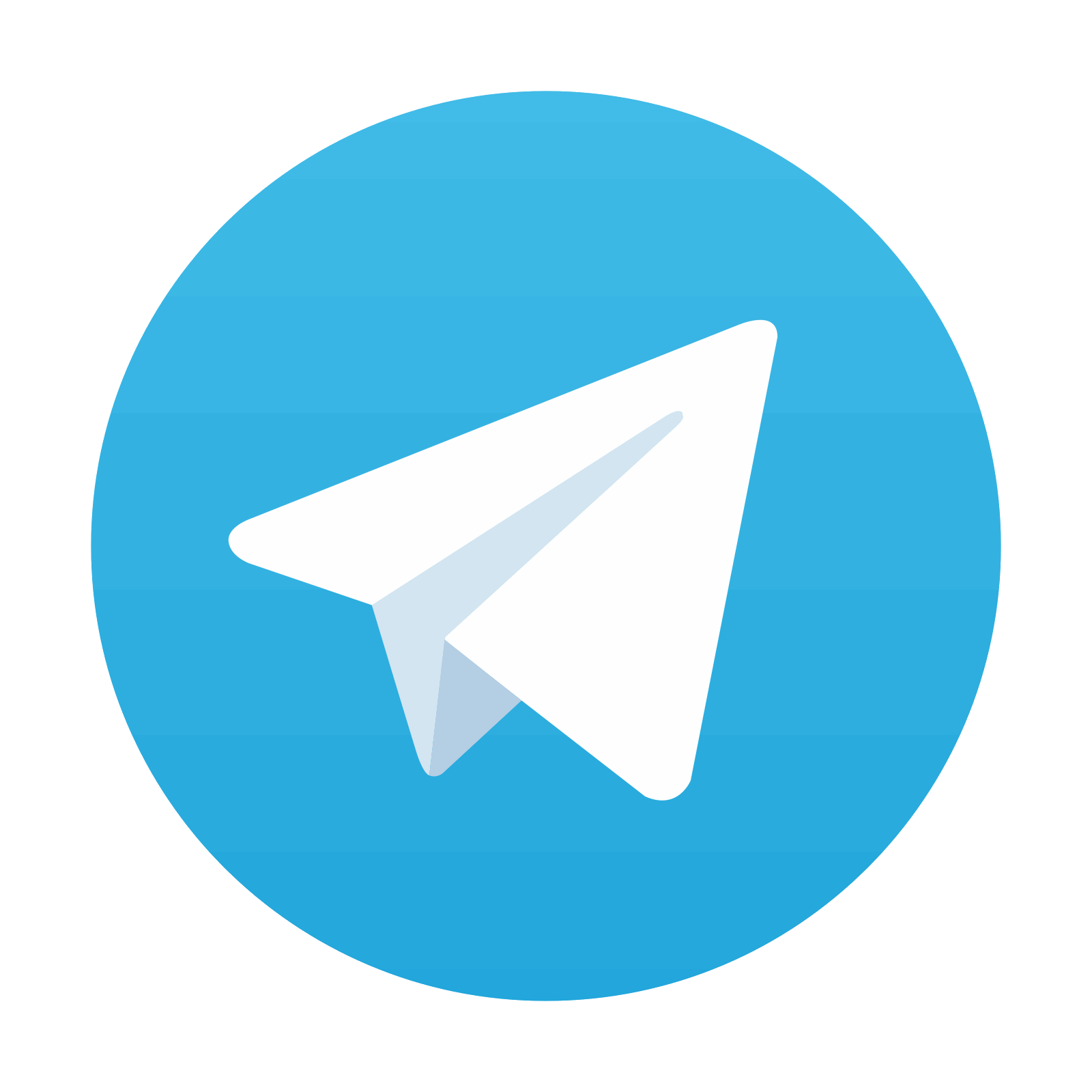
Stay updated, free articles. Join our Telegram channel

Full access? Get Clinical Tree
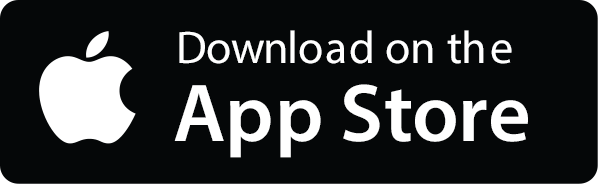
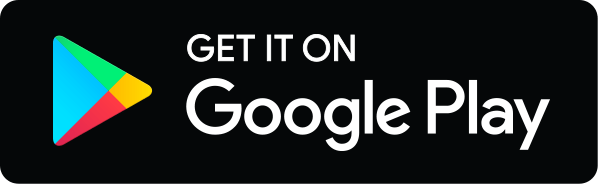
