(3)
Beri Eye Care Associates, Portland, OR, USA
The association of central retinal vein occlusion (CRVO) and glaucoma/elevated intraocular pressure (IOP) has long been observed. The first person to note the role of glaucoma in the development of CRVO seems to have been Verhoeff in 1913.
CRVO is a multifactorial disease with several risk factors, i.e., age, systemic arterial hypertension, diabetes, dysproteinemia, and hyperviscosity disorders predisposing an eye to have CRVO develop and other factors precipitating it. Hemi-CRVO is a variant of CRVO. In this condition, the eyes have a congenital abnormality of two trunks of central retinal vein in the optic nerve instead of one.
While CRVO and HCRVO share the same clinical and pathogenetic features, they should be differentiated from major branch retinal vein occlusion. CRVO/HCRVO consist of two distinct varieties: ischemic and nonischemic.
Earlier observations of association of CRVO and glaucoma were based on histopathologic evidence. Subsequent studies based on nonrandomized comparative case series of 674 consecutive patients reported overall prevalence of glaucoma as 9.9 % and of ocular hypertension as 16.2 %.
Elevated Intraocular Pressure in Pathogenesis of CRVO/HCRVO
Verhoeff postulated that probably the increased IOP compresses and collapses the wall of the central retinal vein (CRV), leading to intimal/endothelial proliferation, which he found in his histopathologic studies to be the primary cause. This further leads to stasis of circulation and subsequent thrombosis of the central retinal vein. Others have reported a significant prevalence of exfoliation syndrome with in CRVO compared with control eyes, thought likely due to the high pressures seen in exfoliation. Stasis of circulation caused by elevated IOP might be one of the contributing factors in Virchow’s triad for development of thrombosis.
Ocular Hypotension with CRVO/HCRVO
The association of ocular hypotony in eyes with CRVO is well established since reported for the first time by Moore in 1922. The pathogenesis still remains an enigma. Theoretically, retinal hypoxia and liberation of hypotensive factor by the hypoxic retina leads to fall of IOP. This may be a self-limited problem. However, patients with an ischemic CRVO/HCRVO may develop NVG.
The Take-Home Messages
1.
Patients with CRVO/HCRVO have higher than normal prevalence of glaucoma/OHT.
2.
Presence of hypotony in the affected eye with CRVO/HCRVO can be misleading. Such eyes may still develop NV if they have ischemia.
3.
The author recommends that the fellow eye with elevated pressure/OHT should be treated with ocular hypotensive agents in order to avoid its progression into glaucoma and to prevent development of CRVO/HCRVO.
4.
The eye with CRVO/HCRVO with hypotony needs no further lowering of IOP by ocular hypotensive agents. However, the eye should be monitored periodically for future consideration of glaucoma therapy as IOP tends to equalize over time.
Bibliography
Cursiefen C, Kuchle M, et al. Pseudoexfoliation syndrome in eye with ischemic central retinal vein occlusion: a histopathologic and electron microscopic study. Acta Ophthalmol Scand. 2001;79:476–8.
Hayreh SS. Central retinal vein occlusion. Ophthalmol Clin North Am. 1998;11:559–90.
Hayreh SS, et al. Hemi-central retinal vein occlusion. Pathogenesis, clinical features and natural history. Arch Opthalmol. 1980;98:1600–9.
Hayreh SS, et al. Systemic diseases associates with various type of retinal vein occlusion. Am J Ophthalmol. 2001;131:61–77.
Hayreh SS, et al. IOP abnormalities associated with central and hemicentral retinal vein occlusion. Ophthalmology. 2004;111(1):133–41.
Moore RF. Some observation on intraocular tension in cases of thrombosis of retinal veins. Trans Ophthalmol Soc UK. 1922;42:115–26.
Saatci OA, Ferliel ST, et al. Pseudoexfoliation and glaucoma in eyes with retinal vein occlusion. Int Ophthalmol. 1999;23:75–8.
Verhoeff FH. The effect of chronic glaucoma on the central retinal vessels. Arch Opthalmol. 1913;42:145–52.
Clinical Presentations
The first clinically apparent manifestations of anterior segment NV (pre-NVG) associated with diabetic retinopathy are often tiny red nubbins or nonradial, irregular surface capillaries adjacent to the pupil or iris defects. Early on, these may not become visible at the slit lamp until irritation during examination and gonioscopy or after topical drugs for pupil dilation. They may also become obvious during fluorescein iris angiography (Fig. 18.1). Under the continued influence of VEGF, a fibrovascular membrane erupts onto and covers the iris surface usually emanating from peripupillary capillaries, eventually spreading to and vaulting the scleral spur to arborize anteriorly across the meshwork (Fig. 18.2). Contraction within the growing membrane, which can occur before IOP elevates, often rolls the pupillary edge anteriorly, producing characteristic ectropion uvea (Fig. 18.3). During the intermediate and later phases of evolving NVG, contraction of the angle membrane produces progressive up-ratcheting of the peripheral iris into contact with the meshwork that progresses from the iris recessing in a posterior-to-anterior direction to complete angle closure via peripheral anterior synechia (PAS) formation (Fig. 18.4). Outflow through the meshwork progressively decreases, eventually overwhelming compensatory mechanisms, possibly including decreased aqueous production, and marked elevation of IOP ensues. After angle closure, continuing high IOP with corneal edema and bullous keratopathy provoke cycles of pain and inflammation with eventual resolution by fibrovascular resurfacing of the cornea and ingrowth into the corneal stroma. Eyes that survive until very late stages may develop thick iris membranes with variable obliteration of the anterior chamber by fibrosis. Vascularization along the lens capsule or lens remnants and cyclitic membrane formation often contribute to contracting vitreous organization and total funnel retinal detachment.
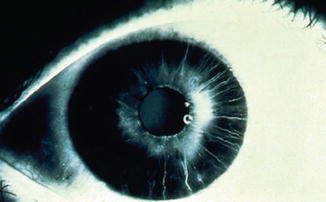
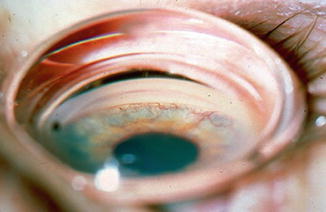
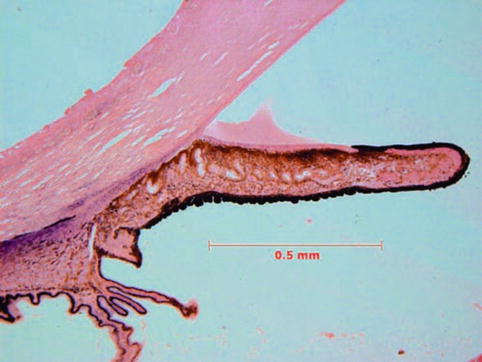
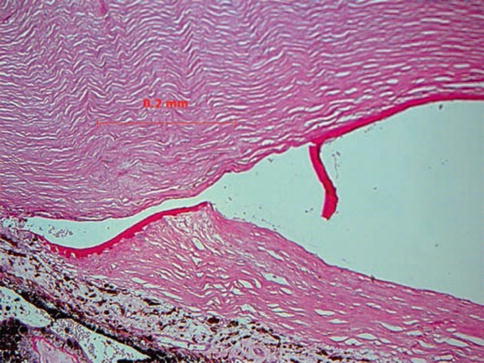
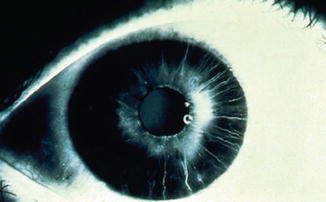
Fig. 18.1
Iris angiogram illustrating early leak from new vessels at pupil margin
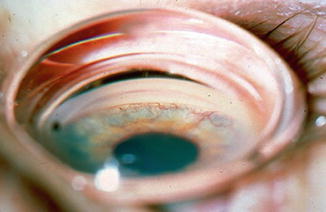
Fig. 18.2
Clinical gonioscopy via Koeppe lens of fully developed rubeosis and angle closure in diabetes
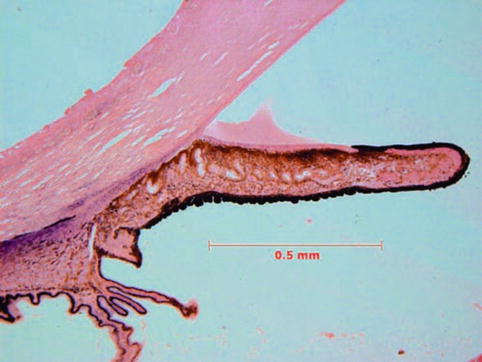
Fig. 18.3
Photomicrograph illustrating ectropion uvea as a consequence of neovascularization (NV) associated with advanced diabetic vitreoretinopathy and retinal detachment. The pupil is everted, bending the sphincter anteriorly due to contraction of a fibrovascular membrane on the iris surface continuous with the same process that has closed the angle by pulling the iris anteriorly (Photomicrograph; hematoxylin and eosin; celloidin embedding, original ×10)
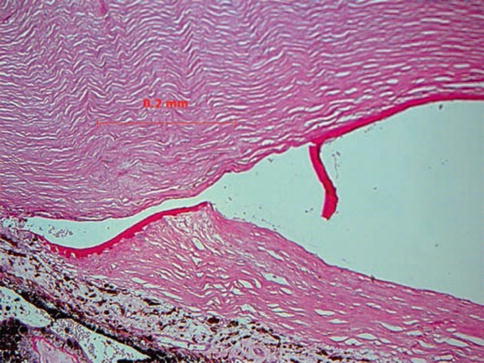
Fig. 18.4
Massive end-stage fibrosis along the anterior iris surface. An artifactually fractured Descemet’s membrane indicates where the “false” angle formed anterior to complete angle closure in diabetes-related neovascular glaucoma (Photomicrograph; periodic acid-Schiff, original ×100)
The contraction accounting for ectropion uveae and PAS formation, in addition to retinal detachment, has long been explained by the presence of a population of myofibroblasts in the fibrovascular membranes, the cytoplasm of which contains arrays of contractile fibrils that react with smooth muscle antibodies (Fig. 18.5) [6, 7]. Myofibroblasts are widely distributed in normal ocular tissues, including the uvea, and can proliferate in reaction to tissue injury or when indirectly stimulated by VEGF. They may also evolve from vascular endothelium and pericytes or quiescent fibroblasts, all also widely distributed in normal ocular tissues.
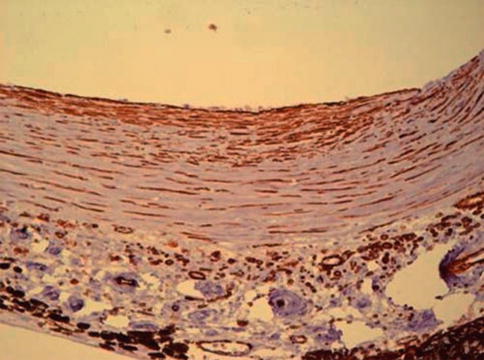
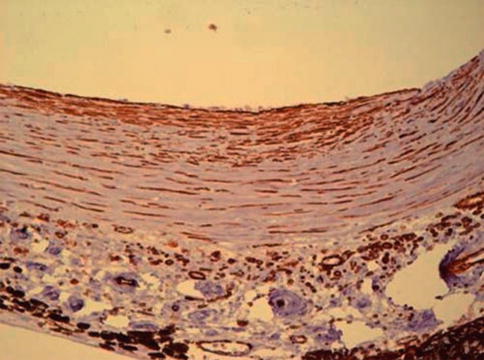
Fig. 18.5
SMA (smooth muscle antigen) immunostaining positive cells are reddish brown within the membrane and along the anterior iris, from same case as in Fig. 18.4 (Photomicrograph, original ×200; SMA [smooth muscle actin] immunostain)
The rate of IOP rise in NVG varies greatly but can be abrupt, leading to a presentation confused with acute primary angle closure and often to laser peripheral iridectomy, seldom indicated as pupillary block is rarely present. Headache and corneal edema with vision decrease are common if IOP rises rapidly. Typical clinical signs of NVG in cases presenting with acute IOP elevation usually include ocular pain, ciliary flush, corneal bedewing, often obvious rubeosis, ectropion uvea, and hyphema. When gonioscopy is possible, variable angle closure with broad peripheral anterior synechiae (PAS) is typical. If gonioscopy is not possible due to corneal opacity, anterior segment imaging with high-resolution ultrasound or optical coherence tomography (OCT) can establish the diagnosis of angle closure. Paracentesis can accomplish rapid, usually transient, IOP decrease and corneal clearing but may provoke angle bleeding and increased hyphema. When IOP is elevated in NVG, abnormal vessels are always present in the angle, usually in several quadrants or circumferentially in diabetes and CRVO (see Sidebar 18.2 ). Neovascularization of the angle may be segmental and limited to a few clock hours anterior to a branch vein occlusion (BVO).
Diabetes
The likelihood of NVG developing in diabetic patients is correlated with glucose control. In the Diabetes Control and Complications Trial (DCCT), 24 % of standard treatment group patients developed NVG over 9 years compared to 8 % in the intensively treated group [8].
A unique and landmark study conducted between 1984 and 1990 in diabetic eyes found that 31 of 100 nonconsecutive diabetic patients (59 males, 41 females) without previous laser therapy had IOPs greater than 21 mmHg in one or both eyes. Standard gonioscopy revealed angle vessels in 30/100 (30 %), but gonioangiography detected abnormal angle vessels in 56/100 (56 %). Importantly, NV was present only in the angle in 20 %, reinforcing the importance of gonioscopy when evaluating diabetic eyes (Fig. 18.6a, b) [9].
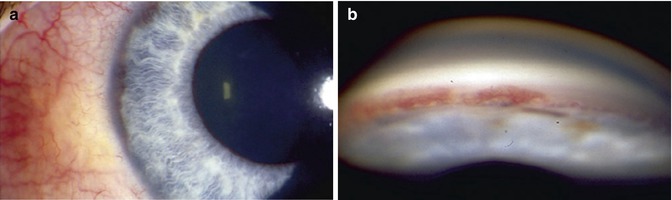
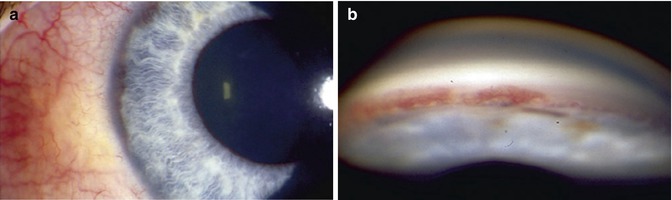
Fig. 18.6
(a) Clinically obvious angle neovascularization in type I diabetes without pupillary neovascularization being evident. (b) Clinically obvious angle NV in type I diabetes without pupillary NV being clinically evident
A history of diabetes and typical retinopathy in the same or opposite eye would strongly support diabetes-related NVG. Painless sudden decrease in vision 60–90 days prior would be typical of CRVO. An open angle in the opposite eye essentially rules out primary angle closure in the affected eye, the most common misdiagnosis when NVG presents with acutely elevated IOP, in turn leading to the most common mistreatment: laser iridectomy. The most florid examples of rubeosis follow CRVO in which relatively huge vessels can course irregularly across the iris surface.
Sidebar 18.2. Central Retinal Vein Occlusion and Monitoring Risk of Neovascular Glaucoma
John D. Hyatt4 , Sarwat Salim5 and Peter A. Netland6
(4)
Hamilton Eye Institute, University of Tennessee Health Science Center, Memphis, TN, USA
(5)
Hamilton Eye Institute, University of Tennessee, Memphis, TN, USA
(6)
Peter A. Netland, MD, PhD Department of Ophthalmology, University of Virginia School of Medicine, Charlottesville, VA, USA
Central retinal vein occlusion (CRVO) is a well-known cause of vision loss, not only due to characteristic acute retinal changes but also due to its sequelae, such as neovascular glaucoma (NVG) and macular edema. In an effort to improve outcomes, there has been much discussion regarding identification of patients with CRVO who are at greatest risk of developing NVG for timely intervention.
CRVO accounts for about one-third of cases of NVG. Not only can CRVO lead to NVG, but elevated intraocular pressure may play a role in its etiology. Neovascularization (NV) of the iris or angle after CRVO is a critical clinical finding that can result in NVG if not recognized and treated promptly. In the Central Vein Occlusion Study (CVOS), 16 % of the eyes developed NV. Hayreh et al. found that approximately 10 % of eyes with CRVO developed NV, while the incidence increased to 45 % in eyes with prominent retinal ischemia. The amount of retinal ischemia seen on fluorescein angiography (FA) has been shown to correlate with development of anterior segment NV. This link is explained by the elaboration of VEGFs by ischemic retina, coinciding with formation of NV. Quantifying retinal ischemia by FA to assess risk of anterior segment NV at times can be problematic because transmission blockage secondary to acute hemorrhage can limit accurate delineation of ischemic retina. There also is controversy in the literature surrounding what terminology and threshold to use for classifying a patient as having an “ischemic” or “non-perfused” CRVO. Though the Central Retinal Vein Occlusion Study (CVOS) used ten or more disc areas of retinal non-perfusion as a cutoff, a significant percentage of patients initially classified as perfused later developed NV. Subsequently, 30 disc areas of non-perfusion at presentation emerged as a more accurate predictor of development of NV. Of the eyes in the CVOS where retinal hemorrhages initially precluded adequate classification of retinal ischemia on presentation, 83 % eventually demonstrated evidence of significant ischemia.
Visual acuity also predicts the likelihood of developing NV. Overall, the CVOS showed a linear correlation between decreasing Snellen visual acuity and risk for NV. In the subset of patients who presented within 1 month after the initial insult, 56 % of the eyes with visual acuity less than 20/200 manifested NV during the study. This finding proved more predictive than any other variable, including non-perfusion on FA.
Neovascularization and associated glaucoma are usually seen within the first few months after the occlusion, giving rise to the commonly used term “90-day glaucoma.” In the CVOS population, the steepest rise in NV formation was during the first 4 months. While analyzing the rate of NV occurring in patients with ischemic CRVO, Hayreh et al. noted a similar precipitous rise in the first few months. In both of these studies, the risk of NV formation stabilized after about 8 months, with a very slow increase in the following years. The rapid development of anterior segment NV in some patients with CRVO makes the first few months after onset a critical time for monitoring and intervention.
At the conclusion of the CVOS, the investigators recommended frequent follow-up in the first 6 months after onset with attention paid to recognizing NV in the anterior segment by meticulously examining the undilated pupillary border and performing gonioscopy, since NV might develop in the anterior chamber angle first. Their schedule was approximately monthly, with potentially longer spacing between appointments for stable or improving eyes with visual acuity better than 20/200 on presentation. Notably, they found that prophylactic panretinal photocoagulation (PRP) did not prevent formation of NV in susceptible eyes. However, they conducted PRP at the earliest signs of NV in an effort to prevent development of NVG, resulting in regression of NV in the first month in 56 % of previously untreated eyes. The mechanism by which PRP works is unclear. It is believed to reduce retinal oxygen demand, reducing hypoxic stimulus and subsequent release of VEGF.
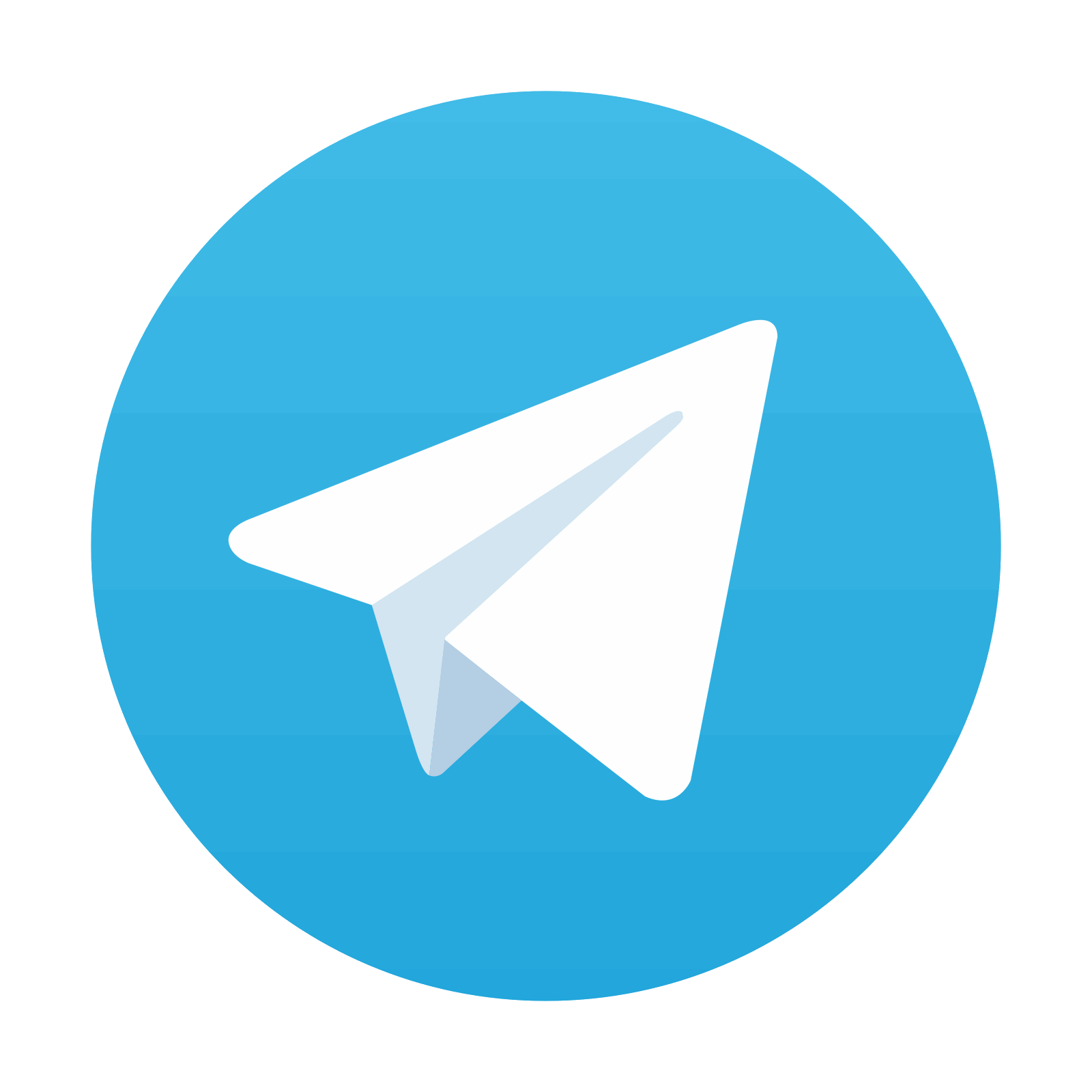
Stay updated, free articles. Join our Telegram channel

Full access? Get Clinical Tree
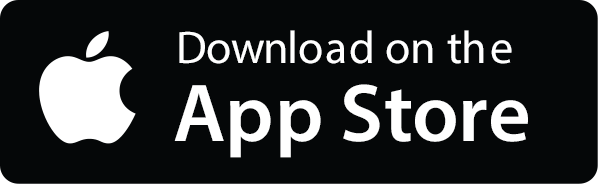
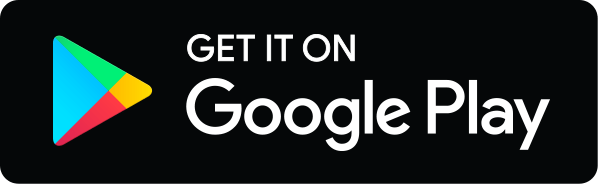