14
Microplasmin and Vitreoretinal Surgery
David T. Goldenberg
Anselm Kampik
Arnd Gandorfer
Michael Trese
INTRODUCTION
The vitreoretinal interface plays an important role in the pathogenesis of many retinal disorders. It is a common belief that traction at this interface contributes to the retinal pathology observed in proliferative diabetic retinopathy, proliferative vitreoretinopathy, macular pucker, diabetic macular edema, vitreomacular traction, macular holes, and retinal detachments. Traction is believed to be mediated by the vitreous cortex and by fibrocellular proliferation, and therefore complete removal of the cortical hyaloid is often a principal goal of vitreoretinal surgery (1). The surgical management of these disorders often targets this interface by separating the posterior hyaloid from the internal limiting membrane (ILM), thereby creating a posterior vitreous detachment (PVD). Mechanical separation of the vitreoretinal interface with vitrectomy may not remove all of the vitreous or completely separate the vitreoretinal junction (2), as cortical vitreous fibrils are left behind on the ILM (3). In addition, incomplete removal of the vitreous may result in surgical failure (4).
Vitrectomy with peeling of the ILM necessitates direct mechanical manipulation of the macula, and although it is generally safe, it can potentially result in trauma to the retina (5, 6). Mechanical separation of the posterior hyaloid from the retina in adult primates demonstrates incomplete vitreoretinal separation and frequently causes damage to the macula and optic disc, including partial or full thickness foveal tears, damage to the optic nerve, separation of the ILM from the retina, and avulsion of the ganglion cells and other retina layers (2). Therefore, the development of a pharmacologic agent that can aid in vitreous liquefaction and PVD creation may facilitate surgical separation of the posterior hyaloid, thus reducing intraoperative time and potential complications. Pharmacologic vitreous liquefaction may help to reduce vitreous viscosity, thereby facilitating removal during vitrectomy and reducing surgical time, especially when using smaller gauge instruments such as 25-gauge and 23-gauge vitrectors.
Intraocular enzymatic agents have been discussed for many years, one of the earliest being alpha-chymotrypsin and its effect on zonular proteins during intracapsular cataract extraction. The search for an appropriate agent to manipulate the vitreous has included several enzymes, most of which are autologous enzymes that activate other endogenous enzymes. Unfortunately, many of the earliest studied agents were fraught with failure due to lack of efficacy, retinal toxicity, or difficult preparation. Microplasmin is a recombinant human enzyme that appears to be a promising agent for pharmacologic manipulation of the vitreous. It has recently been shown to cause vitreous liquefaction and cleavage of the vitreoretinal interface with a single intravitreal injection (7–10). Pharmacologic alteration of the vitreous with microplasmin and other agents is an evolving modality that will likely be used more frequently for therapy and preventative measures (11).
ANATOMICAL CONSIDERATIONS
In the past, the vitreous was considered unimportant in the development of vitreoretinal pathology (7). With recent advancements in biochemistry and vitreous anatomy, we are now able to appreciate the complex arrangement of the vitreous gel and its influence on retinal diseases. The vitreous is a clear, semisolid gel containing hyaluronic acid interspersed in a framework of parallel collagen fibrils coursing in an anteroposterior direction (7). Posterior to the pars plana, the concentration of collagen and hyaluronic acid is greatest in the vitreous cortex, which lies along the inner retinal surface (12). The collagen fibrils, which are condensed to form an outer layer of the vitreous cortex, are adherent to the ILM of the retina (12). Glycoproteins such as laminin and fibronectin are located at the vitreoretinal junction (13) and are believed to contribute to the adhesion of the posterior vitreous cortex to the ILM.
POSTERIOR VITREOUS DETACHMENT
As the human vitreous ages, syneresis and liquefaction of the gel occur with the development of pools of fluid usually in the premacular region or in the central part of the vitreous cavity. Foos and Wheeler (14) found a strong correlation between increasing amounts of vitreous syneresis and the prevalence of PVD. This suggests that the human vitreous gel can tolerate only a certain amount of liquefaction and instability before PVD occurs. Age-related PVD usually occurs as an acute event. A tear in the posterior cortical vitreous allows fluid from the central part of the liquefied vitreous gel to then pass through the break in the vitreous cortex and separate the surrounding cortical vitreous from the retina.
Similar to the development of an endogenous age-related PVD, the success of pharmacologic vitreolysis depends on simultaneous vitreous liquefaction and separation of the vitreoretinal interface. Sebag (7, 15) has used the term “anomalous PVD” to describe the situation in which these two processes are uncoupled, whereby the extent of vitreous liquefaction exceeds the degree of vitreoretinal interface weakening. Anomalous PVD may lead to traction at the vitreoretinal interface and subsequent pathologic conditions including vitreomacular traction syndrome, macular holes, and retinal tears (15).
HISTORICAL CONSIDERATIONS
Prior to the advent of microplasmin, multiple other pharmacologic agents have been developed for enzymatic manipulation of the vitreous. Enzymes such as dispase, chondroitinase, hyaluronidase, tissue plasminogen activator (tPA), and plasmin have had variable success (7, 16). Dispase was initially believed to be a good candidate for pharmacologic vitreolysis due to its ability to hydrolyze several proteins including type IV collagen and fibronectin. In fact, dispase can lead to the creation of a PVD (17, 18); however, it causes anterior chamber and vitreous inflammation, epiretinal membranes, preretinal and intraretinal hemorrhages, cataract, electroretinogram (ERG) amplitude reductions, and ultrastructural damage to the retina (17–19). This intraocular toxicity has limited its clinical utility. Chondroitinase lyses the proteoglycan chondroitin sulfate, which is associated with the vitreoretinal interface (20). A recent masked, placebo-controlled, in vivo study concluded that chondroitinase failed to produce a PVD (21).
Hyaluronidase (Vitrase) is a highly purified ovine enzyme that primarily digests the proteoglycan hyaluronan, which constitutes a large component of the vitreous body. Hyaluronidase has been suggested as an agent to liquefy the central vitreous with the assumption that it may also lead to a PVD after an extended period of time. Hyaluronidase was originally targeted toward patients with dense vitreous hemorrhages in the hope of causing vitreous liquefaction (and settling of blood) to allow for laser photocoagulation in cases of proliferative diabetic retinopathy. A recent prospective, double-masked phase III trial concluded that hyaluronidase is more effective than placebo injections in clearing vitreous hemorrhages (22). Although it has been shown to decrease vitreous macromolecule size suggesting a role for vitreous liquefaction (7), other reports have concluded that intravitreal injection of hyaluronidase cannot induce a PVD in animal models (10, 23, 24). As mentioned earlier, vitreous liquefaction without simultaneous separation of the vitreoretinal interface may induce untoward effects, including vitreomacular traction syndrome, macular holes, and retinal tears (7, 15). Nevertheless, a recent phase III trial concluded that there were no serious safety issues and the incidence of retinal detachment was not statistically different between hyaluronidase and controls (25).
Plasmin enzyme is a nonspecific protease capable of hydrolyzing glycoproteins such as laminin and fibronectin (26, 27), which bridge and bind vitreous collagen fibers between the posterior vitreous cortex and the ILM (13). The liquefaction of vitreous gel by plasmin enzyme is based on its activity on collagenases (16). Unlike dispase, plasmin enzyme spares type IV collagenase, leaving the ILM intact but causing liquefaction in a dose-dependent fashion in the central vitreous cavity (16, 28). tPA has worked through a similar pathway as plasmin enzyme, by activating endogenous plasminogen. The endogenous plasminogen then converts to plasmin enzyme and works on the vitreoretinal junction. Gandorfer et al. (28) reported that a single injection of plasmin enzyme is able to cleave the vitreoretinal junction without causing morphological changes to the retina in postmortem porcine eyes. The authors concluded that the degree of vitreoretinal separation depends on the concentration and length of exposure to plasmin. Plasmin enzyme reaches its peak activity in 15 to 30 minutes and remains at this peak for approximately 90 minutes (29). This activity then falls off over the next several hours to an immeasurable level (29).
Encouraging results with animal studies prompted the desire to pursue human trials. Autologous plasmin enzyme (APE), which can be isolated from the patient’s serum, has been studied extensively in humans. Multiple reports have confirmed that a concentration of 0.4 to 1.2 U of APE can cause vitreous liquefaction and induce a PVD in humans (30–35). Wang et al. (18) evaluated the safety of intravitreal plasmin injections in rabbit eyes. At a concentration that was ten times (4 U) the amount required for PVD formation (0.4 U), they found transient mild intraocular inflammation without ERG changes or morphologic changes to the ILM and retina.
Plasmin-assisted vitrectomy in cadaver eyes demonstrates a smooth retinal surface with only sparse collagen fibrils (3). Human pilot trials with APE-assisted vitrectomy in traumatic macular holes, idiopathic macular holes, and diabetic eyes have been reported (30, 32–35). In these eyes, plasmin enzyme has been shown to allow the vitreous gel to be easier to peel than expected or spontaneously separated in the majority of eyes. The use of plasmin enzyme in diabetics may facilitate membrane peeling by acting on the extracellular matrix fibrin between the cellular pegs of the epiretinal membrane and neurosensory retina, allowing improved exposure and access to the pegs and making them easier to divide (16, 32).
MICROPLASMIN BIOCHEMISTRY
Microplasmin (ThromboGenics Ltd., Dublin, Ireland) is a recombinant protein containing one active protease site of human plasmin but lacking many of the remaining “kringle” domains (36). It functions as a direct-acting thrombolytic agent, and it is a much smaller molecule (29 kD) than human plasmin (88 kD) (36). It has been proposed that the smaller size of microplasmin enables the molecule to penetrate the epiretinal tissue more effectively than plasmin (8). Because it is a recombinant protein, it can be prepared and manufactured in large quantities in limited time. Thus, the venipuncture and labor-intensive preparation that is needed for plasmin can be avoided.
Microplasmin is also in development as a neuroprotective agent with thrombolytic potential for the treatment of ischemic stroke (37). It has been shown to reduce ischemic brain damage and improve neurologic dysfunction in a rat stroke model (38). Microplasmin is currently being investigated in clinical trials for the treatment of acute ischemic strokes and deep vein thromboses.
MICROPLASMIN EFFICACY
In order for pharmacologic vitreolysis to be beneficial, two successful components are necessary: (a) vitreous liquefaction and (b) complete vitreoretinal interface separation (PVD). Sebag (7) has indicated a dose-dependent reduction in the size of porcine vitreous macromolecules (hyaluronan and collagen) after injection with microplasmin. Using dynamic light scattering, the highest dose of microplasmin (0.8 mg) demonstrated an overall reduction in size of about 85% after just 30 minutes. These results suggest that significant vitreous liquefaction is achieved with microplasmin after a relatively brief period of time (7).
Gandorfer et al. (8) injected postmortem human eyes and in vivo feline eyes with microplasmin or saline and confirmed the presence or absence of a PVD with scanning and transmission electron microscopy. The microplasmin-injected eyes demonstrated complete vitreoretinal separation in a dose- and time-dependent fashion in both species (Fig. 14-1). The authors suggested that the effect of microplasmin continues beyond 24 hours. Nevertheless, they concluded that complete vitreoretinal separation can be induced within 30 minutes after injection (Fig. 14-2). Subsequent reports (9, 10) have confirmed the dose dependency of microplasmin and successful PVD induction with microplasmin in animal models using rabbits, guinea pigs, rats, and cats. Thus, microplasmin can successfully induce both vitreous liquefaction and PVD induction in animal studies. These results prompted the idea that microplasmin may be effective in inducing a prophylactic PVD (7). To date, no human pilot trials with microplasmin have been reported; however, clinical trials are underway in Europe and the United States.
Figure 14-1. Scanning electron micrographs of the vitreoretinal interface in human postmortem eyes. A: Intravitreal injection of 62.5 μg of microplasmin resulted in remnants of collagen fibrils covering the internal limiting membrane (ILM). B: 125 μg and C: 188 μg of microplasmin produced complete posterior vitreous detachment (PVD) and a bare ILM. D: Compression of collagen fibrils toward the ILM in an eye treated with 62.5 μg microplasmin and gas. E: Complete PVD following treatment with 125 μg microplasmin and gas. F: Dense network of collagen fibrils in control eye. Magnification 3600x. (From Gandorfer A, Rohleder M, Sethi C, et al. Posterior vitreous detachment induced by microplasmin. Invest Ophthalmol Vis Sci. 2004;45:641–647, reprinted with permission.)
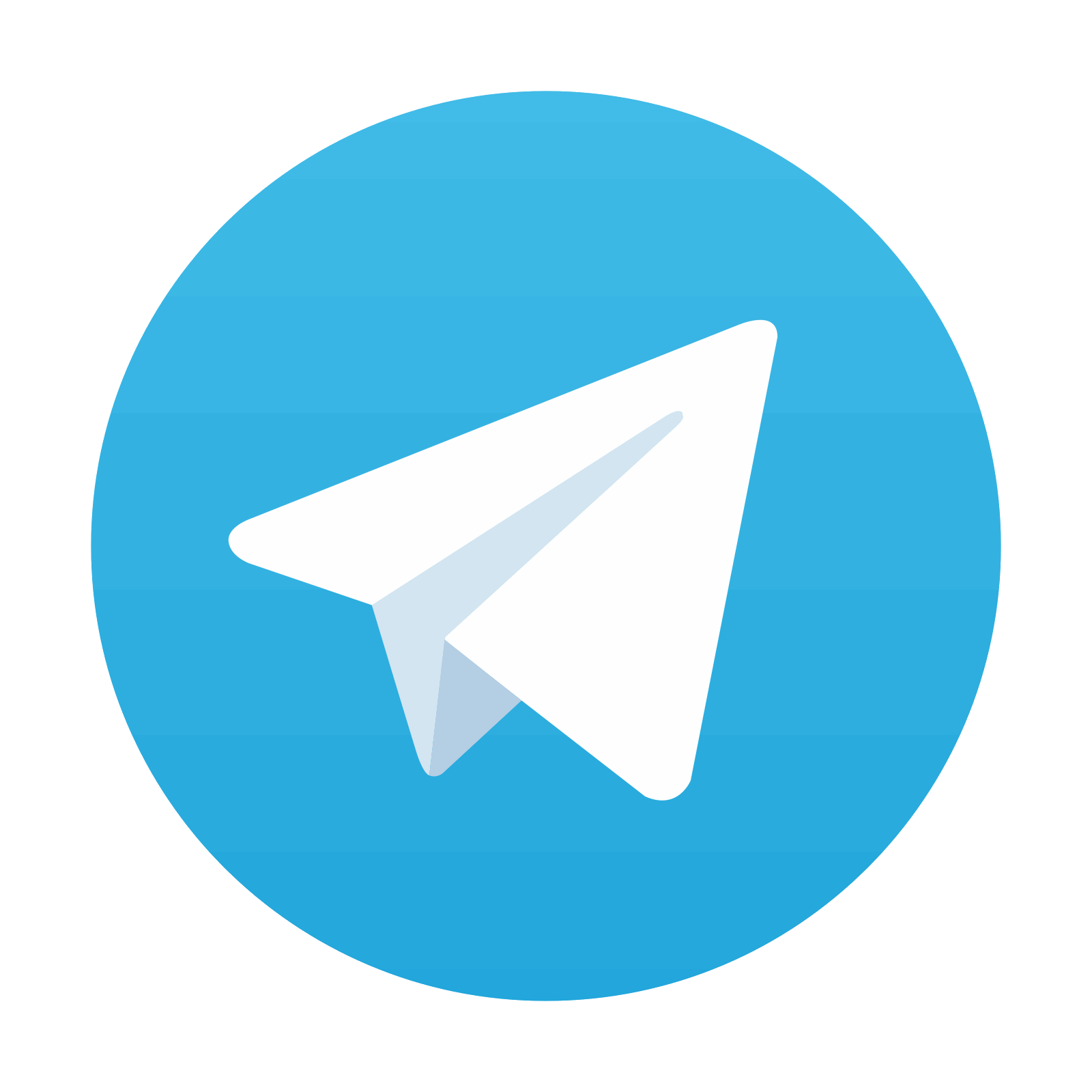
Stay updated, free articles. Join our Telegram channel

Full access? Get Clinical Tree
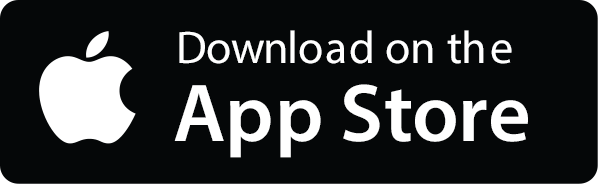
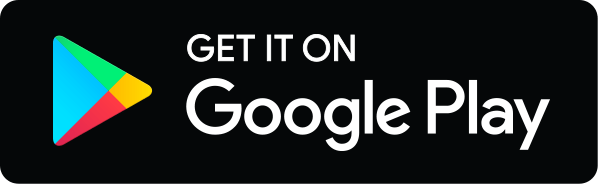