Fig. 14.1
Juvenile myasthenia gravis . (a) Right eye ptosis and (b) adduction deficit and (c) left eye elevator deficit. The child had physiologic anisocoria
As mentioned previously, the most common clinical sign of myasthenia gravis is eyelid ptosis, which can be unilateral, bilateral, or alternating and is characteristically variable over time. Fatigability , which is strongly suggestive of myasthenia, may be elicited on examination, with a visible worsening of eyelid ptosis after sustained upgaze (e.g., for 30 seconds). Curtaining, or lowering of the contralateral eyelid when the more ptotic eyelid is mechanically elevated, is also suggestive, though not specific. The Cogan lid twitch , a brief, rapid overshoot of one or both eyelids with a slower recovery to resting position when recovering from downgaze, is also a characteristic, though not highly specific, finding [12]. A variant of the lid twitch, lid hopping, which consists of a hopping motion of the eyelids in attempted side gaze, can also be seen. Orbicularis oculi weakness is frequently present, and fatigue can sometimes be elicited with attempted sustained forceful eye closure, leading to gradual opening of the palpebral fissure despite efforts to keep the eye closed. Ocular motility deficits in myasthenia can follow any pattern and can deceptively mimic any ocular motor cranial nerve palsy. The eye movements may also exhibit fatigability after sustained gaze in a certain direction, and saccadic velocity may be decreased. Notably, the pupils are not involved clinically in myasthenia gravis.
Simple bedside diagnostic tests for myasthenia gravis include the sleep test and ice test. In the former, patients are allowed to nap for 30 min; a visible improvement in their ptosis after resting is considered suggestive of myasthenia gravis [13]. The ice test involves the placement of an ice pack over the ptotic eyelid for 1 min, with an improvement in ptosis after the ice is removed indicative of likely myasthenia. Nonetheless, ice testing is not very practical in many children, who may not be able to cooperate with this test. Although the above testing maneuvers are simple and readily available, they are not definitive.
The diagnosis of myasthenia should be confirmed, with serum acetylcholine receptor antibody testing and, if possible, electromyography (EMG) with repetitive nerve stimulation testing, single-fiber electromyography, or edrophonium testing. Serum AChR binding antibody testing is essentially diagnostic of myasthenia gravis, as the test is highly specific. For adults, the antibody is present in about 90% of generalized myasthenia patients, but only in about 50–70% of those with ocular myasthenia [14–17]. In children, seronegativity appears to be even more frequent, with about 70% of generalized and 40% of ocular myasthenia patients being positive on antibody testing [2, 18, 19]. Testing for either AChR binding or modulating antibodies shows similar sensitivity in generalized myasthenia gravis (86%), and checking both titers increases the sensitivity slightly to around 90% [20]. AChR blocking antibodies appear to be present in just over half of generalized myasthenia patients, and titers may correlate with disease activity [20]. When performing serological testing for myasthenia, the binding antibody is usually the first-line test. If there is high clinical suspicion and the binding antibody is negative, modulating and blocking antibodies can be ordered subsequently in the attempt to increase diagnostic yield. In our experience, the sensitivity of antibody testing may also be augmented by repeating the serology several months after an initial negative test, when myasthenia gravis is still highly suspected in a patient. A minority of individuals with AChR antibody seronegative myasthenia have serum anti-muscle-specific kinase (anti-MuSK) antibodies . These patients, usually adults, generally have a predilection for oropharyngeal, cervical, and respiratory symptoms and more often require respiratory support and plasma exchange for treatment [21–23]. Single-fiber EMG , which is very sensitive, and repetitive nerve stimulation EMG, though very helpful in diagnosing seronegative myasthenia in adults, are not practical in children because of the pain involved and inability to be performed under anesthesia. Pharmacologic testing with acetylcholinesterase inhibitors can also be used for the diagnosis of myasthenia, with a clear improvement in ptosis and/or ocular motility after administration being a positive result. Intravenous edrophonium or intramuscular neostigmine can be used in children, though the former requires placement of an IV cannula, and children must be monitored closely for side effects such as bradycardia and gastrointestinal upset. Pharmacologic testing appears to be about 90% sensitive in pediatric patients [2]. Pediatric patients with ocular myasthenia gravis do not need routine chest imaging to screen for a thymoma as this is exceptionally rare in children. In patients for whom thymectomy is planned, chest imaging with MRI is preferred due to the lack of ionizing radiation. In addition, if the clinical pattern of ptosis and ocular motor deficits is suggestive of focal cranial nerve involvement, the patient should undergo brain neuroimaging to exclude the possibility of an explanatory lesion before the diagnosis of myasthenia is made.
Oral acetylcholinesterase inhibitors , which increase the concentration of acetylcholine in the neuromuscular junction by slowing its enzymatic degradation, are the first-line treatment for myasthenia gravis. Most often, pyridostigmine is used. These agents work very well for systemic and oropharyngeal weakness, and reasonably well for ptosis, but are poor in improving ocular motility. The most common side effects are gastrointestinal upset, cramping, and diarrhea. Very often, chronic treatment with corticosteroids is required, but may lead to stunted growth. In cases of weakness resistant to acetylcholinesterase inhibitors and steroids, long-term immunosuppression with azathioprine or cyclosporine may be needed, despite many undesirable potential side effects. All myasthenic children under 8 years of age should be regularly screened for amblyopia and treated with patching or pharmacologic penalization if needed. There is limited, though growing evidence that thymectomy, via transsternal or thoracoscopic approaches [24], may increase the rate of spontaneous remission, decrease medication dependence, and reduce the risk of generalization in ocular myasthenics [6, 25]. In this regard, thymectomy should be strongly considered in all patients with either ocular or generalized myasthenia.
Congenital Myasthenic Syndromes
As in myasthenia gravis, the congenital myasthenic syndromes can also present with fatigable ocular and systemic weakness, usually presenting between birth and early childhood, but are not of an autoimmune or antibody-mediated etiology. This family of conditions is caused by genetic defects, most frequently in subunits of the nicotinic AChR, that lead to dysfunctional neurotransmission at the neuromuscular junction [26]. Available treatments include pyridostigmine, 3,4-diaminopyridine, quinidine, fluoxetine, and ephedrine, all exhibiting variable degrees of effectiveness [26]. These patients sometimes need ptosis surgery to prevent amblyopia and improve head position.
Horner Syndrome
Horner syndrome, consisting of the triad of unilateral upper and lower eyelid ptosis, pupillary miosis, and facial anhidrosis, should always be considered in the differential diagnosis of acquired or congenital pediatric ptosis, as it can be one of the early signs of a number of potentially threatening etiologies. The syndrome is caused by the disruption of the ipsilateral oculosympathetic pathway, which consists of three neurons. The first-order neuron starts in the hypothalamus and descends through the midbrain, pons, medulla, and spinal cord to synapse with the second-order neuron in the ciliospinal center of Budge at the C8-T2 spinal level. The axon of the second-order neuron then ascends along the paraspinal sympathetic chain and synapses with the third-order neuron in the superior cervical ganglion, which is located at the C3 spinal level just lateral and posterior to the palatine tonsil. The axons of the third-order neuron then follow the internal carotid artery up to the cavernous sinus where, after a brief course along the abducens (sixth) nerve, they join the ophthalmic branch of the trigeminal nerve to innervate the pupillary dilator muscle, ciliary body, Müller’s (superior tarsalis) muscle, and inferior tarsalis muscle.
Disruption of any of the three neurons of the sympathetic pathway will cause a Horner syndrome, so an assessment of the entire sympathetic pathway must be performed. A lesion of the first-order neuron is infrequent in children, but when present is typically caused by brain tumors involving the hypothalamus or brainstem. More common are lesions involving the second-order neuron due to neuroblastoma or birth-related nerve root or brachial plexus injury. The third-order neuron can be disrupted in the setting of intraoral trauma, nasopharyngeal tumors, vascular anomalies, and carotid artery dissection, among others. Though uncommon in the pediatric population, about 7% of all craniocervical arterial dissections have been reported to occur in children or adolescents [27]. Although the most common reported etiology of Horner syndrome in childhood is idiopathic (~40%), one large series found that up to one-third of children with Horner syndrome of unknown etiology harbored an underlying mass lesion, with neuroblastoma accounting for 9% of total Horner cases [28]. In this regard, prompt and thorough evaluation of patients with unilateral ptosis and miosis is critical to prevent delay in diagnosis and treatment of potentially life-threatening underlying etiologies. Neuroblastoma presenting with ocular signs appears to have a better prognosis overall, likely because the ophthalmic findings triggered earlier diagnosis and treatment of the malignancy [29].
Patients with Horner syndrome are frequently asymptomatic, although older children, adolescents, or parents may complain of a droopy eyelid or different colored irides. Rarely do patients or parents note asymmetric sweating. Patients and/or parents should be queried for history or symptoms associated with craniocervical arterial dissection such as minor neck trauma, sports injuries, new headaches, jaw pain, or dysgeusia. The examiner should also elicit any history of birth injury or craniocervical trauma.
The ptosis of Horner syndrome is usually mild, rarely more than 2 mm relative to the other eye, and a subtle inverse ptosis (from dysfunction of the inferior tarsalis muscle) may be seen, which is strongly suggestive of Horner syndrome (Fig. 14.2). The resultant narrowing of the palpebral fissure may result in pseudoenophthalmos. The miotic pupil is ipsilateral to the ptotic eyelid, and the degree of anisocoria between the two pupils increases in dim lighting, with the smaller pupil dilating poorly. A dilation lag may be seen on the affected side when lights are suddenly turned off, with the miotic pupil dilating to its dim-light size more slowly than the contralateral pupil. The absence of visible dilation lag does not rule out Horner syndrome. An iris on the affected side that is lighter than the contralateral side, termed iris heterochromia , is indicative of congenital or very long-standing Horner syndrome (Fig. 14.1). Asymmetric facial perspiration may result from first- and second-order lesions. Lower intraocular pressure on the affected side may be noted because of disrupted sympathetic input to the ciliary body, resulting in decreased aqueous humor production. In acquired cases, mild conjunctival hyperemia may occur on the affected side. As neuroblastoma can cause palpable lesions, children should be examined for cervical and axillary masses.


Fig. 14.2
Idiopathic left Horner syndrome in a child with ipsilateral ptosis, miosis, and lighter iris (heterochromia)
Pharmacologic testing for Horner syndrome can determine the need for imaging studies. The cocaine test is used to confirm whether or not Horner syndrome is present. Cocaine normally causes pupillary dilation by inhibiting the reuptake of synaptic norepinephrine from the sympathetic nerve terminal on the dilator pupillae muscle. Failure of the pupil to dilate with cocaine, thus, indicates the absence of norepinephrine in the sympathetic terminal due to disruption at any point in the ipsilateral sympathetic pathway. One drop of 10% cocaine solution is applied in each eye, and then this is repeated 5 min later. If anisocoria of ≥1.0 mm (with the affected side smaller) is present 30–60 min later, this is considered a positive cocaine test and indicative of true Horner syndrome on the side of the smaller pupil [30]. In our experience, cocaine can be safely administered to infants 3 months of age and older. Because of increasing difficulty in obtaining cocaine, apraclonidine testing has been proposed as an alternative confirmatory test [31, 32], although significant side effects, such as sedation, have been reported in younger children [33]. Being a weak α1-adrenergic agonist, apraclonidine will not dilate a normal pupil, but will dilate a pupil with sympathetic denervation, as the α1-adrenergic receptors on the dilator pupillae muscle become upregulated in the absence of oculosympathetic input. This denervation hypersensitivity takes several days to develop fully. Apraclonidine testing is performed by placing one drop of 0.5% or 1% apraclonidine in each eye and reassessing the patient after 30–60 min; a reversal of the anisocoria (with the previously miotic pupil becoming larger) is considered a positive test. Reversal of ptosis and any conjunctival hyperemia on the affected side may also be seen and support the presence of Horner syndrome. Apraclonidine testing may not be reliable if it is performed in the acute phase (e.g., 1 week) after the onset of Horner syndrome, as denervation hypersensitivity has not yet developed. Hydroxyamphetamine , which is used to distinguish between first-/second-order and third-order neuronal lesion, is no longer used at most centers because of its questionable reliability and limited availability. Most importantly, patients whose clinical presentation is very consistent with Horner syndrome should undergo a diagnostic workup despite the result of pharmacologic testing. Currently our practice is to confirm Horner syndrome with cocaine in children 2 years and younger and use apraclonidine for this purpose in those older than 2.
Children with pharmacologically confirmed or clinically evident Horner syndrome less than 1 year in duration should undergo imaging of the entire oculosympathetic pathway to exclude an explanatory lesion of consequence, unless the history and/or examination reveal a clear, definite etiology [34]. The optimal imaging studies are MRI with and without contrast of the brain, neck, and upper chest, as well as MRA of the neck to rule out carotid dissection or malformation. MRA is superior to CTA in detecting arterial dissection due to the ability to visualize the mural thrombus in addition to showing the narrowed true lumen, which is the only finding on CTA [35]. Carotid ultrasound is not a reliable study to rule out arterial dissection, as the artery cannot be completely visualized and many cases would be missed [36]. Though urinary homovanillic acid (HVA) and vanillylmandelic acid (VMA) may be elevated in neuroblastoma, this is very rarely the case in patients with purely ocular findings [28]. Thus, testing HVA and VMA in this clinical context has limited utility. If available, children with suspected Horner syndrome would benefit from prompt referral to a pediatric neuro-ophthalmologist for confirmatory testing and further diagnostic evaluation.
Third Nerve Palsy
Third (oculomotor) nerve palsy is a possibility that should always be entertained in setting of ptosis, pupillary changes, and/or ocular motility abnormalities. The most common etiologies for third nerve palsy in children are distinctly different from what is seen in adults. Congenital third nerve palsy, thought to originate from an in utero insult to the nerve or midbrain nucleus, accounted for ~15% to 50% of cases in various series [37–42]. Trauma is the most common cause of acquired pediatric third nerve palsies, while other common etiologies include neoplasms and infection/inflammation (e.g., meningitis) [37–42]. Recurrent ophthalmoplegic cranial neuropathy (previously called ophthalmoplegic migraine) is a rare cause of recurrent painful ophthalmoplegia involving the third cranial nerve in the vast majority of cases and is now thought to be an inflammatory cranial mononeuropathy, as evidenced by gadolinium enhancement of the affected oculomotor nerve on MRI [43–45] (Fig. 14.3). This entity is a diagnosis of exclusion, which can only be invoked after other etiologies have been ruled out by appropriate neuroimaging and lumbar puncture; steroids may be helpful if administered promptly. Aneurysmal third nerve palsy is very rare in the pediatric population but has been reported even in prepubescent children [46, 47]. Diabetic microvascular oculomotor nerve palsy has been reported in children, but is extremely rare [48].


Fig. 14.3
Axial T1-weighted MRI with gadolinium demonstrating enhancement of the third nerve (arrow) in a patient with an oculomotor palsy due to ophthalmoplegic migraine
Clinically, oculomotor nerve palsy presents with variable degrees of eyelid ptosis, pupillary dilation, and impaired ocular supraduction, adduction, and infraduction. Ptosis may vary from absent to complete. Levator palpebrae function in the ptotic eyelid is decreased. The pupil may vary from normal (pupil sparing) to mid-dilated (usually 5–6 mm) with no response to light or attempted viewing of a near target. The affected eye tends to be hypotropic and exotropic in primary gaze, though hypotropia may be seen in upgaze with hypertropia in downgaze (“alternating hypertropia”). Complete ptosis with a dilated nonreactive pupil and absent supraduction, infraduction, and adduction is termed a complete third nerve palsy; any findings short of complete palsy constitute a partial third nerve palsy. Cases of congenital palsy often show signs of aberrant regeneration: elevation of the eyelid in attempted adduction or infraduction, or pupillary miosis on attempted adduction or infraduction, among others. If third nerve palsy is suspected, the pupils, eyelids, and ocular motility must all be carefully evaluated, with attention to the pattern of ocular misalignment.
Unless there is a clear, definite etiology backed by the patient’s history or examination findings, all pediatric patients found to have a third nerve palsy should undergo detailed imaging of their brain and orbits (with attention to the path of the involved third nerve), preferably with MRI with and without contrast. Though remote, the possibility of an aneurysm should be ruled out with an MRA. If imaging does not show an explanatory lesion, LP should be performed to rule out a meningitic or inflammatory cause. In children who are 10 or older, a cerebral angiogram should be performed if MRI/MRA and LP are unrevealing, given the potentially devastating consequences of an undiagnosed aneurysm in a patient with a very long expected life span [47]. Amblyopia is very common in children with congenital or irreversible acquired oculomotor nerve palsies, often necessitating patching, multiple strabismus surgeries to achieve alignment in primary gaze, and surgical management of ptosis.
Congenital Fibrosis of the Extraocular Muscles (CFEOM)
The congenital fibrosis syndromes are characterized by bilateral nonprogressive ptosis and ophthalmoparesis, which are present at birth. The extraocular muscle hypoplasia and fibrosis are thought to be the result of congenital cranial dysinnervation [49, 50]. Eight distinct entities have been described to date, CFEOM1A, CFEOM1B, CFEOM2, CFEOM3A, CFEOM3B, CFEOM3C, Tukel syndrome, and CFEOM3 with polymicrogyria, based on phenotypic and genetic features [50]. CFEOM1 consists of bilateral severe ptosis, downward-pointing eyes, and bilateral supraduction deficits and has been associated with absence of the superior division of the oculomotor nerve [49, 50]. CFEOM1 is an autosomal dominant disorder which has been associated with mutations in the KIF21A gene for CFEOM1A and TUBB3 gene for CFEOM1B. On the other hand, CFEOM2 is autosomal recessive and presents with bilateral ptosis, exotropia, and supraduction, infraduction, and adduction deficits. It is caused by a mutation in the PHOX2A/ARIX gene, resulting in bilateral absence of the third and fourth cranial nerves [49, 50]. CFEOM3 is characterized by variable and asymmetric ptosis, variable exotropia, and variable impairment of horizontal and vertical eye movements. The disorder is inherited in an autosomal dominant fashion. CFEOM3A is linked to mutations in TUBB3 or TUBB2B, CFEOM3B is associated with KIF21A mutations, and CFEOM3C is associated with a chromosomal translocation [50]. CFEOM3 with polymicrogyria has been associated with pathologic variants in TUBB2B. Tukel syndrome is an autosomal recessive condition that is characterized by the association of variable ptosis and variable ophthalmoparesis in the third nerve distribution with oligodactyly and oligosyndactyly of the hands. Imaging in congenital fibrosis patients often reveals atrophy of the extraocular muscles [50]. Management generally involves treatment of amblyopia, correction of refractive error, as well as strabismus and ptosis surgery.
Chronic Progressive External Ophthalmoplegia (CPEO) and Kearns-Sayre Syndrome (KSS)
Gradually progressive bilateral ptosis, ophthalmoplegia, and orbicularis oculi weakness are hallmarks of CPEO, which can present at any age. It may begin asymmetrically, or unilaterally, but over time invariably progresses to bilateral, relatively symmetric involvement [51]. Diplopia is rare in CPEO because of the general propensity for symmetric bilateral involvement. Eye movements are often limited in all directions with low saccadic velocities. KSS comprises the association of CPEO appearing before age 20 and pigmentary retinopathy with at least one of the following: ataxia, heart block, or elevated cerebrospinal fluid protein level. Other reported abnormalities in KSS include hypoparathyroidism, diabetes, short stature, dementia, and sensorineural hearing loss [52]. Both CPEO and KSS fall into the category of mitochondrial myopathies and are associated with mitochondrial dysfunction, ragged red fibers on muscle biopsy, and large mitochondrial DNA deletions [51–53]. No effective pharmacological therapy is available, and these patients sometimes require ptosis and strabismus surgery for symptomatic relief [54]. Referral to cardiology and endocrinology is frequently necessary for evaluation and management of the systemic complications.
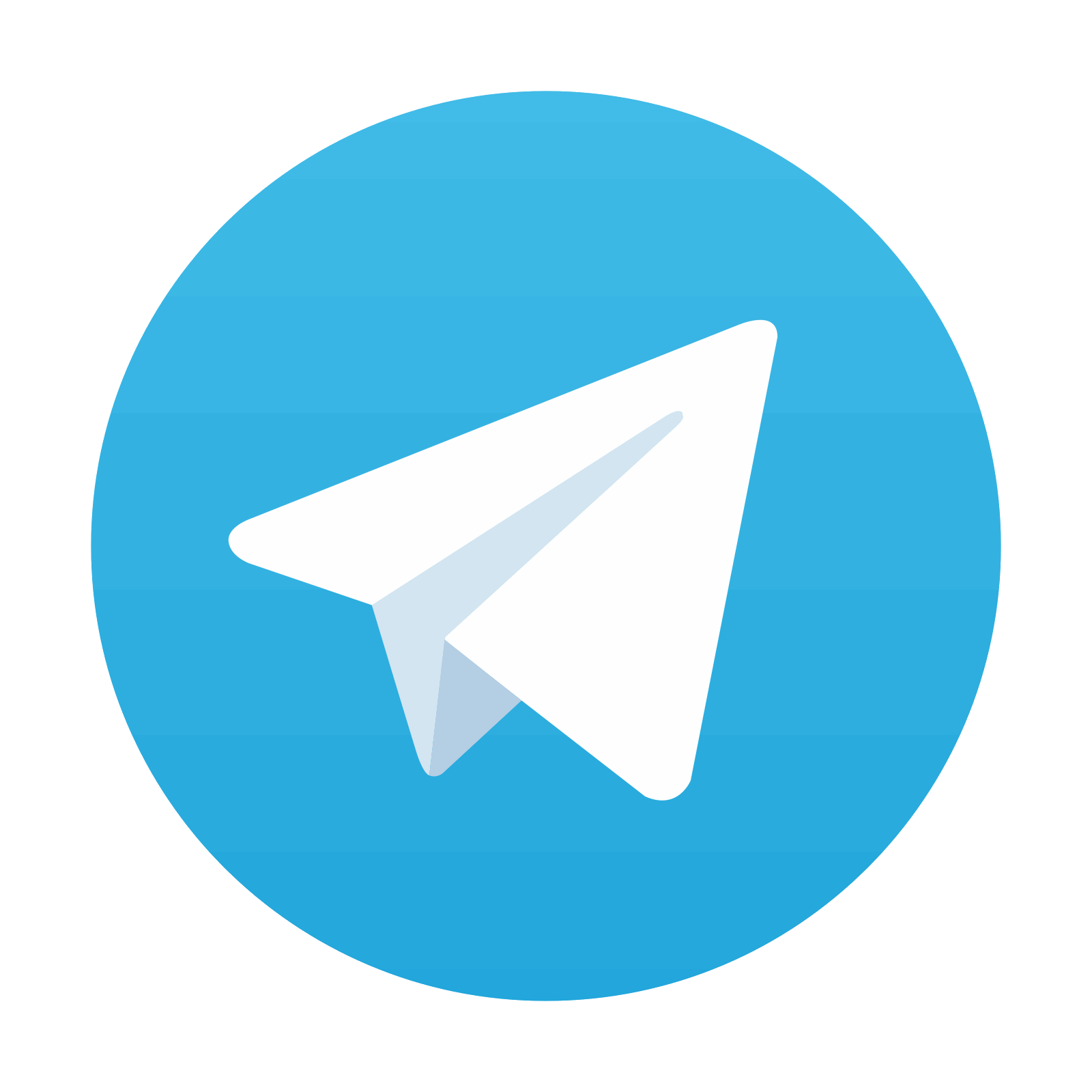
Stay updated, free articles. Join our Telegram channel

Full access? Get Clinical Tree
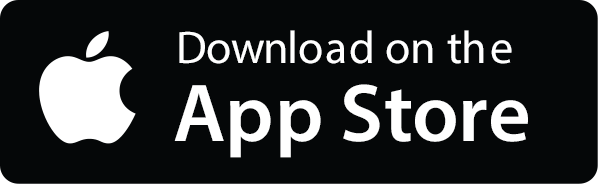
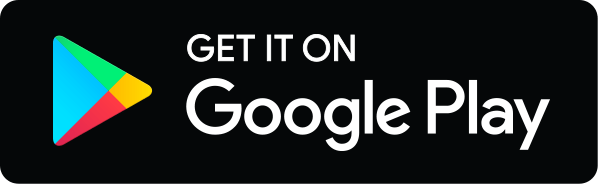