Fig. 16.1
(a) IHC. Schematic showing immunohistochemistry (IHC) methods. Antigen on the left is coupled with a fluorescently labeled primary antibody, which emits a signal when stimulated with light; antigen on the right is coupled with an enzymatically labeled primary antibody, which emits a colored (chromogen) signal when chemically activated. (b) Direct and indirect IHC methods . Graphic representation of direct and indirect antigen detection methods in immunohistochemistry (IHC). The direct method utilizes a labeled primary antibody to bind directly to an antigen, represented on the left, while the indirect method employs labeled secondary antibodies to bind with the unlabeled primary antibody-antigen complex, seen on the right. The direct method results in 1:1 signaling antibody-antigen complex. The signal in the indirect method is amplified since multiple secondary antibodies may bind to the primary antibody-antigen complex
The antibody can be directly conjugated to the signaling molecule or a secondary antibody may be employed, referred to as direct and indirect antigen detection methods, respectively (Fig. 16.1b) [2]. The direct method involves a single staining step, where a labeled antibody binds directly to the antigen. The indirect method, on the other hand, involves two tiers of antibodies: an unlabeled primary antibody that binds to the specific antigen directly and a secondary antibody conjugated to an enzyme or fluorophore that couples with the primary antibody. The indirect method is often preferred because the signal is amplified by the coupling of several secondary antibodies to one primary antibody [5]. The direct method results in a less robust signal due to the 1:1 binding ratio between antibody and antigen but is simpler and more rapid. After IHC staining, a second counterstain such as hematoxylin or DAPI (4′,6-diamidino-2-phenylindole, dihydrochloride) often is performed to highlight or provide context for the IHC stain [2].
Applications
An extensive number of IHC markers are available [2]. A few common ones and their uses are listed in Table 16.1. Improvements in technique and buffers have led to better exposure of antigens and improved antibody-antigen coupling [2, 6, 7]. New IHC stains continue to be added as novel markers are discovered, some of which have reshaped our understanding of tumors [2, 5]. For example, neoplasms previously diagnosed as hemangiopericytoma on morphologic grounds have been reclassified by the World Health Organization (WHO) as solitary fibrous tumors (SFT), partially due to the discovery of the NAB2-STAT6 fusion gene [8, 9]. This fusion gene is easily detected by the nuclear expression of the carboxy terminal part of STAT6 on IHC staining [9]. The discovery of STAT6 and its expression in hemangiopericytomas have led a new understanding and reclassification of this tumor.
Immunohistochemistry (IHC) markers | Target |
---|---|
S-100 | Neural crest lineage |
CD3, CD5 | T-cells |
CD20, CD79a | B-cells |
SMA (smooth muscle actin) | Smooth muscle actin |
DES (desmin) | Striated muscle (intermediate filament) |
AE1/3 (pankeratin marker) | Cytokeratin marker |
HMB45 (human melanoma black), melan A, MITF (microphthalmia transcription factor) | Melanocytes |
NSE (Neuron specific enolase) | Neuroendocrine cells and tumors |
Ki-67 | Proliferating cells (G1, S, G2, and M cell cycle phases) |
IHC can also predict a tumor’s response to treatment, as illustrated by the expression of erythrocyte-type glucose transporter protein Glut-1 expression by capillary hemangiomas [10, 11]. Capillary hemangiomas are common vascular anomalies in the pediatric population that typically regress with age. In certain cases, however, early treatment is required to avoid long-term complications, such as amblyopia [12]. Beta-blockers have been successfully shown to cause regression of these lesions [13]. However, distinguishing capillary hemangioma from other vascular lesions, such as lymphatic or venous malformations or pyogenic granuloma, may be difficult based on clinical and morphological examination alone [10]. Positive expression of Glut-1 can help to confirm the histopathologic diagnosis of capillary hemangioma and, hence, its likely response to beta-blockers (Fig. 16.2) [10, 11].


Fig. 16.2
Glut-1 IHC . Infantile hemangioma composed of benign proliferation of capillary-sized blood vessels lined by somewhat plump endothelial cells and with distinct lumen. No atypia or mitotic figures noted within the blood vessels or stroma. Low (a) and high (b) power magnifications shown. Immunohistochemistry (IHC) with Glut-1 highlights lesional endothelial cells (c) (Courtesy of Chrystalle Katte Carreon, MD; Department of Anatomic Pathology at The Children’s Hospital of Philadelphia; Philadelphia, PA)
Limitations
Despite its widespread use, IHC has some limitations. Enhancements to antibody-antigen signal may result in nonspecific binding and high background signals [2, 7]. Tumors, particularly of uncertain lineage, may also display atypical patterns of antigen expression [7]. The latter can mislead the pathologist, resulting in an erroneous diagnosis. Consequently, IHC stains must be carefully selected, and staining patterns must be rationally assessed to attain the correct diagnosis.
Flow Cytometry (FC)
FC has occupied many roles in research and medicine since devised in 1953 [14]. The introduction of fluorescence in 1968 [15] and multiple lasers in 1978 [16] expanded its domain in pathology. Currently, it is used most commonly in the diagnosis of blood-based disorders and cancers [17].
Method
Similar to IHC, FC characterizes a specimen by antigen-antibody interactions [17]. Instead of utilizing tissue sections, FC detects antigens or molecules suspended in liquid form. Typically, whole cells are subjected to FC, which is illustrated in Fig. 16.3. A sample is incubated initially with a panel of fluorescently labeled antibodies. Cells then travel in a cell-free buffer (sheath fluid), forming a single file of cells that pass through a laser. The signal, or emitted light, is measured at two angles, forward-scattered light (FSC) and side-scattered light (SSC), which helps characterize cell type. In addition, if a fluorescently labeled antibody is present, the laser or a series of lasers excites the fluorophore, which emits a signal that is detected by the fluorescence measuring station.


Fig. 16.3
FC. Illustration demonstrating flow cytometry (FC). Single-cell suspension incubated beforehand with a panel of fluorescently labeled antibodies travels through a sheath fluid. Individual cells pass through a laser, and forward and side-scattered lights are recorded. Fluorescent channels also record the fluorescent signals emitted from the antibodies attached to each cell
The data obtained is referred to as acquisition [17]. By adjusting the parameters, a desired antigen pattern can be selected and plotted graphically. Specific regions or traits can be aggregated by creating certain selection extractions, denoted as “gates.” Improvements in computational methods now offer innovative gating strategies that assist in identifying rare, obscure populations [18].
Applications
Modern flow cytometers are able to analyze thousands of particles every second [17]. They usually have multiple lasers and fluorescence detectors, which allow for multiple antibody labeling and broader characterization. One of the most common applications of FC in pediatric ophthalmology is diagnosis of a lymphoproliferative neoplasm, such as acute myeloid leukemia (AML) [19]. AML can present with extramedullary involvement of the orbital tissues, known as myeloid sarcoma. The differential diagnosis includes tumors such as neuroblastoma or inflammatory conditions such as idiopathic orbital inflammatory syndrome (IOIS). FC can help distinguish AML from these other entities by its distinctive FC expression pattern (Fig. 16.4).


Fig. 16.4
Flow cytometric scatter plots of peripheral blood demonstrating immunophenotype typical of AML: CD13+, CD33(dim/var)+, CD34+, CD117(dim/var)+, HLA-DR+, MPO(small subset)+, and TdT- (Courtesy of Dale M. Frank, MD; Department of Pathology at the Hospital of the University of Pennsylvania; Philadelphia, PA)
Cell sorting is another feature of FC. Fluorescence-activated cell sorting® (FACS) is a trademarked name that has been generalized to most FC cell sorters [20]. It allows a heterogeneous mixture of cells to be sorted based on specific light scattering and fluorescent signals. The value of cell sorting recently has been underscored by the development of personalized treatments, such as peripheral blood stem cell transplants and T-cell-based gene therapy [17, 21, 22]. Some other instruments are able to capture digital images of individual cells. This allows the localization of fluorescent signals within or on the surface of cells, which can provide insight into spatial protein expression and cellular protein-protein interactions [23, 24].
Limitations
Despite the many advantages of FC, there are shortcomings. For solid tissue, FC requires disruption of the tissue to create single-cell preparations [17]. This precludes examination of the specimen’s architecture, as well as the relative location of populations of cells in the tissue. Accordingly, surgical margins are nearly impossible to assess. While IHC employs sections of FFPE, fresh tissue is preferred for FC, limiting the use of fixed, frozen, and archival tissue [17]. Results are aggregated as well, which prohibits analysis of cell-cell interactions [17]. Lastly, analysis must be performed by an experienced FC pathologist due to the computational analysis that is necessary after acquisition [25].
Summary
Although the fundamental approaches to IHC and FC are analogous, both techniques have distinct advantages and disadvantages. IHC can be performed on FFPE sections, which is the customary method of preparing specimens. As a result, the submitting physician typically does not need to notify the pathologist in advance if IHC analysis is required. In addition, the pathologist has a multitude of antibodies at his or her disposal. As long as the tissue in the paraffin block is not exhausted, a variety of IHC stains can be performed. FC, on the other hand, is best performed on fresh tissue and requires a relatively large number of cells. As a result, proper sampling and handling of the specimen requires advanced notification by the submitting physician. However, the advantage of FC is the rapid and simultaneous assessment of a variety of antigens within a large population of cells.
Diagnostic Tools for Genetics and Genomics
Fluorescence in Situ Hybridization (FISH)
While the first two methods detect differences in cellular protein expression, FISH is a diagnostic tool that identifies and localizes genomic sequences [26]. The initial in situ hybridization experiments in 1969 utilized radioactivity to detect RNA-DNA hybridization [27]. Most recent modifications incorporate fluorescent probes, as well as probes that can hybridize to DNA, RNA, and RNA variants such as messenger RNA and microRNA [26, 28].
Method
As the name implies, FISH is based on the hybridization of a detectable probe to a desired genetic target [26]. Hybridization utilizes the precise coupling of nucleic acids: cytosine to guanine and adenine to thymine (or uracil in RNA). The probe usually is a cloned segment of DNA or RNA that is labeled with a fluorophore. Depending on the target, probes can vary in length. The sample and probes may need to be denatured to disrupt any hydrogen bonds between the bases, thereby priming the sample (Fig. 16.5). If the sequence of a probe matches the sample, hybrids form between the probe and its genetic target, while the rest washes away. A fluorescent signal is detected using a fluorescent microscope. Various specimen preparations can be assessed with FISH, including FFPE sections, frozen sections, and fine-needle aspirates.


Fig. 16.5
FISH. Diagram exemplifying fluorescent in situ hybridization (FISH). Target sequence and fluorescently tagged probe undergo denaturing to separate strands. The probes are incubated with the sample. If the probe and sample express complimentary sequences, the probe anneals to the template and extends with the help of polymerases. Signals are then detected under fluorescent microscopy
Applications
FISH can be used for multiple purposes, including diagnosis, prognostication, and disease monitoring. A traditional application is metaphase chromosome analysis [26]. Previous techniques for karyotyping were cumbersome and time-consuming. With multifluor FISH, the process is made easier with the employment of probes that hybridize with entire chromosomes, painting each chromosome a unique fluorescent color. Deletions, duplications, and translocations are subsequently identified by their fluorescence pattern.
While this method is advantageous over prior ones, it still precludes detailed analysis of specific sequence rearrangements and alterations. Therefore, smaller probes that are specific to a particular locus are utilized for finer scrutiny of sequence variations [26]. For example, FISH can be used to detect clinically significant chromosomal translocations such as the Philadelphia chromosome (Ph) [29]. One of the first proofs for a genetic link to cancer, the Ph chromosome is characterized by a reciprocal translocation, resulting in the BCR-ABL fusion gene [30]. The BCR-ABL fusion gene is oncogenic and the causative basis for chronic myelogenous leukemia (CML). It is also used for prognosis in pediatric acute lymphoblastic leukemia (ALL), embodying an independent adverse risk factor [31]. Using FISH, the BCR-ABL fusion gene can be detected easily, and therefore, confirm diagnosis, assess prognosis, or monitor disease remission [29, 32].
Advancements in technique also have enabled pathologists to analyze interphase chromosomes as well as metaphase chromosomes [33]. This permits the analysis of many additional tumors with FISH, including rhabdomyosarcoma, the most common soft tissue sarcoma in children. Alveolar rhabdomyosarcoma (aRMS) is a rare subtype of rhabdomyosarcoma that behaves aggressively [34]. The PAX3-FOXO1 fusion gene is a signature genetic marker found in aRMS , which can be detected by FISH [34, 35]. As illustrated in Fig. 16.6, the wild-type FOXO1 gene appears as a single yellow point, formed by overlapping red and green signals. However, separate red and green signals disclose a translocation event in FOXO1, confirming the diagnosis of aRMS.


Fig. 16.6
FOXO1 FISH. Fluorescent in situ hybridization (FISH) study demonstrating widely separated red and green signals (arrows), consistent with translocation within FOXO1 gene. The wild-type FOXO1 gene lacks the translocation (overlapping red and green signals appear as yellow signal, arrowhead) (Courtesy of Paul J. L. Zhang, MD; Department of Pathology and Laboratory Medicine at the Hospital of the University of Pennsylvania, Immunohistochemistry Laboratory; Philadelphia, PA)
Limitations
As with all clinical tests, high sensitivity and specificity are essential to minimize false positive and negative results. Sensitivity of FISH depends on the resolution of the probe signal, while specificity relies on probe construction and accurate hybridization [26]. Resolution is limited by the ability to distinguish a distinct point, which is highly dependent on the conformation of the target. Chromosomes in metaphase are extremely compact, followed by interphase and then naked DNA. Resolution decreases with compaction, which can lead to errors in observations and interpretations [36]. Advancements in microscopy and automated analysis programs have overcome some obstacles to improve resolution significantly, even as far as single-molecule resolution [28].
While probe length may also contribute to sensitivity, where longer sequences are easier to detect than shorter sequences, probe construct greatly impacts specificity [26]. A long probe can partially anneal spuriously, leading to erroneous hybridization. A short strand of DNA or RNA can identify more specific targets, such as a translocation breakpoint [26, 36]. Dual-color break-apart probes, for example, are often employed in such cases. These probes are composed of different colored DNA segments on either side of a breakpoint [37]. If a breakpoint exists, two colors are observed, rather than a single-colored signal indicating no translocation breakpoint. These types of probes have greatly facilitated the visualization of a translocation breakpoint. However, potential diagnostic ambiguity may still arise, especially when the fusion partner is undetectable [37].
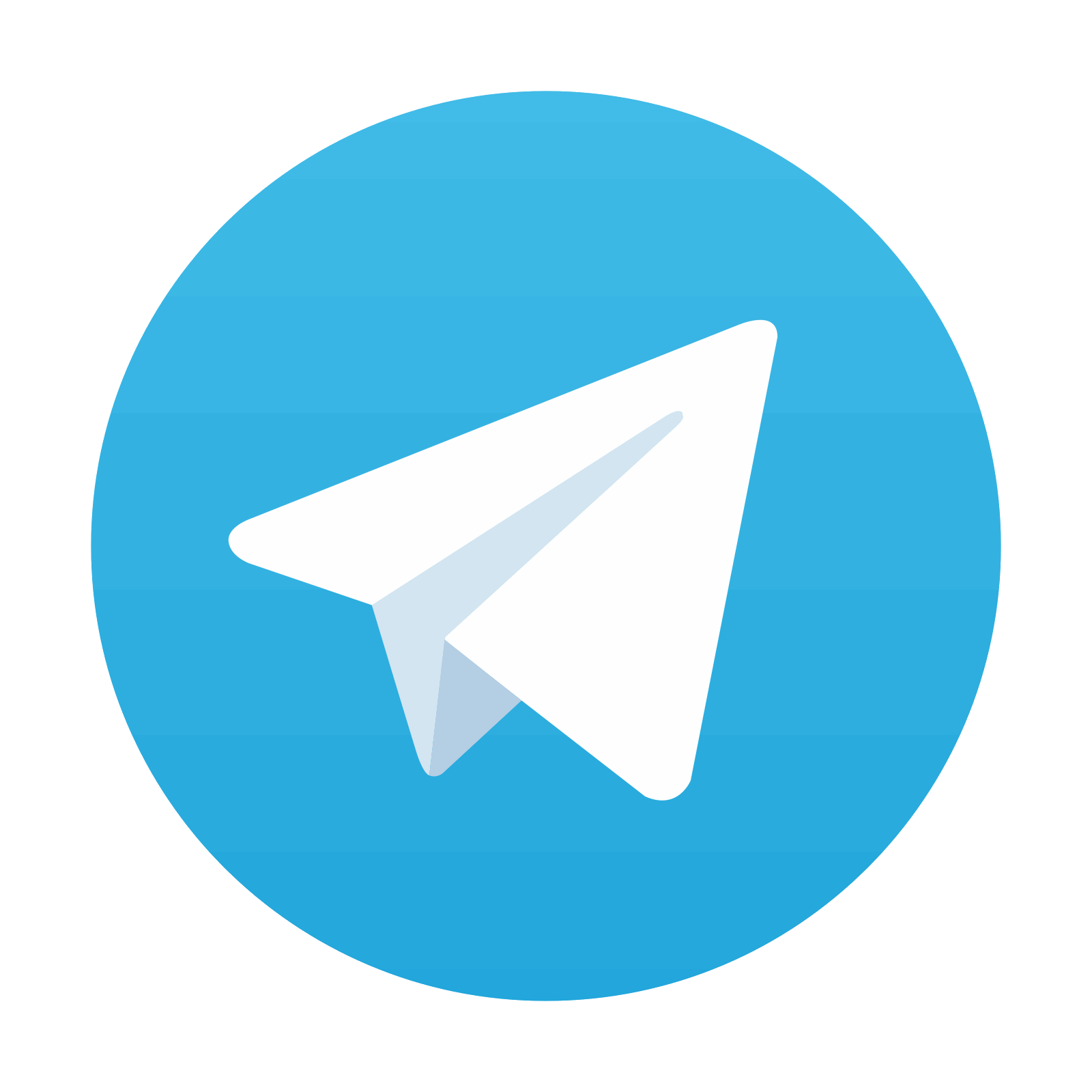
Stay updated, free articles. Join our Telegram channel

Full access? Get Clinical Tree
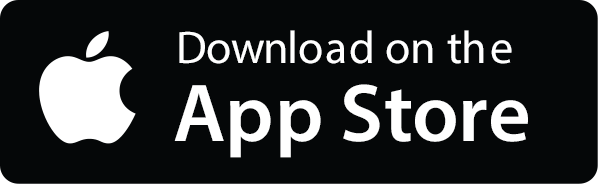
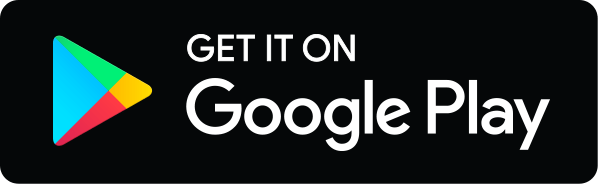