New and Future Antiangiogenic Therapy
Rishi P. Singh
Peter K. Kaiser
INTRODUCTION
Blood vessel development is termed either vasculogenesis or angiogenesis. Vasculogenesis is the process of normal blood vessel formation during development. Angiogenesis occurs when pre-existing vascular beds sprout new vessels in response to stimuli. Angiogenesis in adulthood is primarily seen in pathologic situations and is a highly complex process requiring the coordinated action of growth factors and cellular events. The process is controlled by a balance of pro- and antiangiogenic factors. Although there are several potential regulators of angiogenesis, reports have suggested that vascular endothelial growth factor (VEGF) may be one of the most important regulators of vascular development and differentiation seen in age-related macular degeneration (AMD), perhaps because it is the most specific growth factor for the vascular endothelium. This hypothesis was verified with major breakthroughs in molecules that suppress VEGF including pegaptanib (Macugen™, OSI-Eyetech, New York, NY), bevacizumab (Avastin™, Genentech, South San Francisco, CA), and ranibizumab (Lucentis™, Genentech, South San Francisco, CA), and they have helped in achieving significant success in the treatment of AMD (1,2).
Despite the impressive gains in vision seen with anti-VEGF therapy, not all patients exhibit improvement after treatment. In the MARINA trial of ranibizumab for AMD, 10% of patients suffered three lines of visual loss despite receiving monthly injections for 24 months. One possible explanation for this is that anti-VEGF therapy only addresses one of multiple pathways of pathogenesis. Another explanation is that as vessels mature, they lose their VEGF sensitivity and thus other pathways are required to treat these patients. Furthermore, anti-VEGF therapies are very costly and often require monthly intravitreal injections. Thus, there is a prevailing clinical need for pharmacotherapeutics that are less costly, less invasive, and require less frequent administration either through combination therapy or different delivery methods in monotherapy. Finally, studies with ranibizumab have elucidated that anti-VEGF drugs are not without safety issues including a risk of stroke in patients with prior history of stroke (3). This has led to the development of alternative antiangiogenesis therapies to improve the results of anti-VEGF therapy.
When examining potential future targets for angiogenesis, researchers have returned to the root causes of AMD. There is increasing evidence that suggests that AMD is not strictly a vascular disease, but instead is a more complex process. The process of choroidal neovascular membrane coroidal neovascularization (CNV) formation is a multifaceted interplay between genetic predisposition, hypoxia, inflammation, oxidative stress, and other factors. CNV was once thought to be only a vascular disease; however, lately nonvascular components including myofibroblasts, macrophages, and other inflammatory cells have been found in AMD specimens. In addition, disregulation and mutations in complement factor 3 and other mutations in the alternate complement pathway have also been causally related to an increased risk of development choroidal neovascularization in AMD, leading to researchers looking at the inflammatory cascade as a potential target. Finally, drusen accumulation has been shown to lead to localized tissue hypoxia as it impairs nutrient transport from the choriocapillaris to outer retinal tissues. These factors lead to modification of the upstream and downstream regulators of VEGF that are proangiogenic and promotion of antiangiogenic molecules such as pigment epithelium derived factor (PEDF) as the potential targets (Fig. 12-1). The purpose of this chapter is to describe new therapies for pathologic angiogenesis currently under development. The majority of targets discussed have never appeared in the ophthalmic literature but have shown significant promise in other fields of medicine.
HYPOXIA-INDUCED FACTORS IN AGE-RELATED MACULAR DEGENERATION
Hypoxia has been established as a major contributor to tumor growth. However, only recently has it also been identified as a risk factor in the formation of choroidal neovascularization in AMD. Studies have established that relative hypoxia is caused by disturbance of the limited blood supply in the macula and the high oxygen demand of the photoreceptors over time. Alterations in choroidal blood flow have been previously described in patients with AMD (4). With age, Bruch’s membrane undergoes an accumulation of incompletely degraded cellular debris (lipofuscin) that leads to the formation of drusen. This change in Bruch’s membrane has a pronounced impact on the metabolic transfer leading to localized hypoxia.
The regulation of angiogenesis by hypoxia is mediated by hypoxia-inducible factor (HIF-1). This factor switches on a series of genes participating in compensatory mechanisms that support cell survival. In particular, the α subunit stimulates neovascularization by activating the transcription of over 60 genes including the activation and release of erythropoietin, hemo-oxygenase (mediates oxygen binding to heme), VEGF, and inducible nitric oxide synthase, which participates in local blood vessel dilation (5, 6, 7 and 8). The increased vascular permeability seen in angiogenesis, which is largely mediated by VEGF,
leads to the extravasation of plasma proteins such as fibrin, inflammatory mediators, and numerous growth factors (fibroblast growth factor, FGF; insulinlike growth factor, IGF-1). Growth factor liberation in turn causes increased expression of integrins, which are cellular adhesion molecules that interact with the extracellular matrix (ECM), and proteinases that breakdown the surrounding ECM. Both of these processes are essential for cellular migration and tubule formation. Therefore, the inhibition of HIF-1α could be a potential therapeutic target in AMD.
leads to the extravasation of plasma proteins such as fibrin, inflammatory mediators, and numerous growth factors (fibroblast growth factor, FGF; insulinlike growth factor, IGF-1). Growth factor liberation in turn causes increased expression of integrins, which are cellular adhesion molecules that interact with the extracellular matrix (ECM), and proteinases that breakdown the surrounding ECM. Both of these processes are essential for cellular migration and tubule formation. Therefore, the inhibition of HIF-1α could be a potential therapeutic target in AMD.
One example of such an inhibitor is RTP801i (Quark Biotech, Inc./Pfizer). This molecule uses RNA interference to block the production of RTP801 which is upstream of HIF-1α, leading to direct transcriptional inhibition of HIF-1α. RTP801i has been shown to be effective in animal models of ischemic disease. For example, a study using RTP801 knockout mice revealed a significant reduction in retinal neovascularization (9). In animal models of ischemic stroke, RTP801 is significantly upregulated, and its inhibition results in decreased neovascularization (10). RTP801i is in phase 2 testing for exudative AMD.
mTOR INHIBITION AS AN INHIBITOR OF ANGIOGENESIS
Rapamycin is produced by Streptomyces hygroscopicus and was first isolated from soil samples taken from Easter Island (11). It was initially used as an antifungal and potent immunosuppressant (12,13). It was not until much later that the downstream target of rapamycin, mTOR (mammalian target of rapamycin), was identified as a serine/threonine kinase in budding yeast Saccharomyces cervisiae (14). mTOR functions to control cellular growth by regulating the cellcycle dependent polarization of the actin cytoskeleton.
Recent studies have shown that HIF-1α transcription is dependent on mTOR activation. Thus, rapamycin inhibitors would indirectly cause HIF-1α inhibition and lead to decreased angiogenesis and choroidal neovascularization. Current rapalogs in clinical trials for nonocular diseases include rapamycin/sirolimus (Wyeth), RAD-001/everolimus (Novartis), and A23573 (Ariad). Each of these molecular structures differs only slightly from each other in solubility and stability (15). Rapamycin and its analogs form a ternary complex with FK-506 binding protein and mTOR resulting in a potent inhibition of mTOR signaling.
Systemic rapamycin has been shown to inhibit retinal and choroidal neovascularization in animal models of disease. Adult mice that underwent laser-induced CNV formation and treatment with rapamycin showed significant reduction in CNV size and extent compared to controls. The impact on retinal neovascularization by rapamycin was studied using the ROP hyperoxia/hypoxia model in mice. Again, a significant reduction in retinal neovascularization in rapamycin-treated animals over placebo was observed. Increases in VEGF were seen in both studies suggesting that rapamycin was acting in a pathway different from the VEGF pathway for inhibition (16). Sirolimus (Macusight) is an ocular rapalog being tested in phase 1 study in AMD.
INTEGRINS
Integrins are receptors on the cell surface that have been shown to be critical in a wide variety of cellular events including adhesion, migration, invasion, proliferation, cell survival, and apoptosis. Integrins also serve as receptors on cell surfaces allowing for adhesion of cells to ECM proteins such as vitronectin, fibronectin, laminin, collagen, and fibrinogen. Integrins are obligate heterodimers containing two distinct membrane spanning chains, called the α (alpha) and β (beta) subunits. In mammals, 19 α and 8 β subunits have been characterized. The subunits span through the cellular membrane, and in general have very short cytoplasmic domains of about 40 to 70 amino acids. Recent studies have also demonstrated that growth factors such as bFGF and VEGF isoforms (189, 165, but not soluble VEGF 121) can directly bind to α5β3 (17, 18 and 19). The binding of integrins to ECM proteins causes intracellular signal transduction leading to the activation of intracellular tyrosine kinases and transcription factors.
Initial observations regarding the role played by integrins in angiogenesis came from tumor vasculature studies. Brooks and then Friedlander were the first to identify that integrin blockade can lead to inhibition of the angiogenic response seen in tumors and in retinal neovascularization (20, 21, 22 and 23). In particular, the α5β3 and α5β1 integrins are found on proliferating endothelial cells. However, the presence of these integrins is dependent on the tissue studied. Only α5β3 was found in choroidal neovascular membrane specimens. However, both sets of integrins are found on retinal neovascular specimens from proliferative diabetic retinopathy (21,24). Blockade of α5β3 or α5β1 function by an antibody or peptide antagonists prevents blood vessel formation in numerous preclinical models of angiogenesis including chick chorioallantoic membrane, rabbit cornea, and mouse retina (20,22 and 25).
The process of integrins-induced angiogenesis is still being elucidated and several potential mechanisms are hypothesized. Overall, integrins are required for the transmission of the initial signals leading to the activation of the angiogenesis cascade and are subsequently altered to allow for invasion and remodeling of the ECM. Crosstalk between integrins and other growth factor receptors has been demonstrated and are thought to play a critical role in angiogenesis. Reynolds et al. found that inhibition of α5β3
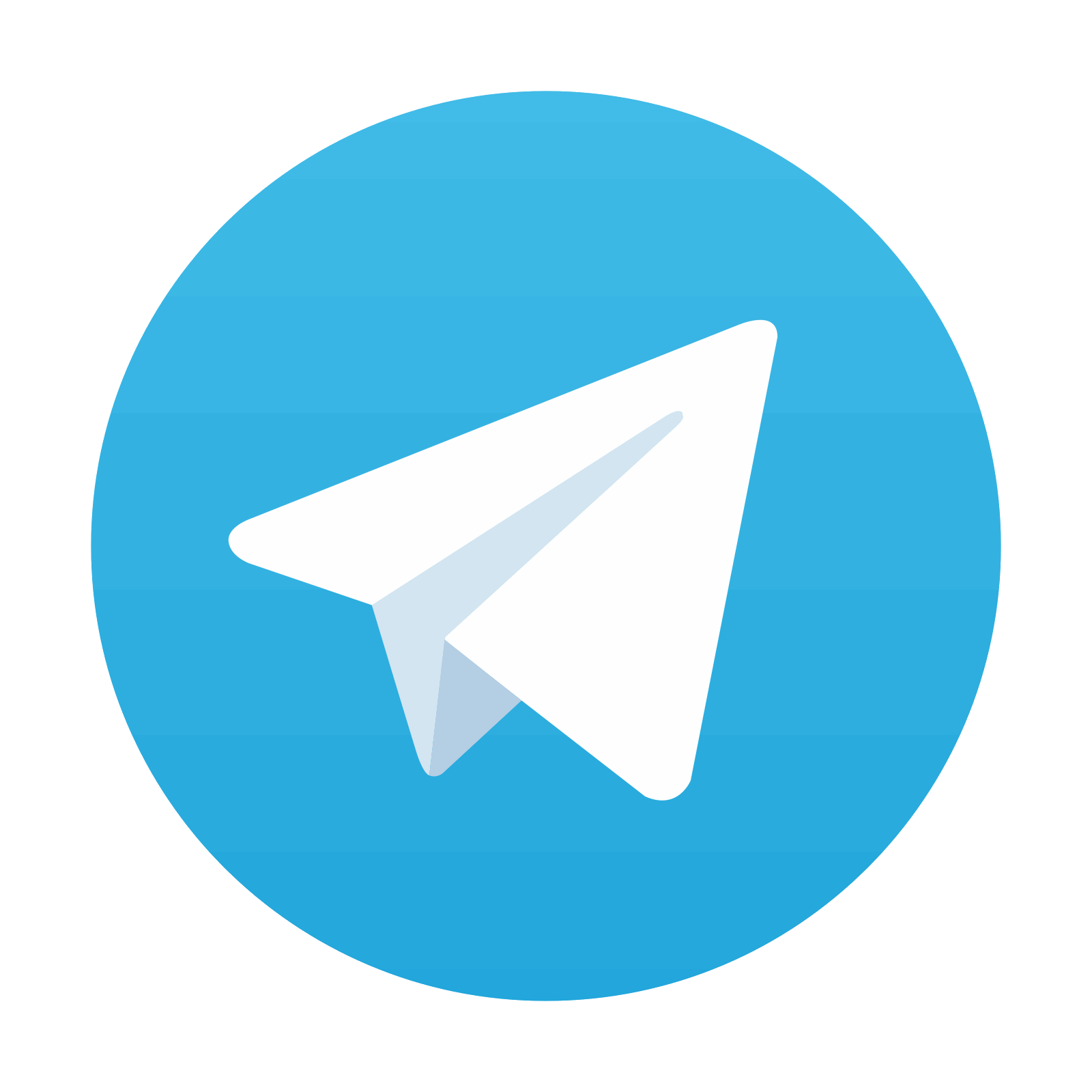
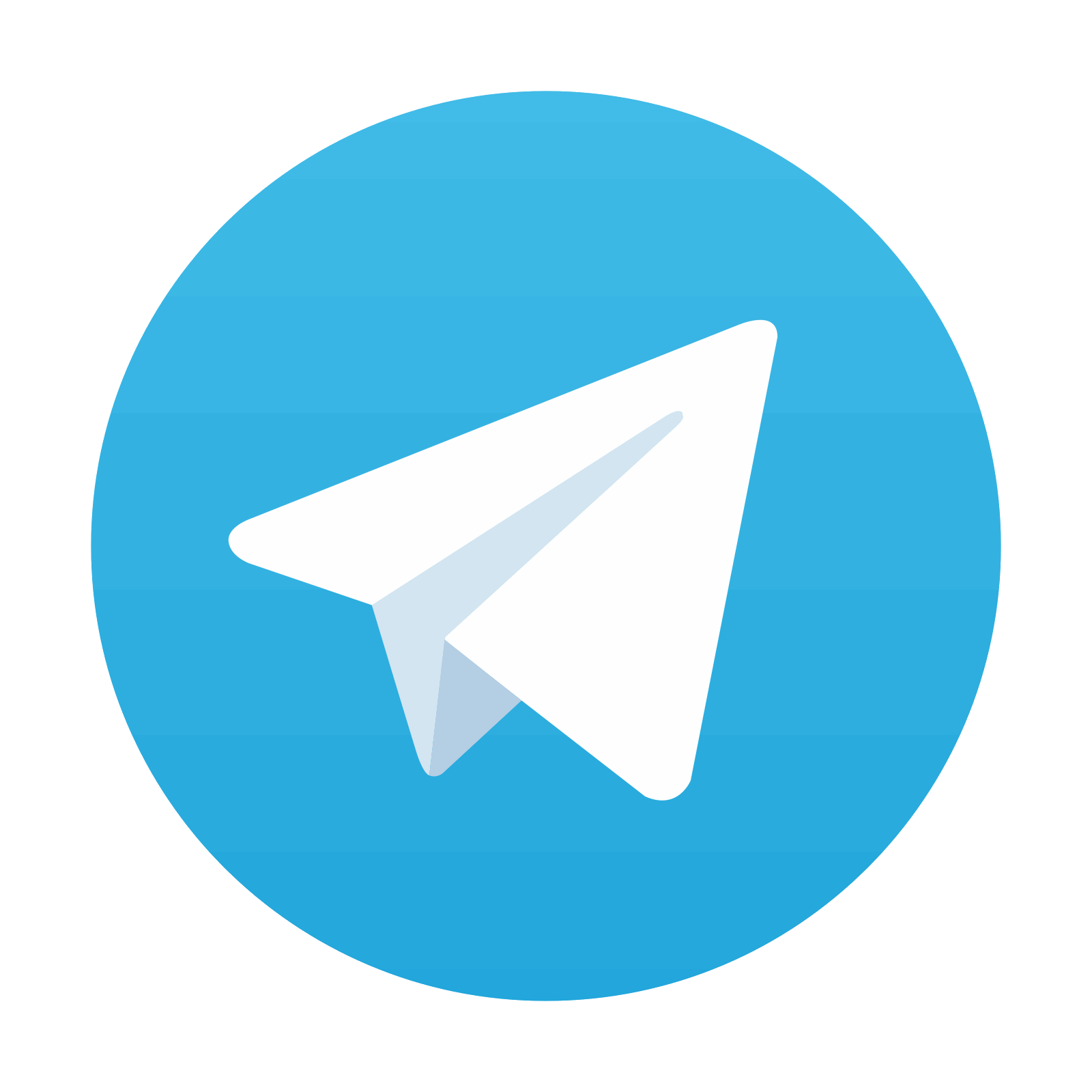
Stay updated, free articles. Join our Telegram channel

Full access? Get Clinical Tree
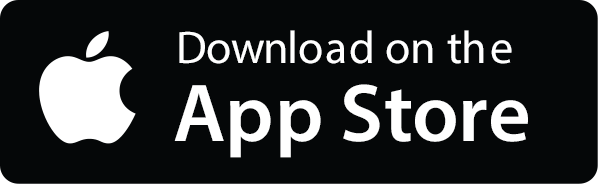
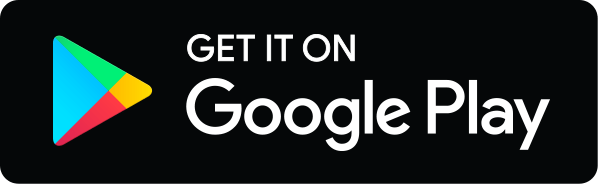