Purpose
To examine a female subject, her father, and a brother harboring a missense mutation of the retinitis pigmentosa 1-like 1 ( RP1L1 ) gene, over 2 years of follow-up.
Design
Observational case series.
Methods
setting : Fondazione G.B. Bietti IRCCS, Rome, Italy. study population : RP1L1 family members and controls. main outcome measures : Images of the cone mosaic acquired with an adaptive optics retinal camera, spectral-domain optical coherence tomography (SD OCT), and full-field and multifocal electroretinography (mfERG).
Results
In the proband, best-corrected visual acuity (≤0.7 logMAR) was stable in both eyes during follow-up, though analysis of adaptive optics images showed decreased cone density in the central 9 degrees from the fovea with respect to controls ( P < .05) and cone density loss in the parafoveal area (2 degrees; <12%–16%) during follow-up. Texture analysis of SD OCT images identified abnormalities of the ellipsoid zone in the central 7 degrees, while mfERG response amplitudes were reduced only in the central 5 degrees relative to controls. In the proband’s father, who had 0.0 logMAR visual acuity, significant cone loss was found in the central 7 degrees from the fovea ( P < .05); abnormal SD OCT and mfERG values with respect to controls were found in corresponding retinal areas. No defects in the cone structure and function were found in the proband’s brother, who had 0.0 logMAR visual acuity.
Conclusions
Occult macular dystrophy was diagnosed based on genetic and multimodal ophthalmic findings. The quantitative assessment of photoreceptor survival or loss, based on analysis of adaptive optics retinal images, was valuable to monitor disease progression at a cellular level.
Occult macular dystrophy is a rare macular dystrophy presenting with progressive bilateral central visual loss, normal fundus appearance, and normal fluorescein angiography even in advanced stages. For this reason, occult macular dystrophy has been frequently misdiagnosed as normal-tension glaucoma, retrobulbar optic neuritis, or amblyopia. Several authors have hypothesized that this retinal dystrophy may include various disease entities with different genetic bases and autoimmune pathophysiology. Indeed, patients with diagnosis of occult macular dystrophy have shown different clinical and functional signs. Several putative pathogenic variants of the retinitis pigmentosa 1-like 1 ( RP1L1 ) gene (p.R45W, p.Arg.45Trp, p.S1199C, p.Gln2311Pro, p.Asp1425His, p.Ser676Cys and p.W960R; available at http://www.ncbi.nlm.nih.gov/projects/SNP/ ) have been reported in sporadic cases or in families, with autosomal dominant inheritance, in association with the occult macular dystrophy phenotype. It is therefore well accepted that several independent mutations can lead to the occult macular dystrophy phenotype ( Supplemental Text , available at AJO.com ). New questions have arisen around this rare inherited retinal disease: whether 1 or more genes are responsible for the variable phenotype and whether the disease involves only the macula or even larger retinal areas.
In this work, we aim to track structural and functional retinal changes in a young female subject harboring a missense mutation of the RP1L1 gene over 2 years of follow-up. In addition, her family members underwent genetic and ophthalmic screening.
Methods
Study Population
A 23-year-old woman (proband) with progressive bilateral visual loss for 6 years was referred to our Clinical Trials Center in September 2012. She had normal ophthalmoscopic findings, normal fluorescein angiography and indocyanine green angiography, normal fundus autofluorescence, normal full-field electroretinogram for cone and rod components, normal spinocerebral magnetic nuclear resonance imaging, and negative lumbar puncture (to exclude oligoclonal band status for the diagnosis of multiple sclerosis). The proband, her parents (father, 57 years old; mother, 52 years old) and brother (27 years old), and her paternal grandfather (78 years old) were recruited in a study protocol approved by the local Ethical Committee (Azienda Sanitaria Locale Roma A) and gave written informed consent after a full explanation of the procedures. All research procedures described in this work adhered to the tenets of the Declaration of Helsinki.
The proband and her family, except for her grandfather, underwent comprehensive ophthalmologic examination including best-corrected visual acuity (BCVA), contrast sensitivity function testing using a Pelli-Robson chart (Precision Vision, Lasalle, Illinois, USA), full-field electroretinogram (ERG, Erev 2000; Lace Elettronica, Pisa, Italy), multifocal electroretinogram (mfERG; VERIS Clinic 4.9; Electro-Diagnostic Imaging, San Mateo, California, USA) recorded according to International Society for Clinical Electrophysiology of Vision (ISCEV) standards, MP1 microperimetry (Nidek Technologies, Padova, Italy), and ocular biometry using the IOL Master (Carl Zeiss Meditec AG, Hennigsdorf, Germany). Monocular administration of color test was performed using Ishihara pseudoisochromatic plates (24 plates edition, Kanehara Trading Inc, Tokyo, Japan) in natural daylight. An adaptive optics flood illumination retinal camera ( rtx1 ; Imagine Eyes, Orsay, France) was used to acquire images of the photoreceptor mosaic. In addition, combined scanning laser ophthalmoscopy and spectral-domain optical coherence tomography (SD OCT) was performed using a Spectralis device (Heidelberg Engineering GmbH, Heidelberg, Germany); fundus autofluorescence was also acquired with the Spectralis. The proband was evaluated over 2 years of follow-up (from September 2012 to July 2014); her family members underwent ophthalmic examination only in February 2014. Blood samples were collected from the whole family and genomic DNA was extracted from peripheral blood lymphocytes using conventional methodologies for genetic testing.
Five healthy volunteers (age, 23.3 ± 1.2 years) were recruited in the study as controls for adaptive optics and SD OCT data acquired from the proband and her brother. Normative data by Curcio and associates were used as controls for adaptive optics data acquired in the proband’s parents. In addition, 2 populations of 5 healthy subjects each (1 population with age ranging from 20 to 30 years and the other with age ranging from 50 to 60 years) were used as controls for multifocal electroretinogram testing. Control subjects had no history of systemic or ocular diseases and no previous eye surgery.
Genetic Testing by Molecular Analysis
Genomic DNA was isolated from peripheral blood using a commercial kit (QIAGEN Blood Pure Gene Gentra, QIAGEN S.r.l., Milan, Italy). A panel of 61 candidate genes known to be involved in retinal dystrophies was analyzed by next-generation sequencing (NGS; Illumina Inc, Hayward, California, USA). Purified DNA was used to synthesize an NGS library according to the protocol Illumina Custom Amplicon (available at http://products.illumina.com/products/truseq_custom_amplicon.html ) and was sequenced on Illumina miSeq with protocol PE 2×250. The reads were aligned to the reference genome (UCSC/hg19) using the software provided by the manufacturer. Post-processing was performed using the Genome Analysis Toolkit (GATK) package. Briefly, we performed a local realignment of in-del regions using the indelRealigner tool; the base qualities have been recalculated with the BaseRecalibrator tool and finally the variants have been identified using the UnifiedGenotyper tool (standard parameters). The selection of variants of clinical interest was made using the following filters: (1) variant with minor allele frequency <1%; (2) variant localized in the coding sequence (exon ± 8 nucleotides); (3) variant previously reported in the literature (The Human Gene Mutation Database) as pathogenic; (4) variant type (nonsense, splicing, non-in-frame deletion or insertion > missense > in-frame deletion or insertion); and (5) new missense variant defined as damage in silico predictions (LJBSIFT, POLYPHEN2, LRT, Mutation Taster, MutationAssessor). The selected variant in the RP1L1 gene was confirmed by Sanger sequencing with a CEQ 8000 automated sequencer (Beckman Coulter, Milano, Italy) in the proband and her family members starting from a new genomic DNA extraction.
Acquisition and Analysis of Adaptive Optics Retinal Images
The rtx1 was used to acquire images at several retinal locations covering an area of 8 × 20 degrees around the fovea. Each image sequence consisted of 40 frames acquired by illuminating a retinal area subtending 4 degrees of visual angle. A proprietary program provided by the manufacturer was used to correct for distortions within frames of the raw image sequence and to register and frame-average in order to produce a final image with enhanced signal-to-noise ratio prior to further analysis, as described in previous studies. The final images were montaged with i2k Retina Pro (DualAlign LLC, Clifton Park, New York, USA) using the “retinal montage tool.” The corrected magnification factor was calculated for each eye in order to correct for the differences in optical magnification and thus retinal image size between eyes.
In the proband, the follow-up adaptive optics montages were aligned to one another using a cross-correlation algorithm in order to determine their relative position displacements using the “template matching plugin” (available at https://sites.google.com/site/qingzongtseng/template-matching-ij-plugin ) in Image J ( http://imagej.nih.gov/ij , National Institutes of Health, Bethesda, Maryland, USA). The baseline image (acquired in September 2012) was used as reference and a template of image features was selected on it in order to improve the alignment algorithm precision. This procedure ensured that the analysis of cone metrics was from exactly the same retinal location at each examination.
The local analysis of density, spacing, and packing arrangements of cones was performed on 64 × 64-μm sampling areas every 1.0 degree along the temporal meridian, from 1 to 9 degrees from the foveal center. The image cone labeling process was performed using an algorithm implemented with the image processing toolbox in Matlab (The Mathworks Inc, Natick Massachusetts, USA), as previously described. After automated cone identification, 2 expert investigators (M.L. and G.L.) reviewed each area and manually identified cones that they agreed to be missed or selected in error by the algorithm. The x-y coordinates of the cones were then used to calculate density, spacing, and packing arrangements of cones. Cone counts were converted into local densities by calculating their number per square millimeter (cones/mm 2 ). The center-to-center cone distance (μm) was calculated using the nearest-neighbor distance distribution (NND). For each location, the average NND was determined by calculating the average of the minimum distances from the center of that cone to the centers of 6 neighboring cones in the mosaic. Voronoi diagrams were computed to analyze the preferred cone packing arrangement, as previously described. The Voronoi tessellation was implemented by the Matlab Voronoi function using the x-y centroid coordinates of the labeled cones.
Acquisition and Analysis of Spectral-Domain Optical Coherence Tomography Images
The SD OCT images were acquired using a volume scan 10 × 15 degrees in the high-resolution modality. In the proband, the SD OCT images were acquired using the “follow-up scan protocol” by Spectralis that automatically aligns the scans across follow-up examinations during acquisition. The baseline scans (September 2012) were used as reference. An automated algorithm was developed in Matlab to segment the SD OCT retinal scans between the external limiting membrane and the inner edge of the retinal pigment epithelium (ie, the interdigitation zone). A thickness map of the photoreceptor layer was developed and its thickness was evaluated across the foveola (0.25 mm diameter) and 3 concentric zones centered on the foveola, such as the parafovea (0.26–1.55 mm), the perifovea (1.56–3.80 mm), and the mid-periphery (3.81–4.20 mm).
Texture analysis of SD OCT scans was performed in order to track potential disruptive changes of the ellipsoid zone during follow-up. This analysis was performed using the Gray Level Co-occurrence Matrix. Before calculating the Gray Level Co-occurrence Matrix, each SD OCT image was normalized by histogram equalization. In this study, the Gray Level Co-occurrence Matrix was calculated considering 32 gray levels and 2 specific spatial positions of pairs of neighboring pixels: the horizontal direction (0 degrees) and the vertical direction (90 degrees). Thereafter, the correlation was calculated as follows:
C o r r e l a t i o n = ∑ i , j ( i − μ i ) · ( j − μ j ) · P i j σ i · σ j
Acquisition and Analysis of Multifocal Electroretinogram Recordings
The multifocal stimulus, consisting of 61 scaled hexagons, was displayed on a high-resolution, black-and-white monitor (size: 30 × 30 cm) with a frame rate of 75 Hz. The array of hexagons subtended 20 degrees of visual field; each hexagon was independently alternated between black (1 cd/m 2 ) and white (200 cd/m 2 ) according to a binary m-sequence (99% contrast). The luminance of the monitor screen was 100 cd/m 2 . A small red target (0.5 deg) was placed in the center of the stimulation field to maintain a stable fixation and the eye’s position was monitored by a video camera. Trial lenses were used when necessary. The pupils were pharmacologically dilated with 1% tropicamide, the cornea was anesthetized with 1% dicaine, and the mfERGs were recorded binocularly. The mfERGs were recorded between an active electrode (Dawson Trick Litzkow bipolar contact electrode) and a reference electrode (Ag/AgCl skin electrode) placed on the correspondent temporal side of the frontal lobe. An Ag/AgCl skin ground electrode was placed at the center of the forehead (inter-electrode resistance <3 kΩ).
The signal was amplified (gain 100 000) and filtered (band pass 1–100 Hz) by BM 6000 (Biomedica Mangoni, Pisa, Italy). After automatic rejection of artifacts by VERIS Clinic 4.9 software (Electro-Diagnostic Imaging), we examined the first-order kernel response, K1. The mfERG signal-to-noise ratio was estimated following the methodology shown by Hood and Greenstein. In this study, we analyzed the averaged response obtained from 5 concentric annular retinal regions (rings) centered on the foveola. The N1-P1 response amplitude was analyzed from 0 to 2.5 degrees (ring 1, R1), 2.5 to 5 degrees (ring 2, R2), 5 to 10 degrees (ring 3, R3), 10 to 15 degrees (ring 4, R4), and 15 to 20 degrees (ring 5, R5). For each averaged response, we evaluated the response amplitude (nV/deg 2 ) between the first negative peak, N1, and the first positive peak, P1, and the implicit time (ms) of the first positive peak (P1).
Statistics
Statistical analysis was performed using the SPSS software (version 17.0; SPSS Inc, Chicago, Illinois, USA). The 95% confidence intervals (CI) of control data, defined as mean ± 2 standard deviations, were plotted to define the normal ranges with which to compare SD OCT and mfERG data from the proband and her family members. In the proband, the Steel-Dwass test was used to compare the differences among data acquired at different follow-up examinations and between the proband and controls. The Wilcoxon signed rank test was used to statistically compare the adaptive optics data between the proband’s father and Curcio’s control data. Differences with a P value of .05 or less were considered statistically significant.
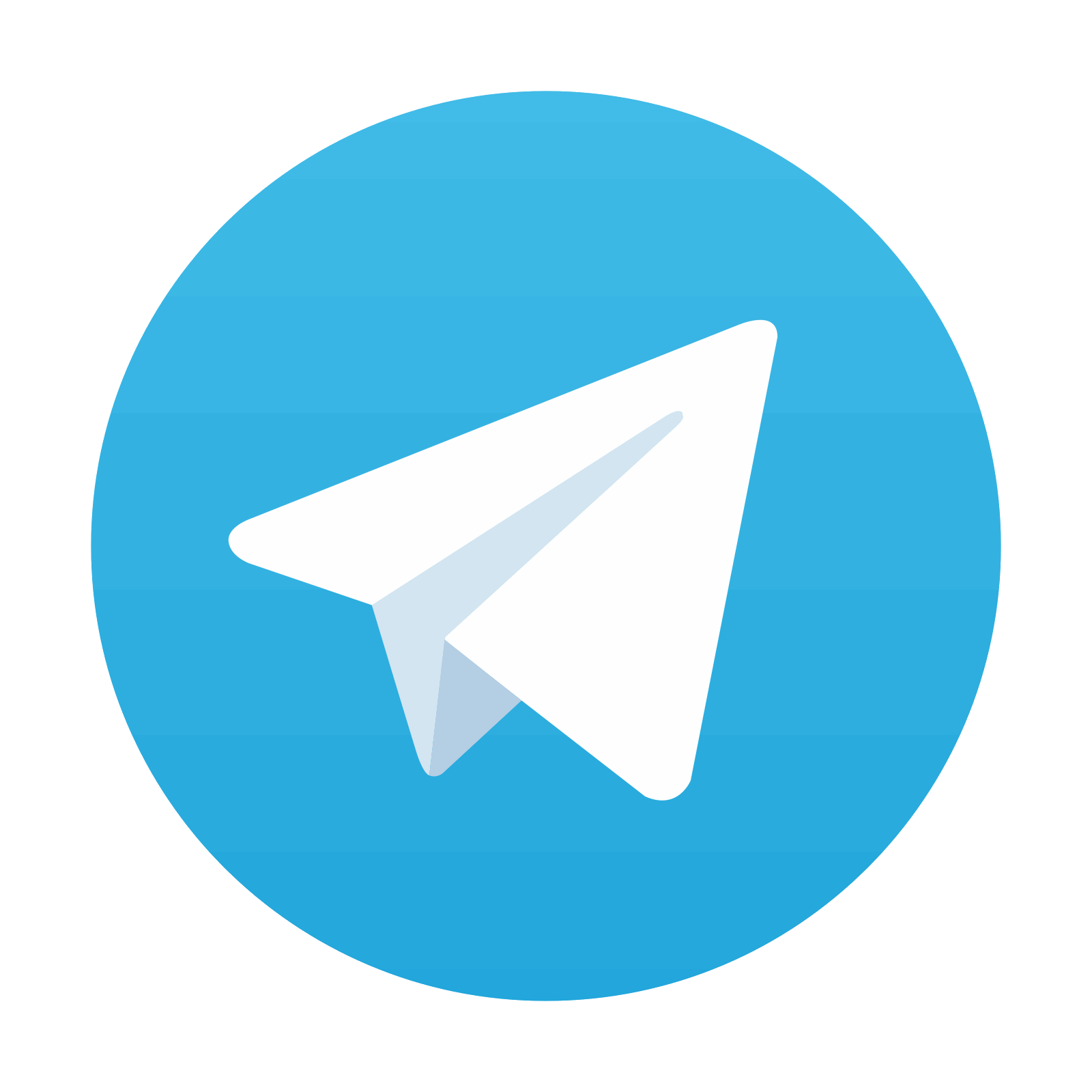
Stay updated, free articles. Join our Telegram channel

Full access? Get Clinical Tree
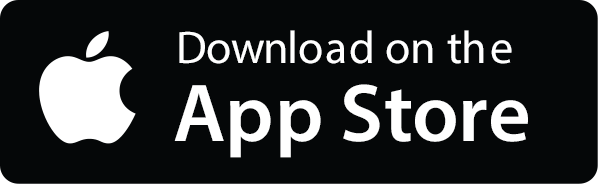
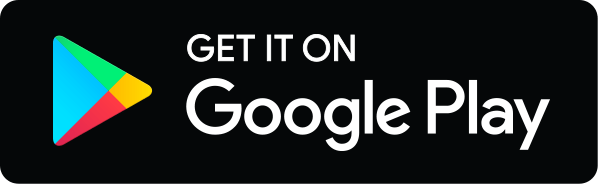