Fig. 5.1
Structural en face optical coherence tomography (OCT) of normal eyes obtained with swept-source OCT. In the upper row, en face OCT was registered taking the Bruch’s membrane as a reference surface to compensate the natural curvature of the eye. Each scan documents an individual choroidal layer, as follows: retinal pigment epithelium (RPE) (a), choriocapillaris (b), inner choroid (c), and outer choroid (d). Note at the level of the outer choroid (d) a peripapillary area without choroidal vessels, correspondent to the so-called watershed zone. In the middle row, original en face OCT scans without registration. Each scan documents multiple choroidal layers; the following levels are documented in the center of the scans: RPE (e), choriocapillaris (f), inner choroid (g), and outer choroid (h). In the lower row, cross-sectional OCT centered at the fovea. Individual choroidal layers are not readily appreciated. The horizontal lines indicate the level of en face scans, as follows: RPE (i), choriocapillaris (j), inner choroid (k), and outer choroid (l)

Fig. 5.2
Structural en face optical coherence tomography (OCT) of normal eye obtained with swept-source OCT. Images were registered taking the Bruch’s membrane as a reference surface to compensate the natural curvature of the eye. En face OCT at the level of the retinal pigment epithelium (a) is characterized by homogeneous hyperreflective layer. The only hyporeflective structures are the optic disc and the shadowing artifact of overlying retinal vessels. En face OCT at the level of the choriocapillaris (b) shows homogeneous reticular pattern throughout the scan. En face OCT at the level of the inner choroid (c) shows in the macular area a complex, interwoven network of short and relatively thin vascular segments crossing over each other and homogeneously distributed, while in extramacular areas the vessels are fewer in number. En face OCT scans at the level of the outer choroid (d) show wider vessels, interwoven in the center of the macula but assuming a distinct radial distribution beyond the macular area toward the equator

Fig. 5.3
Structural en face optical coherence tomography (OCT) of normal eye obtained with a swept-source OCT prototype operating at 1050 nm. Images were registered taking the Bruch’s membrane as a reference surface to compensate the natural curvature of the eye. Magnified fields show details of the individual layers as follows: retinal pigment epithelium (a), choriocapillaris (b), inner choroid (c), and outer choroid (d). Vertical and horizontal scale bars measuring 1.5 mm
5.2.3 Optical Coherence Tomography Angiography
OCT angiography is a recently developed technology that allows noninvasive documentation of vascular structures and is a useful investigative tool of the choriocapillaris and choroidal microvasculature in physiological and pathological conditions. The basic principle of OCT angiography assumes that all other elements remaining static, the only difference between sequential OCT scans in a given location of the tissue is caused by the motion of erythrocytes within the vessels. It can be obtained with several different technologies, classified into two major groups: amplitude based and phase based. Important examples of amplitude-based technologies are the split-spectrum amplitude-decorrelation angiography (SSADA), scattering OCT, and speckle variance (Jia et al. 2012), while examples of phase-based technologies are phase-variance OCT and Doppler OCT (Fingler et al. 2007; Kim et al. 2011). All of these can be implemented in high-speed Fourier-domain OCT, either SD-OCT or SS-OCT systems.
OCT angiography has fundamental differences when compared to standard fluorescein angiography or indocyanine green angiography; direct comparison between these methods is not always feasible and should be taken with caution. The proper interpretation of OCT angiography must consider the physical principles of imaging acquisition: images do not change throughout the acquisition process, and since no exogenous dye is used, leakage, staining, or pooling is not documented. On the other hand, it is subject to specific artifacts mostly related to the optical reconstruction of vascular tissues. One important limitation is the fact that OCT angiography identifies the vascular flow within a limited dynamic range. There is a sensitivity limit, which is the slowest detectable flow determined by the elapsed time between sequential scans. There is also a saturation limit, which is the fastest detectable flow that is differentiated by the decorrelation signal. All these considerations are relevant when interpreting OCT angiography of the choroidal vasculature. Although the choroid has the highest blood flow per unit weight tissue in the human body (Alm and Bill 1973), the blood flow in the choriocapillaris and choroidal vasculature may occasionally be too slow or too fast to be documented by this imaging method.
5.3 Normal Choroidal Thickness on Structural Optical Coherence Tomography
While choroidal thinning on OCT has been associated with various pathological conditions such as AMD, age-related choroidal atrophy, and high myopia, it is important to note that the total choroidal thickness also varies widely in the normal population at both the subfoveal and the extramacular locations (Branchini et al. 2013; Flores-Moreno et al. 2013; Kim et al. 2011; Spaide 2009; Wei et al. 2013). Variability related with gender, age, axial length, and refractive error has been reported (Barteselli et al. 2012; Margolis and Spaide 2009; Sanchez-Cano et al. 2014). It may be challenging, therefore, to establish normative values or to identify a clear threshold between normal and pathological choroidal thickness in the clinical setting.
The subfoveal total choroidal thickness has been found to correlate significantly with age, and progressive subfoveal total choroidal thinning occurs in normal aging eyes (Fujiwara et al. 2009; Ikuno and Tano 2009; Ikuno et al. 2010; Manjunath et al. 2010; Margolis and Spaide 2009; Hirata et al. 2011; Ooto et al. 2015). Subfoveal choroidal thickness was also found to be negatively correlated with axial length (Benavente-Perez et al. 2010; Esmaeelpour et al. 2010; Hirata et al. 2011; Li et al. 2011; Ooto et al. 2015) and positively correlated with refractive error (Fujiwara et al. 2009; Ikuno and Tano 2009; Ikuno et al. 2010). Diurnal variations on the same individuals were also reported (Brown et al. 2009; Ikuno et al. 2010; Tan et al. 2012) and seem to correlate with diurnal variations of the ocular axial length (Chakraborty et al. 2011).
Progressive choroidal thinning in normal aging eyes was documented in both post–mortem histopathological studies and in vivo investigations, using either cross-sectional OCT or en face OCT (Adhi et al. 2015; Ferrara et al. 2016; Ikuno et al. 2010; Manjunath et al. 2011; Margolis and Spaide 2009; McLeod and Lutty 1994; Spaide 2009; Wei et al. 2013). Investigations on en face OCT suggested that the proportion between individual choroidal layers may also change in older individuals, and it is possible that in normal aging eyes, the choriocapillaris loss may be relatively more significant than loss of medium-size and large-size choroidal vessels. Morphological changes in the choriocapillaris and choroidal vasculature patterns have not been correlated so far with the normal aging process (Ferrara et al. 2016).
Caution is needed when comparing choroidal thickness measurements reported in different studies available in the literature, because variations in demographic or clinical characteristics may preclude a direct correlation between different cohorts. In one of the first attempts to obtain normative values for the normal population using SD-OCT, the mean subfoveal total choroidal thickness in a small group of patients, mean age of 50 years, was found to be 287 μm, which was found to decrease approximately 15 μm for each decade of life (Margolis and Spaide 2009). Subfoveal total choroidal thickness seems to decrease especially after the age of 40 (Ferrara et al. 2016); Ruiz-Medrano et al. 2014). In another cohort, the following measurements were found on SS-OCT for each age range: 325.6 ± 51.1 μm (0–10 years), 316.7 ± 90.1 μm (11–20 years), 313.9 ± 80.3 μm (21–40 years), 264.6 ± 79.3 μm (41–60 years), and 276.3 ± 88.8 μm (older than 60 years), in addition to different choroidal thickness profiles for each age group with greater variations in the temporal quadrant of the fundus (Ruiz-Medrano et al. 2014). Several other studies have reported highly variable values for mean subfoveal total choroidal thickness, investigating cohorts of different demographic characteristics (Benavente-Perez et al. 2010; Hirata et al. 2011). As a gross estimate, in vivo subfoveal total choroidal thinning was considered to progress 1.56 μm per year, which is higher than 1.1 μm per year estimated from post–mortem specimens (Margolis and Spaide 2009). Although several aspects can justify such difference, to some extent it may be related to the lack of blood flow in histological examination (Feeney-Burns et al. 1990; Ramrattan et al. 1994; Sarks 1976).
In addition, normal variations of the choroidal-scleral contour also contribute to the variability in total choroidal thickness measurements, which vary throughout the fundus (Adhi et al. 2014; Branchini et al. 2013). In normal eyes the choroid is thickest under the fovea, showing marked thinning toward the periphery in all four quadrants – nasal, temporal, superior, and inferior (Esmaeelpour et al. 2010; Hirata et al. 2011; Ikuno et al. 2010; Manjunath et al. 2010). The peripapillary total choroidal thickness was found to be the thinnest inferiorly to the optic disk (Ho et al. 2011; Tanabe et al. 2012).
The suprachoroidal layer is not commonly observed on OCT in normal eyes. It is estimated to be documented on cross-sectional OCT in only 5 % of healthy emmetropic eyes as two distinct bands: an upper hyperreflective band corresponding to the suprachoroidal layer and a lower hyporeflective band supposedly corresponding to the suprachoroidal space, which in vivo was previously visualized only in eyes with choroidal effusion (Michalewska et al. 2015).
5.4 Normal Choroidal Morphology on Structural En Face Optical Coherence Tomography
The normal choroid has been investigated on recent research using depth-resolved en face OCT, extracted from tridimensional datasets, which is strikingly superior than cross-sectional OCT for this matter. Most of these recent studies use SS-OCT, since it documents deeper structures better than SD-OCT (Adhi et al. 2015; Ferrara et al. 2014; Ferrara et al. 2016; Montaghiannezam et al. 2012; Sohrab et al. 2012; Sohrab and Fawzi 2013; Srinivasan et al. 2008). The morphological features of the choroid do not seem to present qualitative changes during normal aging, although quantitative changes are typically observed, as discussed above (Ferrara et al. 2016).
Structural en face OCT of the RPE in normal eyes is regularly homogeneous and hyperreflective throughout the scan, including the macular and peripapillary areas. The only hyporeflective elements correspond to the optic disk and to the tomographic shadowing artifact of the overlying normal retinal vessels (Ferrara et al. 2016). These references are important for the proper interpretation of the image, because this artifact is transmitted on en face layers throughout the volumetric dataset and should not be confounded with actual choroidal vessels.
Structural en face OCT of the choriocapillaris and choroidal microvasculature are challenging to be obtained, even in normal eyes, because of the small caliper and complexity of the vascular network. No distinct capillary vessels can be readily identified on en face OCT, with the current available technology. Instead, they appear as homogeneous reticular pattern throughout the scan, including the macular and peripapillary areas, lying at a level external to the RPE and internal to the choroidal vasculature. Microvascular choroidal anastomoses between the choriocapillaris and terminal arterioles or venules from the inner choroid are also present at this level. However, since both choriocapillaris and microvascular anastomosis appear on en face OCT as a reticular pattern, no tomographic distinction can be made between them (Ferrara et al. 2016) (Fig. 5.4).


Fig. 5.4
Optical coherence tomography (OCT) angiography of a normal eye obtained with ultrahigh speed swept-source OCT prototype operating at 1060 nm (Choi et al. 2013). Scans document the choriocapillaris in different sectors of the fundus. In the center of the macula (a), the choriocapillaris shows dense reticular appearance, while it shows less dense appearance in the mid-periphery (b) and a lobular appearance toward the equator (c)
Structural en face OCT of the inner and outer choroid in normal eyes has distinct characteristics, depending on the area of the fundus and on the level of the scan. In the macular area, choroidal vessels appear on en face OCT as highly complicated, interwoven, short vascular segments crossing over each other. In en face scans of extramacular areas, choroidal vessels are straighter and fewer in number representing a radial distribution outward from the center of the macula toward the equator (Ferrara et al. 2016). En face scans at the level of the inner choroid present thinner vessels, homogeneously distributed in the macular area through the topography of the retinal vascular arcades. En face scans at the level of the outer choroid present wider vessels, interwoven in the center of the macula but assuming clear radial distribution beyond the macular area toward the equator (Montaghiannezam et al. 2012; Sohrab et al. 2012). No distinct anatomic boundary can be determined between inner and outer choroid, since vessel caliber and distribution change in a progressive gradient. Also, arteries and veins cannot be differentiated based on tomographic features (Ferrara et al. 2016). A peripapillary area of preserved choriocapillaris but absent medium-size and large-size choroidal vessels, corresponding to the angiographic description of the so-called watershed zone (Hayreh 1975; Hayreh 1990), can be documented on en face OCT (Fig. 5.5).


Fig. 5.5
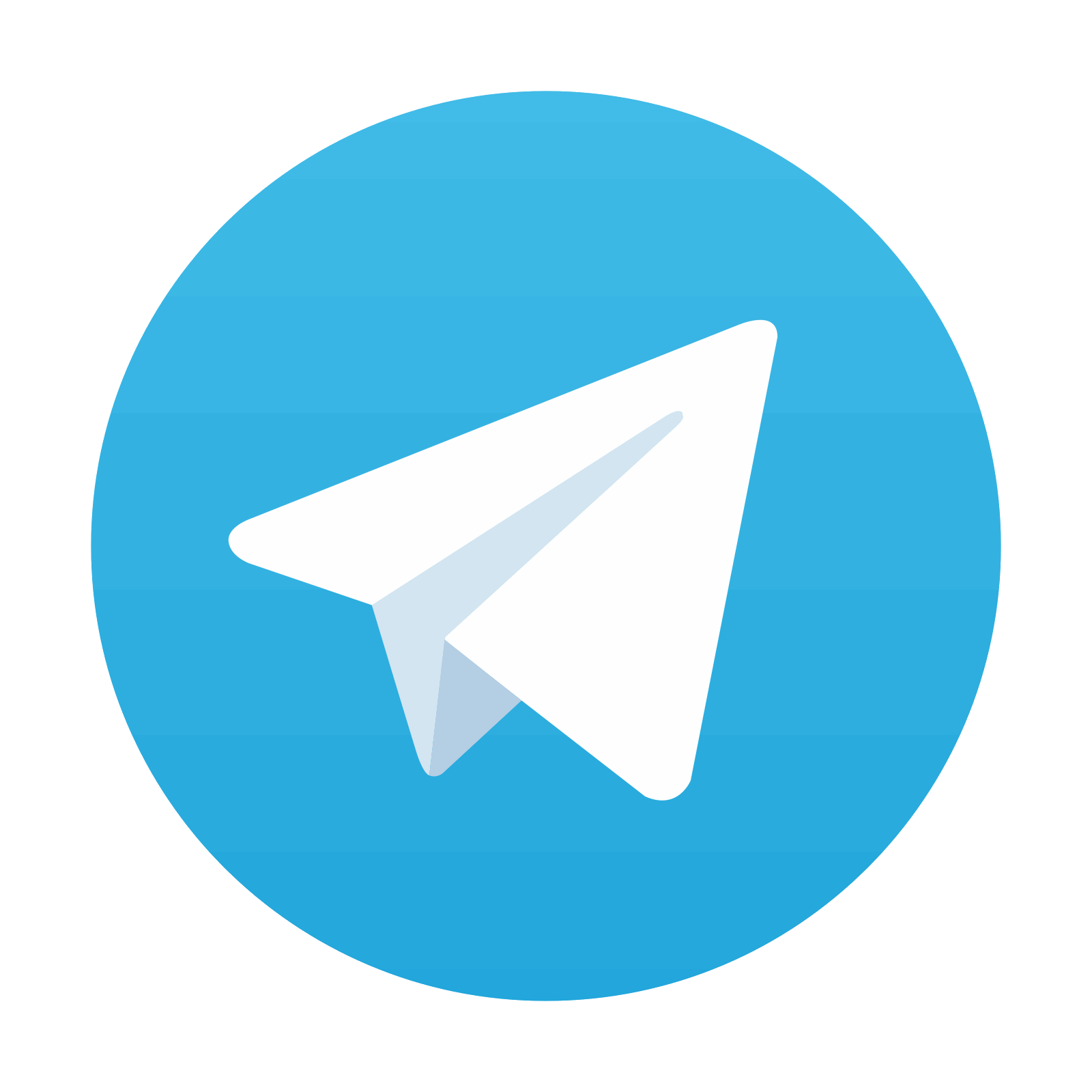
Optical coherence tomography (OCT) angiography of a normal eye obtained with a commercially available spectral-domain OCT. OCT angiography documents the choriocapillaris (a) in the center of the macular area. OCT angiography of the retinal circulation is also shown in comparison with the choroidal circulation, at the level of the deep retinal plexus (b) and superficial retinal plexus (c)
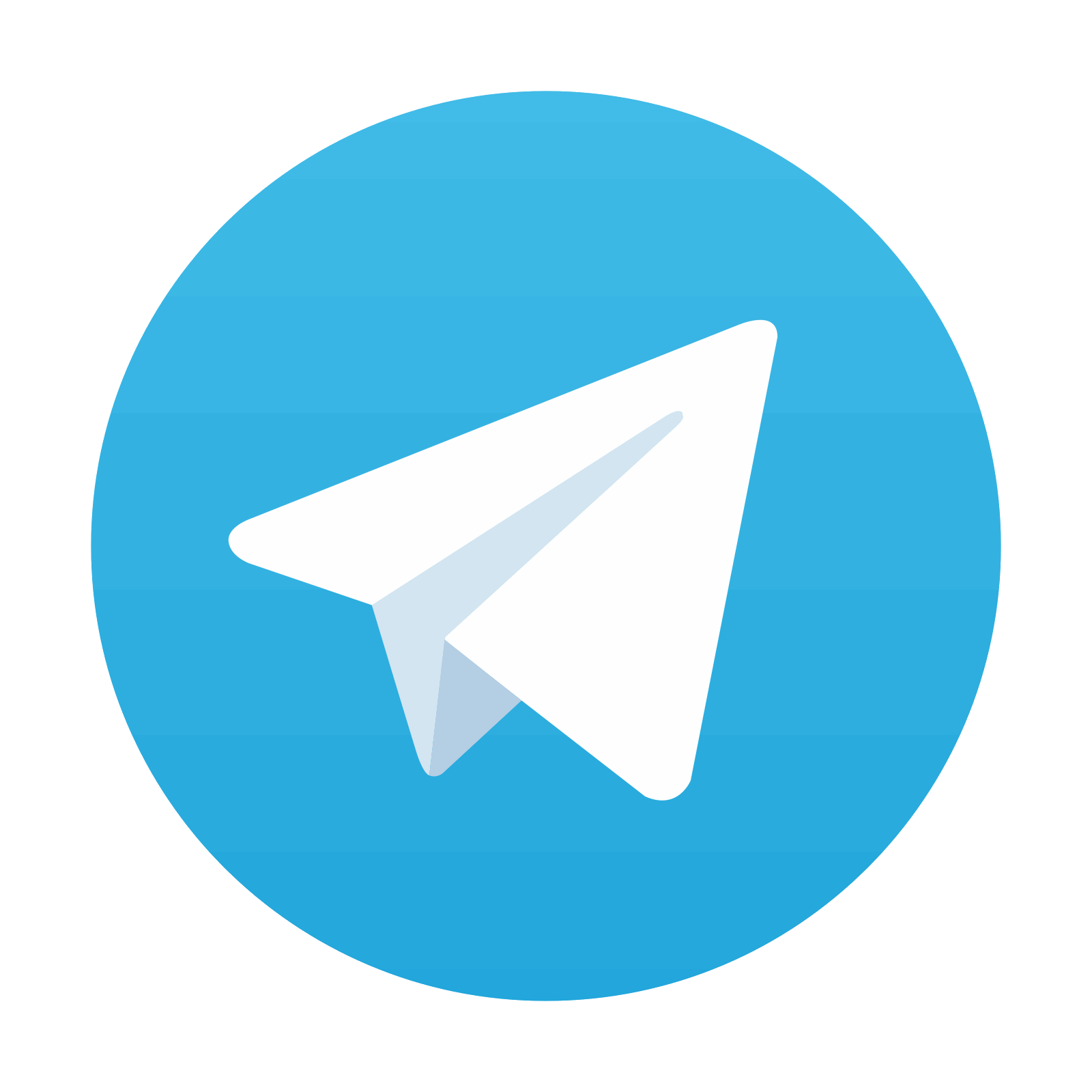
Stay updated, free articles. Join our Telegram channel

Full access? Get Clinical Tree
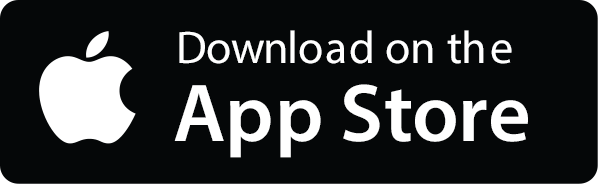
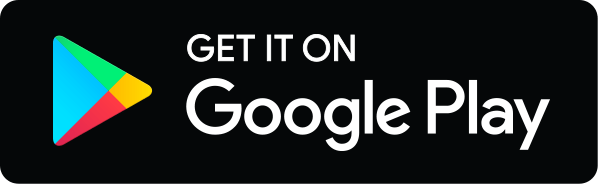
