(5)
P.N. Schacknow, MD, PhD Visual Health Center, Palm Springs, FL, USA
During the 1970s, 1980s, and 1990s, the Zeitgeist was such that most medical insurance carriers required that the patient should have exhausted “maximum tolerated medical therapy (MTMT)” before the insurance carrier would consider either laser trabeculoplasty or incisional glaucoma surgery. In many cases, patients were using three, four, or even five different topical medications and perhaps an oral carbonic anhydrase inhibitor. This common use of maximal therapy in all instances resulted in great expense for the patient, poor compliance with complicated and expensive dosing regimens, preservative overload, and symptoms of ocular irritation. MTMT offers little benefit in terms of further reduction of intraocular pressure over what could be accomplished with only one or two different drops. Sherwood and his colleagues at the University of Florida have more recently shown that compliance indeed decreases with the number of drops used, more so as time passes, and with little benefit in the reduction of intraocular pressure for the third or fourth medication [12]. We would like ophthalmologists to evolve from the concept of “maximum tolerated medical therapy” to the idea of “maximum acceptable medical therapy.” An even better concept is “maximum reasonable medical therapy.” The idea is that given all the problems, with using three or more topical medications and oral antiglaucoma medications as well, it is more reasonable to try patients on a single topical glaucoma medication as a first-line agent. If this has only partial success in reaching target pressure, consider adding a second topical agent. If further pressure reduction is required, try substitution of one agent for another or perhaps add a second bottle if it is a combination product of two topical medications. The goal is to keep the number of topical medications at no more than three and the number of bottles at no more than two. If this maximum reasonable medical therapy is unsuccessful, then laser trabeculoplasty, incisional surgery, or one of the new surgical methods would come next in the sequence of treatment.
Medical Therapy
Intraocular Pressure Control Is the Mainstay of Treatment
Medical Therapy to Control Intraocular Pressure
In the USA, the Food and Drug Administration (FDA) has granted approval for glaucoma medications based on their ability to lower intraocular pressure. Considerations of visual field preservation, vascular dysregulation, the toxic effects of preservatives, dosing regimens to optimize compliance, and neuroprotection have not played any role in the FDA drug approval process. Yet all these factors remain major concerns for clinicians in the day-to-day care of glaucoma patients.
The role of fluctuations in intraocular pressure, minute by minute, day to day, and month by month, with respect to the progression of optic nerve head damage and visual field loss, is a matter of interest and controversy [13–17]. Some studies have concluded that fluctuations in intraocular pressure make a significant and independent contribution to glaucoma progression separate from the magnitude of intraocular pressure. Other studies, generally those looking at earlier in the disease course of the patient, suggest that fluctuations in pressure play little role. It seems likely that fluctuation is more important among specific subpopulations of glaucoma patients [15]. Because of the possible role of fluctuations in the progression of glaucoma, it is important to consider the clinical characteristics of medications for treating glaucoma with respect to not only their ability to lower intraocular pressure but providing relatively flat 24-h circadian IOP curves and both diurnal and nocturnal IOP controls (Sidebar 23.2).
Sidebar 23.2. Circadian Variation of Aqueous Humor Dynamics: Implications for Glaucoma Therapy
Arthur J. Sit6
(6)
A.J. Sit, SM, MD Department of Ophthalmology, Mayo Clinic College of Medicine, Rochester, MN, USA
Intraocular pressure (IOP) varies dynamically throughout the circadian cycle. Diurnal variations in IOP were first described by Drance who found that daytime IOP tends to be highest in the morning and decreases over the course of the day. However, 24-h IOP profiles measured in the physiologic positions (upright while awake, supine while asleep) indicate that peak IOP occurs during the nocturnal period for most normal subjects as well as glaucoma patients. The reasons for this pattern are unclear but may have significant implications for the management of glaucoma.
IOP varies with posture, and the elevation of IOP at night can be at least partially explained by the change from the sitting position to the supine position. When IOP measured in the supine-only position is considered, most of the nocturnal elevation of IOP is eliminated for normal subjects, while glaucoma patients show a slight decrease in nocturnal IOP. However, these findings present a paradox since numerous studies on aqueous humor flow indicate that aqueous humor production in the nocturnal period drops by at least 50 % in normal and glaucomatous eyes. If all other factors remained equal, there should be a significant decline in nocturnal IOP, which is not seen in either the supine or physiologic positions.
A recent study examining the circadian variations in aqueous humor dynamics investigated other factors that could produce the observed circadian IOP pattern. Outflow facility measured with Schiotz tonography appeared to decrease slightly at night but not enough to compensate for the decrease in aqueous production. Mathematical modeling of aqueous humor outflow dynamics suggested that the most likely factor responsible for the nocturnal IOP pattern was a change in episcleral venous pressure. However, other factors may have contributed as well, including changes in uveoscleral flow. This research is ongoing, and the mechanisms responsible for the 24-h IOP pattern remain to be elucidated.
Consistent reduction of IOP over the 24-h period is a goal in glaucoma treatment even if the exact reasons for the circadian IOP pattern may be unclear. However, some common glaucoma medications demonstrate a circadian variation in efficacy. The reasons for this can be understood from their mechanisms of action and our current understanding of aqueous humor dynamics.
Beta-adrenergic blockers, such as timolol, appear to lower IOP during the daytime but have reduced or minimal effect during the nighttime. Orzalesi et al. found that twice-a-day timolol had no statistically significant IOP-lowering effect when compared with baseline when IOP was measured in the supine position during the night. Liu et al. confirmed this finding, demonstrating that timolol gel-forming solution lowered IOP once during the day but had essentially no IOP-lowering effect during the night when IOP was measured in physiologic positions (sitting during the day and supine at night). Quaranta et al. did find a statistically significant IOP reduction with twice-a-day timolol when IOP was measured sitting during the day and supine at night but of smaller magnitude than during the day. These results are not surprising when the mechanism of action of timolol is considered. Studies of aqueous humor flow using anterior segment fluorophotometry have repeatedly demonstrated that timolol is a potent aqueous suppressant during the day but does not significantly reduce aqueous flow at night. This is likely related to the normal reduction in aqueous production at night that likely occurs because of a nocturnal decrease in circulating beta-adrenergic agonists. Interestingly, when IOP is measured in the sitting position during the nocturnal period, timolol does appear to maintain IOP-lowering effect, although less than during the diurnal period. This suggests the possibility of different mechanisms of action for timolol in the sitting and supine positions. However, the clinical significance of this finding is unclear since most individuals will assume a supine position for sleep.
The prostaglandin analogues (PGs) have excellent IOP control throughout the 24-h period, although less at night than at the day. Reduction of IOP from PGs is considered to result from an increase of aqueous humor outflow, presumably from the enhanced uveoscleral outflow. The long-term effect is likely related to the remodeling of the extracellular matrix in the ciliary muscle bundles by matrix metalloproteinases, but other mechanisms such as an increase in conventional outflow facility also are involved. The decrease in nocturnal efficacy may be related to the slight decrease in conventional outflow facility that occurs at night. As well, there appears to be a more rapid attenuation of the IOP-lowering effect during the diurnal period than during the nocturnal period after stopping treatment with PGs. This suggests that improvement of conventional outflow facility may be more important during the day, but the effect is of shorter duration than the remodeling of the uveoscleral outflow pathway.
Alpha-2 adrenergic agonists appear to have variable IOP-lowering effect with unclear nocturnal efficacy. Orzalesi et al. found that brimonidine lowered IOP significantly during the day but did not significantly lower IOP at night. In contrast, Quaranta et al. found statistically significant IOP reduction throughout the 24-h period with brimonidine but less than with latanoprost at all time points. The mechanism of action of brimonidine is also unclear. It appears to be an aqueous suppressant but may also have effects on the uveoscleral outflow pathways. The nocturnal effects of brimonidine on aqueous humor flow are currently unknown.
Carbonic anhydrase inhibitors (CAIs) are aqueous suppressants that reduce aqueous humor production. Since they act directly upon carbonic anhydrase in the ciliary body to reduce aqueous humor formation, they are not influenced by the circadian variation of circulation hormones like beta-blockers. As a result, CAIs reduce IOP during the day as well as at night, although less than with PGs.
The 24-h efficacy of cholinergic agonists like pilocarpine is unknown. However, these medications reduce IOP by improving conventional outflow facility. If outflow facility only changes minimally at night, we may speculate that cholinergic agonists will reduce IOP during the nocturnal period, but this remains to be demonstrated.
Conclusion
Optimal therapy for glaucoma would involve IOP control throughout the 24-h period. However, not all therapies are equally efficacious at all times of the day and night. Understanding of the mechanisms of action of different therapies, along with the knowledge of the circadian changes in aqueous humor.
dynamics, allows us to predict the therapies that will provide the most consistent IOP reduction. As well, understanding of aqueous humor dynamics may help in the development of future therapies with consistent 24-h IOP control.
Bibliography
Brubaker RF. Flow of aqueous humor in humans [The Friedenwald lecture]. Invest Ophthalmol Vis Sci. 1991;32(13):3145–3166.
Dailey RA, Brubaker RF, Bourne WM. The effects of timolol maleate and acetazolamide on the rate of aqueous formation in normal human subjects. Am J Ophthalmol. 1982;93(2):232–7.
Drance SM. The significance of the diurnal tension variations in normal and glaucomatous eyes. Arch Ophthalmol. 1960;64: 494–501.
Dubiner HB, Sircy MD, Landry T, et al. Comparison of the diurnal ocular hypotensive efficacy of travoprost and latanoprost over a 44-hour period in patients with elevated intraocular pressure. Clin Ther. 2004;26(1):84–91.
Garcia-Feijoo J, Martinez-de-la-Casa JM, Castillo A, et al. Circadian IOP-lowering efficacy of travoprost 0.004 % ophthalmic solution compared to latanoprost 0.005 %. Curr Med Res Opin. 2006;22(9):1689–97.
Ingram CJ, Brubaker RF. Effect of brinzolamide and dorzolamide on aqueous humor flow in human eyes. Am J Ophthalmol. 1999;128(3):292–96.
Kacere RD, Dolan JW, Brubaker RF. Intravenous epinephrine stimulates aqueous formation in the human eye. Invest Ophthalmol Vis Sci. 1992;33(10):2861–65.
Kaufman PL, Barany EH. Loss of acute pilocarpine effect on outflow facility following surgical disinsertion and retrodisplacement of the ciliary muscle from the scleral spur in the cynomolgus monkey. Invest Ophthalmol. 1976;15(10):793–807.
Konstas AG, Maltezos AC, Gandi S, et al. Comparison of 24-hour intraocular pressure reduction with two dosing regimens of latanoprost and timolol maleate in patients with primary open angle glaucoma. Am J Ophthalmol.1999;128(1):15–20.
Konstas AG, Papapanos P, Tersis I, et al. Twenty-four-hour diurnal curve comparison of commercially available latanoprost 0.005 % versus the timolol and dorzolamide fixed combination. Ophthalmology. 2003;110(7):1357–60.
Konstas AG, Boboridis K, Tzetzi D, et al. Twenty-four-hour control with latanoprost-timolol-fixed combination therapy vs. latanoprost therapy. Arch Ophthalmol. 2005a;123(7):898–902.
Konstas AG, Katsimbris JM, Lallos N, et al. Latanoprost 0.005 % versus bimatoprost 0.03 % in primary open-angle glaucoma patients. Ophthalmology. 2005b;112(2):262–6.
Konstas AG, Lake S, Economou AI, et al. 24-Hour control with a latanoprost-timolol fixed combination vs. timolol alone. Arch Ophthalmol. 2006;124(11):1553–7.
Larsson LI. Aqueous humor flow in normal human eyes treated with brimonidine and timolol, alone and in combination. Arch Ophthalmol. 2001;119(4):492–5.
Lim KS, Nau CB, O’Byrne MM, et al. Mechanism of action of bimatoprost, latanoprost, and travoprost in healthy subjects. A crossover study. Ophthalmology. 2008;115(5):790–5.e4.
Liu JH, Kripke DF, Hoffman RE, et al. Nocturnal elevation of intraocular pressure in young adults. Invest Ophthalmol Vis Sci. 1998;39(13):2707–12.
Liu JH, Kripke DF, Twa MD, et al. Twenty-four-hour pattern of intraocular pressure in the aging population. Invest Ophthalmol Vis Sci. 1999;40(12):2912–17.
Liu JH, Zhang X, Kripke DF, Weinreb RN. Twenty-four-hour intraocular pressure pattern associated with early glaucomatous changes. Invest Ophthalmol Vis Sci. 2003;44(4):1586–90.
Liu JH, Kripke DF, Weinreb RN. Comparison of the nocturnal effects of once-daily timolol and latanoprost on intraocular pressure. Am J Ophthalmol. 2004;138(3):389–95.
Lutjen-Drecoll E, Wiendl H, Kaufman PL. Acute and chronic structural effects of pilocarpine on monkey outflow tissues. Trans Am Ophthalmol Soc. 1998;96:171–191; discussion 92–5.
McCannel CA, Heinrich SR, Brubaker RF. Acetazolamide but not timolol lowers aqueous humor flow in sleeping humans. Graefes Arch Clin Exp Ophthalmol. 1992;230(6):518–20.
Orzalesi N, Rossetti L, Invernizzi T, et al. Effect of timolol, latanoprost, and dorzolamide on circadian IOP in glaucoma or ocular hypertension. Invest Ophthalmol Vis Sci. 2000;41(9): 2566–73.
Orzalesi N, Rossetti I, Bottoli A, et al. Comparison of latanoprost, brimonidine and a fixed combination of timolol and dorzolamide on circadian intraocular pressure in patients with primary open-angle glaucoma and ocular hypertension. Acta Ophthalmol Scand Suppl. 2002;236:55.
Orzalesi N, Rossetti L, Bottoli A, et al. The effect of latanoprost, brimonidine, and a fixed combination of timolol and dorzolamide on circadian intraocular pressure in patients with glaucoma or ocular hypertension. Arch Ophthalmol. 2003;121(4):453–7.
Orzalesi N, Rossetti L, Bottoli A, Fogagnolo P. Comparison of the effects of latanoprost, travoprost, and bimatoprost on circadian intraocular pressure in patients with glaucoma or ocular hypertension. Ophthalmology. 2006;113(2):239–46.
Ota T, Murata H, Sugimoto E, et al. Prostaglandin analogues and mouse intraocular pressure: effects of tafluprost, latanoprost, travoprost, and unoprostone, considering 24-hour variation. Invest Ophthalmol Vis Sci. 2005;46(6):2006–11.
Quaranta L, Gandolfo F, Turano R, et al. Effects of topical hypotensive drugs on circadian IOP, blood pressure, and calculated diastolic ocular perfusion pressure in patients with glaucoma. Invest Ophthalmol Vis Sci. 2006;47(7):2917–23.
Schadlu R, Maus TL, Nau CB, Brubaker RF. Comparison of the efficacy of apraclonidine and brimonidine as aqueous suppressants in humans. Arch Ophthalmol. 1998;116(11):1441–4.
Sit AJ, Weinreb RN, Crowston JG, et al. Sustained effect of travoprost on diurnal and nocturnal intraocular pressure. Am J Ophthalmol. 2006;141(6):1131–3.
Sit AJ, Nau CB, McLaren JW, et al. Circadian variation of aqueous dynamics in young healthy adults. Invest Ophthalmol Vis Sci. 2008;49(4):1473–9.
Topper JE, Brubaker RF. Effects of timolol, epinephrine, and acetazolamide on aqueous flow during sleep. Invest Ophthalmol Vis Sci. 1985;26(10):1315–9.
Toris CB, Gleason ML, Camras CB, Yablonski ME. Effects of brimonidine on aqueous humor dynamics in human eyes. Arch Ophthalmol. 1995;113(12):1514–7.
Toris CB, Zhan G, Fan S, et al. Effects of travoprost on aqueous humor dynamics in patients with elevated intraocular pressure. J Glaucoma. 2007;16(2):189–95.
Toris CB, Zhan GL, Camras CB, McLaughlin MA. Effects of travoprost on aqueous humor dynamics in monkeys. J Glaucoma. 2005;14(1):70–3.
Tsukamoto H, Larsson LI. Aqueous humor flow in normal human eyes treated with brimonidine and dorzolamide, alone and in combination. Arch Ophthalmol. 2004;122(2):1 90–3.
Walters TR, DuBiner HB, Carpenter SP, et al. 24-Hour IOP control with once-daily bimatoprost, timolol gel-forming solution, or latanoprost: a 1-month, randomized, comparative clinical trial. Surv Ophthalmol. 2004;49 Suppl 1:S26–35.
Weinreb RN, Toris CB, Gabelt BT, et al. Effects of prostaglandins on the aqueous humor outflow pathways. Surv Ophthalmol. 2002;47 Suppl 1:S53–64.
The Decision to Treat
Risks and Benefits of Treatment
Setting Target Pressure
The rationale for beginning medical treatment of glaucoma is based on the assumption that lowering intraocular pressure is the most important thing we presently offer the glaucoma patient. Many multicenter, randomized clinical trials have sustained the importance of lowering intraocular pressure [18]. Some of these studies are shown in Table 23.1.
Table 23.1
Multicenter, randomized controlled clinical trials demonstrating importance of reduced intraocular pressure to slow or halt progression of glaucoma
NEI-sponsored clinical trials | ||||
---|---|---|---|---|
Trial | Number of patients/eyes | Diagnosis | Treatments | Follow-up |
EMGT [1] | 255 patients | OAG | Therapy (argon laser trabeculoplasty and betaxolol) vs. observation | 4–9 years |
OHTS [2] | 1,636 patients | OHT | Medical therapy vs. observation | 5 years |
CIGITS [3] | 607 patients | OAG | Medical therapy vs. surgery | 5 years |
AGIS [4] | 738 eyes | OAG | Argon laser trabeculoplasty vs. surgery | 8 years |
CNTGS [5] | 140 eyes | NTG | Medical therapy and/or surgery vs. observation | 7 years |
There are, of course, many other non-IOP factors that influence the choice of medical therapy as initial treatment for any given patient. The decision really rests upon the clinician’s accounting of risk factors for an individual patient. In addition to the ability of medications to lower intraocular pressure, we must also consider the natural course of the disease and the age of the patient, topical and systemic side effects of medications, preservative toxicities, dosing intervals, adherence to medical regimens, and, of course, the possibility of no treatment at all for select patients.
The best predictor of the IOP that an eye will sustain in the future is the pressure that it has had in the past [19]. Before beginning medical therapy, the physician should set a “target IOP” for the patient. The target IOP is the clinician’s best estimate of the maximum intraocular pressure the specific glaucoma patient can tolerate without further damage to their optic nerves and visual fields [20]. This IOP may, of course, differ between the two eyes of the patient. We emphasize that this target pressure is only a clinical estimate and must be updated periodically if progression is noted. It is unfortunate that many ophthalmologists do not reassess the IOP of the patient at each visit with respect to the clinical findings! [21].
The target pressure may serve as a useful reminder for managing the patient, though very often, clinicians articulate the fear that writing such a number in the medical record will work against them in court. First, note that medical malpractice cases in glaucoma care are rare and commonly surround the issue of failure to diagnose [22, 23]. Second, once the ophthalmologist perceives and acts upon the target pressure as an ever-evolving concept based on the current clinical condition of their patient, modified if necessary at each visit, the physician is unlikely to visit target pressure as a legal matter. As of this writing, we are unaware of any instance where target pressure has been invoked in a medical–legal case. Therapy may be adjusted in line with the setting of a new target pressure at each clinical visit as circumstances dictate.
Rather than a single numerical value for an initial target pressure, most glaucoma specialists set a preliminary goal for target IOP as a range of percentage reduction from the initial presenting IOP in the office setting [24, 25]. Expert panel recommendations for initial target pressure range from 25 % reduction for patients with mild glaucoma to more than 35 % initial reduction for patients with severe damage [26]. Factors to be considered in determining initial and subsequent target pressures include the IOP level at which the optic nerve damage occurred (“diagnostic” IOP), the extent of glaucomatous damage (cup-to-disk ratio, disk hemorrhage, visual fields, optic nerve head appearance, and retinal nerve fiber layer imaging), the rate of progression of glaucomatous damage if known, the presence of other known risk factors (age, race, CCT, family history/genetic predisposition), expected life span, and finally the patient’s medical history (systemic hypertension, diabetes, sleep apnea, age, systemic medications).
Some specialists feel that patients are ill-served by setting a target pressure or target-IOP range. One argument sometimes advanced against using a target range is that, theoretically, it seems likely there should be some absolute value of intraocular pressure below which the patient’s glaucoma will not progress, and by using a range, we are simply admitting that we do not know how low to go for that individual patient. But setting a target-IOP range is useful when it is based on the clinician’s experience and the many factors described previously, as long as it is reevaluated at each office visit based on clinical findings.
Intraocular pressure determinations are made in the ophthalmologist’s office during clinic hours. Recent studies have suggested that a large proportion of patients’ highest daily IOPs occur outside the usual office hours [27, 28] and may also be the highest during the portion of the night when the patient is supine and sleeping. Target IOPs have traditionally been determined based on one or two measurements of the patient’s IOP during usual daylight office hours and thus may not be representative of the true IOPs when glaucomatous damage may occur. Patients who suffer from sleep apnea [29–34] or may have body builds with “thick” necks may pose additional problems when trying to determine their true IOPs and setting target pressures. Most patients have their highest intraocular pressure upon awakening. This may mean that it is ideal to dose medication upon awakening rather than waiting until later in the morning. Occasional clinic visits early in the morning before the office normally would open and may reveal elevated intraocular pressures that would not otherwise be detected.
Patients should be involved in discussions about target IOPs. Patients need to be apprised that simply determining how close their IOP is to “target” at each office visit is not sufficient to determine the stability or progression of their glaucoma. Changes in the optic nerve head, via ophthalmoscopy, photography, or imaging devices, and functional visual field and acuity changes are the true measures of glaucoma status. IOP is an easily measured but poor surrogate for “defining” glaucoma.
Anatomy and Physiology of Aqueous Humor Production and Outflow
In the first section of this chapter, we have had a discussion of the rationale behind the medical therapy of glaucoma. Before beginning a discussion of the medications themselves, we will now describe the anatomical and physiologic substrates related to the ingress and outflow of aqueous humor within the eye.
Anatomy of Aqueous Humor Production
The lens and cornea are avascular structures that are supplied nonetheless with nutrients and oxygen by the aqueous humor, which also serves to remove metabolic waste products as it is drained from the eye. Aqueous humor is produced within the 70–80 ciliary processes that are radial folds in the pars plicata of the ciliary body. Both active and passive mechanisms are involved in aqueous production. The two-layered epithelium covering the ciliary processes is sealed together by tight junctions (zonula occludens) forming the blood–aqueous barrier between the posterior chamber and blood vessels that pass through the ciliary body and its processes [35, 36].
Passive mechanisms of aqueous production include diffusion and ultrafiltration:
Diffusion – lipid-soluble substances pass through the cell membrane in response to a concentration gradient across the membrane.
Ultrafiltration – water and water-soluble molecules flow through micropores in the cell membrane based on an osmotic gradient, IOP, blood pressure in the ciliary vasculature, and the plasma oncotic pressure (from colloidal proteins).
Active mechanisms of aqueous production involve secretion:
Secretion – large water-soluble molecules are actively moved across cell membranes. This requires and involves enzymes (e.g., Na-K ATPase and glycolytic) found in nonpigmented epithelial cells. The majority of aqueous production is mediated by active transport mechanisms.
Aqueous production starts in the blood with the final product eventually reaching the anterior chamber. The route is as follows (Fig. 23.1):
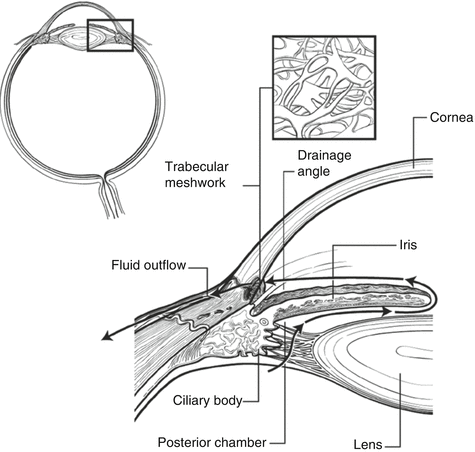
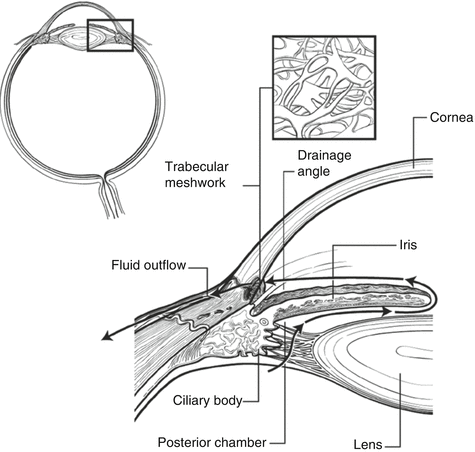
Fig. 23.1
Route of aqueous humor production and outflow
Blood plasma ultrafiltrate > capillary endothelial cells > ciliary process interstitial tissue > pigmented epithelium > nonpigmented epithelium (active transport) > posterior chamber > pupil > anterior chamber.
Aqueous humor is a “complex mixture of electrolytes, organic solutes, growth factors, and other proteins that supply nutrients to the nonvascularized tissues of the anterior chamber (i.e., trabecular meshwork (TM), lens, and corneal endothelium).” [37] Aqueous is produced at a rate of 2.0–3.0 μl/min. or 1.5 % of anterior chamber volume/min. The anterior chamber holds approximately 250 μl of aqueous humor, and the posterior chamber about 60 μl of aqueous humor.
All the aqueous is replenished about every 1.5–2 h during the daytime hours. Relative to blood plasma, aqueous has approximately 15 times greater ascorbate and much less protein (0.02 % in aqueous versus 7 % in plasma). Aqueous humor formation decreases with sleep [38] (suppression of 45 ± 20 %) and advancing age [39] (decrease of 2.5 % per decade). Men and women on average have the same rate of aqueous flow [38].
After entering the anterior chamber and angle structures, there are two pathways for the outflow of aqueous humor from the eye [40]. The “conventional” or trabecular-meshwork outflow path is said to be “pressure dependent.” This pathway is usually thought to account for about 80 % of aqueous outflow. The route of aqueous outflow through the trabecular meshwork is as follows:
Anterior chamber > trabecular meshwork > Schlemm’s canal > scleral collector channels > episcleral aqueous veins > anterior ciliary veins > superior ophthalmic veins > venous system
The “unconventional” or uveoscleral outflow path is said to be “pressure independent.” This pathway accounts for the remaining 20 % of aqueous outflow, although there is conjecture that the actual number is higher. The root of aqueous outflow through the uveoscleral pathway is as follows:
Anterior chamber > iris root > ciliary body interstitial tissue > suprachoroidal space (negative pressure) > sclera? vortex veins? along the nerves? > posterior ciliary veins > venous system
The majority of glaucoma medications are eye drops that are placed on the surface of the eye. They enter through the conjunctiva and cornea, with the fornix serving as a reservoir. Usually, drops are applied in great excess, and most of the medication does not enter the eye. The root of absorption into the eye from an eye drop is from the conjunctival cul-de-sac, then becoming part of the tear film (mucin, water, and oil) that is 7–9 μm thick, then passively diffusing into cornea and conjunctiva, and then becoming part of the aqueous humor. Peak concentration of drug in the aqueous humor takes from 30 to 60 min. Glaucoma drugs are generally not uniformly distributed throughout the eye, because of both physical barriers like iris, lens, and ciliary body, as well as circulatory factors like bulk flow of aqueous humor and the blood circulation. In addition, medications often differentially bind to tissues, especially those containing melanin [40].
The great majority of the drop applied to the eye (some estimate this to be 80 % or greater) exits via the lacrimal drainage apparatus and does not enter into the eye at all (see Sidebar 23.3). The cul-de-sac holds at most 30 μL of an eye drop. Most of the drop drains from the cul-de-sac of the eye within the first 5 min. Blinking eliminates about 2 μL of fluid from the cul-de-sac [41]. The route of nasolacrimal duct drainage is conjunctival cul-de-sac > inferior and superior nasal puncta > canaliculi > lacrimal sac > nasolacrimal duct > valve of Hasner > inferior nasal turbinate > then absorption into the vascularized nasopharyngeal mucosa or swallowed [40, 118].
Sidebar 23.3. How to Use Eye Drops to Treat Glaucoma
Odette V. Callender7
(7)
Odette V. Callender, MD Department of Ophthalmology, Wilmington VA Medical Center, Wilmington, DE, USA
It is easy to tell patients to “take these two pills twice a day with meals.” Patients understand these instructions and usually are able to adapt this regimen to their lifestyle. Swallowing is something we all do naturally, and, even if currently healthy, most of us have swallowed pills at some point in our lives – vitamins, aspirin, acetaminophen, ibuprofen, etc. For those who have difficulty swallowing, pills can often be crushed and dissolved in a liquid or mixed with food. We can usually discretely swallow a pill even when in a public venue.
How about the doctor instead instructing the patient to “take these two drops twice a day”? This may be a tall order requiring much more than just adapting the schedule to the patient’s lifestyle. Instilling drops in our eyes is not something we do naturally. In fact quite the opposite is true given the instinctive protective reflex of closing your eyes when something approaches them. Not only do our patients have to overcome this natural reflex, but many of them are elderly and have difficulty handling the small bottles of eye drops because of arthritis or perhaps cognitive issues. Many people have never had to use eye drops before the prescription you just gave to them. It’s impossible to discretely instill an eye drop at the dinner table. Using eye drops requires a new skill set and social adjustments different from taking other kinds of medications prescribed by non-eye care physicians. Yet when writing a prescription or providing a sample, do you (or your staff) routinely ask patients if they have ever instilled drops in their eyes before? Does your practice routinely provide written or hands-on instruction on how to instill eye drops? Do you watch patients demonstrate instillation of drops with perhaps a sample bottle of artificial tears? With our incredibly busy office/clinic schedules, the answer to these questions is often NO.
A study by Winfield et al. looking at the causes of patient noncompliance revealed a wide variety of problems patients have instilling their drops. The most important finding was that 69 % of those patients in the study stated that they would not have reported compliance barriers to their physician even if specifically questioned. We cannot rely on patients to tell us spontaneously that they are having difficulty using their drops, but we as their physicians must instead take a proactive approach. One-on-one training between patients and physicians or staff, while ideal, is often not feasible. In office video, education is likely only available in a limited number of practices. Written material gives the patient something which they may refer and share with family and friends who may assist with drop instillation. Step-by-step instructions that include answers to “frequently asked questions” will benefit patients while saving you and your staff time. Any patients for whom we prescribe drops, not just our glaucoma patients, can benefit from such instructions.
It is instructive to occasionally ask your long-term patients to demonstrate drop instillation in the office. You may be in for a rude awakening when you find that more of the medication reaches their cheeks than their fornices! Such a situation provides an opportunity for reinstruction in instillation techniques. Do not let your patient leave the office until you feel comfortable that they or their accompanying care provider can accurately administer glaucoma drop medications. Provide instructions for both new and “experienced” patients and consider offering compliance and/or instillation aids. Although 78 % of patients in the study by Winfield et al. responded favorably to the suggestion of using compliance aids, none of them were aware that aids were available.
Many doctors instruct patients to perform punctual occlusion. However, despite instruction, many patients do not perform proper punctual occlusion negating the benefits. “Nasolacrimal occlusion, a technique in which digital pressure on the periphery of the nasolacrimal drainage system, obstructs drainage to the nasopharyngeal mucosa, and has been shown to significantly decrease systemic absorption. Eyelid closure for 5 min following drug application also achieves the same purpose by inhibiting nasolacrimal pump action.” This drop instruction sheet describes eyelid closure that may be easily done by all patients without requiring digital dexterity. It is a reasonable alternative to punctual occlusion. If you prefer that patients perform punctual occlusion, they should also do so while closing their eyes. We personally demonstrate punctual occlusion on ourselves and then ask the patient to do so in front of us. We reinstruct them and guide their fingers as necessary to adequately perform this technique.
Bibliography
Gerber SL, Cantor LB, Craig Brater D. Systemic drug interactions with topical glaucoma medications. Surv Ophthalmol. 1990;35(3):205–18.
Winfield AJ, Jessiman D, Williams A, Esakowitz L. A study of the causes of non-compliance by patients prescribed eyedrops. Br J Ophthalmol. 1990;74:477–80.
Corneal Penetration by Drugs
The multilayered cornea is not easily penetrated by drugs. The corneal structure may be described as a lipid–water–lipid sandwich [42] (Fig. 23.2). The zonula occludens (tight junctions) limit the passage of aqueous-based medications through the epithelium and into the stroma. Some preservatives, such as benzalkonium chloride (BAK), act as detergents and break up the tight junctions, greatly enhancing the entrance of water-soluble substances.
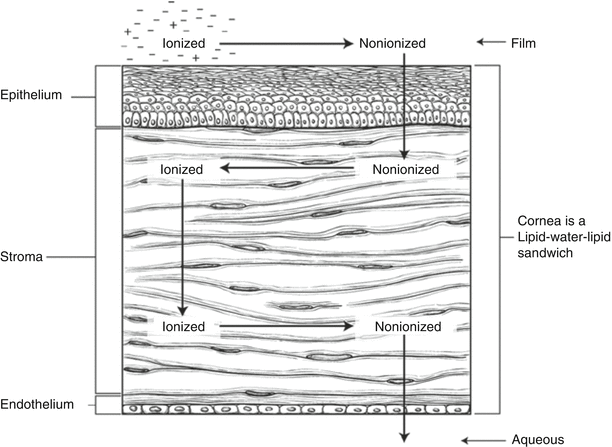
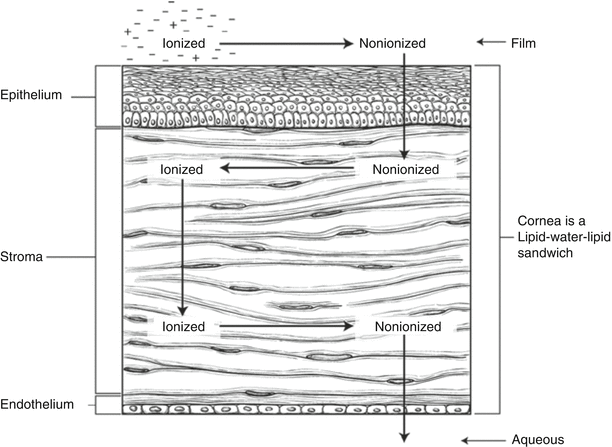
Fig. 23.2
Anatomy of drug penetration into the eye
Unfortunately, BAK contributes greatly to symptoms of ocular surface disease experienced by many glaucoma patients, and its presence has been ubiquitous in glaucoma medications [8, 10, 43–45]. Newer drugs, such as brimonidine [46, 47] with Purite and travoprost [48] with the preservative SofZia and timolol in the single-use Ocudose form, do not contain BAK (see Sidebar 23.4 for more discussion on the role of preservatives in glaucoma medications and ocular surface disease). Lipid-based molecules do easily penetrate the corneal epithelium but have somewhat retarded passage through the stroma of the cornea. Hydrophilic drug molecules can be esterified to become lipophilic and function as “prodrugs” that easily pass through the corneal epithelium. The cornea is rich in esterases that in turn hydrolyze the ester bond as the drug passes through it. Glaucoma medications such as Propine (esterified epinephrine) and latanoprost and travoprost (esterified derivatives of prostaglandin F2α (F2 alpha)) make use of the prodrug principle. As prodrugs, these compounds are about ten times [49] more soluble than their original molecules.
Sidebar 23.4. Preservatives and Glaucoma Medications
Clark L. Springs8
(8)
Clark L. Springs, MD Department of Ophthalmology, Indiana University, Indianapolis, IN, USA
Ocular surface disease (OSD) in the glaucoma patient is a frequent cause of discomfort and visual degradation. It is often under-recognized, undertreated, and a frequent source of frustration for patient and physician, alike. OSD encompasses aqueous tear deficiency, lipid abnormalities (i.e., meibomian gland dysfunction, blepharitis), and mucin deficiencies due to decreased goblet cell function. These deficiencies can lead to increased tear osmolarity, an upregulation in inflammatory mediators, and, ultimately, ocular irritation. A variety of risk factors can contribute to this dysfunctional tear state such as age, systemic diseases, and medications, as well as preservatives in topical ophthalmic medications. Climate and relative humidity are also a major factor.
Benzalkonium chloride (BAK), a quaternary ammonium compound with surfactant properties, is the most commonly used preservative in topical ophthalmic medications. It is a highly effective antimicrobial agent that prevents contamination. BAK can also enhance corneal penetration of some drugs by separating the epithelium. The mechanism of action of BAK is by irreversible binding of cell membranes altering cell permeability.
The effects of BAK are nonselective, which can adversely affect the health of various ocular tissues. On the ocular surface, BAK can decrease tear breakup time and compromise epithelial cell integrity, which can impair healing and exacerbate OSD. In the conjunctiva, BAK can induce chronic subclinical inflammation and fibroblastic transformation, which can negatively impact goblet cell density and accessory lacrimal gland function. The effects of BAK are dose dependent and cumulative. In cell culture systems, at a concentration of 0.0001 %, cell arrest occurs; at 0.01 %, apoptosis occurs; and at 0.05–0.1 %, cell necrosis occurs. The concentration of BAK in commercially available glaucoma medications ranges from 0.05 to 0.2 %.
In glaucoma, the use of chronic BAK may be a significant concern. Many patients are elderly and use multiple ophthalmic medications on a chronic basis for the treatment of their glaucoma and other ophthalmic conditions (e.g., OSD). These factors can be responsible for poor tolerance of glaucoma eye drops, due to inflammation and toxicity. Further, patients undergoing glaucoma filtering have a higher rate of failure if they have been on topical medications preserved with BAK. BAK has also been implicated in the exacerbation of postoperative cystoid macular edema because of its ability to break down the blood–aqueous barrier. Between 8 and 15 % of the US population have symptoms of ocular surface disease. However, in the glaucoma population, the prevalence of OSD symptoms is significantly higher, 40–58 %. Further, the severity of ocular surface symptoms increased with the number of medications used.
Currently, ophthalmologists do not have a single practical, reproducible test that can help them diagnose and stage OSD. Physicians must therefore rely on their clinical judgment and formulate treatment plans based on a combination of objective testing and their assessment of individual patients’ symptoms. Questionnaires such as the Ocular Surface Disease Index are well-validated tools for detecting and staging ocular surface disease especially when they are used in conjunction with clinical testing. In 2007, the International Dry Eye Workshop published its guidelines for diagnosing and treating ocular surface disease. In addition to identifying critical clinical tests, this report recommended that physicians adhere to the following sequence when objectively assessing patients for OSD:
1.
Measure tear breakup time with fluorescein staining.
2.
Perform vital staining with lissamine green or rose bengal.
3.
Evaluate the production of tears with Schirmer testing.
4.
Assess the morphology of the eyelids and meibomian glands.
5.
Test the function of the meibomian glands.
Performing these tests out of order may affect their integrity and provide inaccurate information.
The elimination of BAK from OTC lubricants is recognized as the single most critical advance in the treatment of OSD. Similarly, limitation of BAK load in glaucoma drops is a desirable goal and one that is being actively pursued by industry. Preservative-free preparations can be compounded extemporaneously, and a topical preservative-free beta-blocker is commercially available (Timoptic Ocudose; Merck, Whitehouse Station, New Jersey). The cost and inconvenience of preservative-free preparations are often prohibitive. Gentler oxidizing preservatives that dissipate on the ocular surface have recently been introduced in topical glaucoma medications. Brimonidine preserved with sodium chlorite (Alphagan-P; Allergan, Irvine, California) is the only alpha-agonist available in a BAK-free formulation. Travoprost preserved with a buffered solution of borate, zinc, and polyols (Travatan Z; Alcon Laboratories, Ft. Worth, Texas) is currently the only commercially available prostaglandin analogue without BAK. In an in vitro model of conjunctival epithelial cells, travoprost without BAK-maintained cell viability is comparable to physiologic buffered saline, while BAK-preserved preparations demonstrated significant loss of cell viability and apoptotic activity. An in vivo rabbit model has shown a beneficial effect of the oxidizing preservatives sodium chlorite and SofZia with preservation of microvilli and less conjunctival inflammation, in comparison to BAK-containing glaucoma medications. Horsley and Kahook found that replacing latanoprost with 0.02 % BAK with travoprost SofZia for 8 weeks increased the mean tear breakup time of 20 consecutive patients (40 eyes) from 2.02 ± 0.71 to 6.34 ± 1.31 (p < 0.001). The patients also reported an improvement in their dry eye symptoms on the Ocular Surface Disease Index at the end of the study (26.31 ± 8.25–16.56 ± 6.19 (p < 0.001)). Further studies are under way to determine the significance of this preliminary report.
In summary, OSD is a serious and debilitating condition that is prevalent among the elderly. Further, OSD appears to be exacerbated by the use of topical ophthalmic medications preserved with BAK. Together, these factors increase the risk that elderly glaucoma patients will develop OSD.
Physicians can prevent or minimize OSD among their glaucoma patients by carefully evaluating clinical signs and symptoms. Minimizing or eliminating exposure to extrinsic factors such as the preservative BAK may reduce the incidence and severity of OSD among those who use glaucoma medications.
Bibliography
Baudouin C, de Lunardo C. Short term comparative study of topical 2 % carteolol with and without benzalkonium chloride in healthy volunteers. Br J Ophthalmol. 1998;82:39–42.
Baudouin C, Pisella PJ, Fillacier K, et al. Ocular surface inflammatory changes induced by topical antiglaucoma drugs. Ophthalmology. 1999;106(3):556–63.
Baudouin C, Riancho L, Warnet J, et al. In vitro studies of antiglaucomatous prostaglandin analogues: travoprost with and without benzalkonium chloride and preserved latanoprost. Invest Ophthalmol Vis Sci. 2007;48:4123–28.
Bourcier T, DeSaint Jean M, Brignole F, Goguel A, Baudouin C. Expression of CD40 and CD40 ligand in the human conjunctival epithelium. Curr Eye Res. 2000;20:85–94.
Broadway DC, Grierson I, O’Brien C, et al. Adverse effects of topical antiglaucoma medication. Arch Ophthalmol. 1994;112:1437–45.
De Saint Jean M, Debbasch C, Brignole F, Warnet JM, Baudouin C. Relationship between in vitro toxicity of benzalkonium chloride (BAC) and preservative-induced dry eye. Adv Exp Med Biol. 2002;506(Pt A):697–702.
Fechtner R, Budenz, D, Godfrey D. Prevalence of ocular surface disease symptoms in glaucoma patients on IOP-lowering medications. Poster presented at the 18th annual meeting of the American Glaucoma Society; March 8, 2006; Washington, DC.
Horsley MB, Kahook MY. Changes in tear break-up time and ocular surface disease index after initiation of travoprost with Sofzia in patients previously using latanoprost with benzalkonium chloride. Poster presented at the 18th annual meeting of the American Glaucoma Society; March 8, 2006; Washington, DC.
Kahook MY, Noecker RJ. Comparison of corneal and conjunctival changes after dosing of travoprost preserved with Sofzia, latanoprost with 0.02 % benzalkonium chloride, and preservative-free artificial tears. Cornea. 2008;27(3):339–43.
Leung EW, Medeiros FA, Weinreb RN. Prevalence of ocular surface disease in glaucoma patients. J Glaucoma. 2008;17(5):350–5.
Management and therapy of dry eye disease: report of the management and therapy subcommittee of the international dry eye workshop (2007). Ocul Surf. 2007;5(2):163–78.
Miljanovic B. Dana R. Sullivan DA. Schaumberg DA. Impact of dry eye syndrome on vision-related quality of life. [Journal Article. Randomized Controlled Trial. Research Support, N.I.H., Extramural. Research Support] Am J Ophthalmol. 2007;143(3):409–15.
Miyake K, Ibaraki N, Goto Y, et al. ESCRS Binkhorst lecture 2002: pseudophakic preservative maculopathy. J Cataract Refract Surg. 2003;29:1800–10.
Montes-Mico R. Role of the tear film in the optical quality of the human eye. J Cataract Refract Surg. 2007;33(9):1631–35.
Noecker R. Effects of common ophthalmic preservatives on ocular health. Adv Ther. 2001;18:205–15.
Noecker RJ, Herrygers LA, Anwaruddin R. Corneal and conjunctival changes caused by commonly used glaucoma medications. Cornea. 2004;23(5):490–6.
Ocular Surface 2007 Report of the International Dry Eye Workshop. Ocul Surf. 2007;5(2):65–206.
Sasaki H, Nagano T, Yamamura K, Nishida K, Nakamura J. Ophthalmic preservatives as absorption promoters for ocular drug delivery. J Pharm Pharmacol. 1995;47(9):703–7.
Schiffman RM, Christianson MD, Jacobsen G, Hirsch JD, Reis BL. Reliability and validity of the ocular surface disease index. Arch Ophthalmol. 2000a;118:615–21
Schiffman RM, Christianson MD, Jacobsen G, Hirsch JD, Reis BL. Reliability and validity of the ocular surface disease index [see comment]. [Journal Article Research Support, Non-U.S. Gov’t] Arch Ophthalmol. 2000b;118(5):615–21.
Prostaglandins Versus Prostamides
Allergan contends that bimatoprost, with an amide moiety rather than an ester group, does not function as a prodrug but enters the eye through the sclera as an intact prostamide [50]. Several studies have shown, however, that amidases within the corneal epithelium cleave the amide group from the basic bimatoprost molecule. This hydrolyzed bimatoprost molecule has been shown to activate the same prostaglandin FP receptors as the hydrolyzed molecules of latanoprost and travoprost [51, 52]. It is our opinion that most glaucoma specialists consider bimatoprost, latanoprost, and travoprost and the recently introduced tafluprost to likely work through the same set of receptors and have the same mechanism(s) of action [51]. Unoprostone, a docosanoid, had been taken out of the US market for several years but was recently reintroduced by Sucampo. Most glaucoma specialists consider it chemically related to the other hypotensive lipids, likely having a similar mechanism of action. It does not lower IOP to the same degree as the other four approved medications in this class and is the only one that requires twice-per-day dosing.
Relatively high concentrations of active medications found in glaucoma drops can result in significant systemic side effects when these drops get into the body after exiting the eye through the nasolacrimal duct system. Occlusion of the nasolacrimal puncta near the medial canthus of both upper and lower eyelids of each eye after topical application of glaucoma drops may reduce systemic absorption up to 70 %. The technique of nasolacrimal punctal obstruction (NLO) can be easily taught to patients (Fig. 23.3), but it is important to watch them personally demonstrate the technique in the office [53, 54]. In many cases, patients incorrectly use digital pressure on the nasal bones or through the eyelid in the middle of the globe rather than over the anatomical area where the puncta are found. Patients should be asked to perform NLO for between 2 and 5 min, although this is often impractical 100 % of the time. Hand washing and keeping the nails trimmed are often neglected but useful adjuncts for nasal lacrimal duct obstruction.
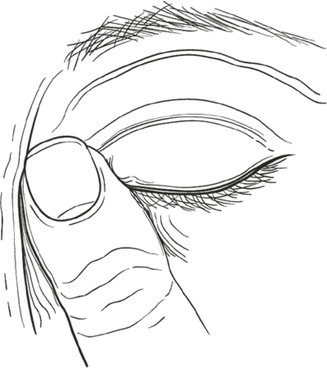
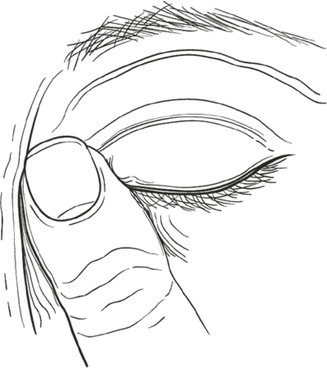
Fig. 23.3
Nasolacrimal occlusion digital massage technique. Put the index finger on corner of eye near nose, closing the tear duct drainage system. Keep lid closed with gentle pressure for 1–2 min
Pharmacological Basis of Drug Activity: The Autonomic Nervous System
Medications work by acting on receptors found on nerve cells and target end organs. Drugs used to treat glaucoma, both eye drops and oral agents, act as either agonists or antagonists after binding to receptors. What follows is a brief discussion of the organization of the nervous system and the major receptor classes that are of interest to us regarding the biological activity of glaucoma drugs.
These sympathetic nerve ganglia start within the vertebral column found between the first thoracic segment and the third lumbar segments of the spinal cord. These ganglia not only contain the sympathetic trunks but also include the cervical ganglia that send sympathetic nerve fibers to the head and thorax organs and to the celiac and mesenteric ganglia that in turn direct sympathetic fibers to the abdominal organs. The neurotransmitter secreted by the preganglionic cells is acetylcholine where they synapse with the postganglionic cell. The postganglionic cell soma is found in the ganglion – its nerve fiber distributed to target organs or glands.
Norepinephrine is found at the synapses of postganglionic cells where they join with the target site. Depolarization of the cell membrane causes the release of norepinephrine stored within granules. Epinephrine is the neurotransmitter secreted by the adrenal medulla directly into the blood where it circulates to reach target organs.
The adrenergic receptors are the major receptor subtypes found within the sympathetic nervous system. A landmark study by Ahlquist [55] in 1948 described two classes of adrenergic receptors – alpha and beta – based on the potency of response to neurotransmitters. When first proposed, this idea was considered radical, because at that time, most scientists thought it was the neurotransmitter itself that determined the response of the end organ, not the receptor that was activated.
There are two alpha-receptor subtypes. The neurotransmitters epinephrine and norepinephrine stimulate both subtypes equally well. The alpha-1 receptor is the more common, and it is found mostly in smooth muscles and some glands. The alpha-2 receptor is found at presynaptic terminals of the adrenergic nerves. It functions as an autoreceptor, which when activated decreases the subsequent release of neurotransmitter in a negative feedback loop.
Three subclasses of beta-receptors have been identified. Beta-1 receptors are located in heart muscle and kidney. When stimulated, they increase heart rate and contractility and cause the release of renin from the kidney. Both epinephrine and norepinephrine are effective in stimulating beta-1 receptors. Beta-2 receptors reside in smooth muscle that relaxes upon activation. Beta-2 agonists cause bronchodilation in the lungs and vasodilation in skeletal and cardiac muscle. Epinephrine is much more effective in stimulating these receptors than norepinephrine. The final beta-3 receptor class is found in fat cells. Activation promotes lipolysis, causing the release of fatty acids. Both epinephrine and norepinephrine work about equally well in activating these receptors.
Norepinephrine can be an agonist that affects both pre- and postsynaptic receptors. It can be removed by uptake into the presynaptic neuron or by uptake into the postsynaptic neuron (see Fig. 23.4). Some norepinephrine simply leaks out of the synapse into the general circulation [56]. Both epinephrine and norepinephrine are deactivated by catechol-O-methyltransferase (COMT), which is found on the postsynaptic cell membrane. They are also inactivated by monoamine oxidase-a (MOA-a) [57], which occurs after “uptake 1” as depicted in Fig. 23.4.
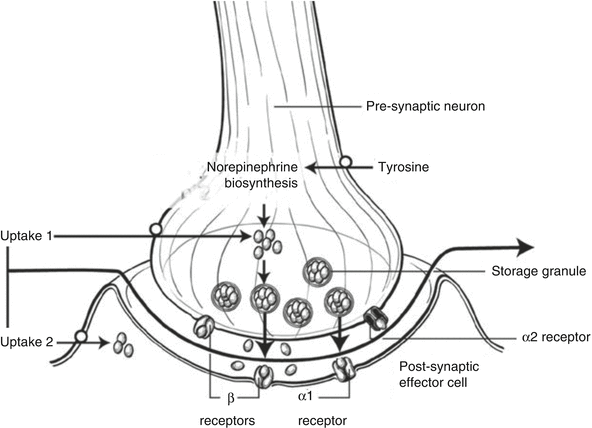
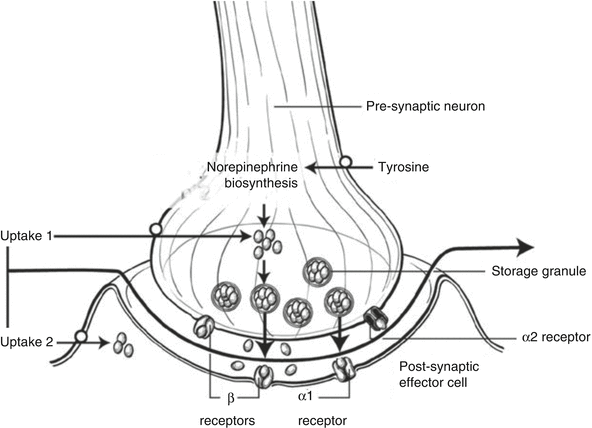
Fig. 23.4
Stimulation and the activation of neurotransmitter
Some adrenergic agonists are said to be direct acting, that is, they bind directly to the receptor and cause a response through their intrinsic activity. Other adrenergic agonists act indirectly by binding to the receptor and causing the release of either epinephrine or norepinephrine. Some indirect agonists work by inhibiting the reuptake of norepinephrine into the synapse. Finally, some drugs work as “mixed-action agonists,” that is, they have both direct agonist characteristics but also cause the release of epinephrine and norepinephrine.
Both adrenergic agonists and adrenergic antagonists (blocking agents) may demonstrate nonselectivity by activating several of the beta-receptor subtypes, or they may be relatively selective and have greater activity at a specific subtype. For example, beta-blockers such as timolol eye drops are nonselective, activating both beta-1 and beta-2 subtypes, while betaxolol eye drops have much greater activity at the beta-1, rather than the beta-2, subtype of receptor. Alpha-agonists such as apraclonidine eye drops are nonselective activating both alpha-1 and alpha-2 receptors, while brimonidine eye drops are relatively selective with greater activity for the alpha-2 receptor. These differences in relative selectivity result in variation in both drug potency and side effect profiles for these agents. For example, nonselective beta-blockers such as timolol lower intraocular pressure more than beta-1 selective agonists such as betaxolol, but betaxolol is less likely to have an adverse effect on the respiratory function than timolol. Similarly, apraclonidine causes more allergic dermatitis and hyperemia than brimonidine, likely due to it being less selective in its receptor class activation. “Original” versions of ophthalmic medications, both for glaucoma and other eye diseases, have often been improved upon in second- and third-generation varieties by making them more selective for the receptors they activate.
Some of the parasympathetic nerve ganglia begin inside the skull (cranial nerves CN III, oculomotor nerve; CN VII, facial nerve; CN IX, glossopharyngeal nerve; and CN X, vagus nerve). Other parasympathetic ganglia originate from the sacral region of the vertebral column (spinal nerves S2 and S4). About 75 % of all parasympathetic nerve fibers belong to the vagus nerve.
Similar to the sympathetic nervous system, there are several receptor subtypes within the parasympathetic system (see Fig. 23.5). The parasympathetic nerves release acetylcholine as the neurotransmitter. Nicotinic receptors are found at the synapses between pre- and postganglionic neurons. Muscarinic receptors are found on all effector cells stimulated by postganglionic parasympathetic fibers. Five different types of muscarinic receptors have been described. All five receptor classes are found within the central nervous system, while receptors M1–M4 are found in various tissues [42]:
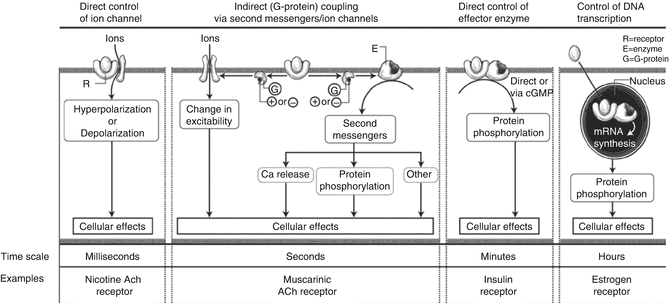
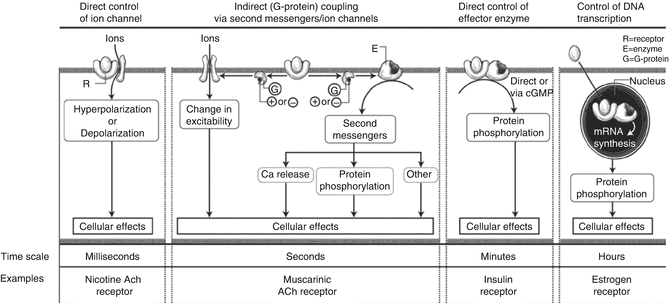
Fig. 23.5
Parasympathetic receptor subtypes
M1 – secretory glands.
M2 – heart muscle.
M3 – smooth muscles and glands. Pilocarpine acts here.
Medications for Glaucoma Therapy
Pharmacodynamics is the branch of medicinal chemistry that is concerned with how a drug works, its mechanisms of action, and the physiologic and medically beneficial effects of the drug. Drugs work by binding to regulatory macromolecules, such as the receptors we have described in the previous section, or in some cases by attaching to and working with enzymes. Recall that drugs that bind to receptors may be agonists or antagonists (or in some cases have mixed action). Pharmacokinetics, however, is concerned with the “where” and “when” of drug functionality, absorption, distribution, metabolism, and excretion of medications.
Glaucoma drugs come in many preparations. Topical medications for glaucoma are available as aqueous solutions, suspensions, ointments, inserts placed within the fornices of the eye, and gels. Punctal plugs may be used in the future to administer glaucoma medications [58]. Systemic medications used to treat glaucoma also have several routes of administration, oral, intravenous, intraocular injections, and periocular injections [59] (e.g., anecortave acetate [59], although it has been withdrawn as an active clinical candidate by its sponsor, Alcon Laboratories). While the ophthalmologist is most concerned about the activity of the medication contained within the bottle, inactive ingredients in the container help adjust the pH, viscosity, and osmolarity (effective tonicity of dissolved concentrates) of the vehicle to facilitate delivery and stability of the medication. The normal pH of tears is 7.4; if the drug vehicle pH is much different than that, then reflex tearing will occur. The addition of methylcellulose and polyvinyl alcohol enhances drug absorption by increasing corneal contact time.
The US Food and Drug Administration requires all multidose containers to have adequate protection from contaminating bacteria and yeasts, and as described earlier, the most common [60] of these in ophthalmic preparations is benzalkonium chloride (BAK).
Choosing a Drug for Primary Therapy of Glaucoma
What are the characteristics of an ideal drug for glaucoma therapy? Primarily, both the physician and the patient want a drug that is efficacious in preserving visual acuity and visual field, that is, they want a drug that works! They want drugs that will slow down or halt the progression of glaucoma, both structurally and functionally. Second, glaucoma medications ideally should be extremely “patient friendly,” so that patients will be inclined to use them. The former US Surgeon General, C. Everett Koop, purportedly has commented “Drugs don’t work in patients who don’t take them,” a succinct statement of the importance of medication compliance.
Tables 23.2 and 23.3 summarize both the efficacy- and compliance-related factors desirable in glaucoma medications. Some of these attributes are present in the medications available today; some are on the horizon and some in the more distant future. As we look at the different classes of glaucoma medications and evaluate their pros and cons, keep in mind the various factors listed in these two tables.
Table 23.2
Efficacy characteristics of an ideal glaucoma drug: “the drug works”
Available in current drugs | Future availability | |
---|---|---|
Maximum IOP lowering as monotherapy | Prostaglandin class lowers IOP about 30 % [61] | New classes of receptors and intraocular target sites undergoing research |
High responder rates to desired target IOP | PGAs and beta-blockers have response rates (as monotherapies) of 50–70 % | Genetic profiling may help select high-response drugs for individual patients [62] |
Flat 24-h IOP curves (low IOP fluctuation) and diurnal and nocturnal control of IOP | PGAs have fairly flat 24-h IOP curves; beta-blockers do not work at night 87 | |
No tachyphylaxis (short-term) or long-term drift | New classes of receptors and intraocular target sites undergoing research | |
Increase ocular blood flow – ocular perfusion pressure | ||
Disease-modifying agents (e.g., “fix” the TM or other outflow pathways) | None at the moment | |
Rho-kinase inhibitors [87] | ||
Neuroprotection by direct action on retinal ganglion cells | “Neuroprotection” has been the elusive holy grail of glaucoma, much searched for but to date not found. The hunt continues |
Table 23.3
Compliance characteristics of a desirable glaucoma drug: “patients will adhere to its use”
Presently available | Future availability | |
---|---|---|
Once-a-day or less frequent dosing intervals | PGAs, beta-blockers – Q day | Look for better than once-a-day applications in the future (anecortave acetate?) [59] |
Multiple medications in one container – better compliance, less total preservative dose | Combination products | In development |
Cosopt | ||
Combigan | PGA/beta-blocker combinations available outside the USA | |
Long duration of action in case of missed doses | Ongoing research | |
No BAK or other detergent-type preservatives | Alphagan-P | Many future glaucoma drugs will have newer, non-detergent preservatives |
Travatan Z | ||
Timolol Ocudose | ||
Easy to hold/use bottle and/or dosing aid | Yes – but patients vary in their preferences for containers | Improvements are possible in addressing issues such as pliability |
No systemic side effects | Timolol – many | Ongoing research |
PGAs – few | ||
Alpha-agonist – moderate | ||
TCAIs – few | ||
No topical side effects | Timolol – few | Ongoing research |
PGAs – hyperemia, periorbital skin hyperpigmentation, lash growth | ||
Alpha-agonist – conjunctivitis, dermatitis – common | ||
TCAIs – stinging (dorzolamide), dermatitis | ||
Compliance monitoring readily available | No – efforts have been (e.g., Travatan Dosing Aid) made but no device is in widespread clinical use [85] | Yes – bottle communication with wireless bus at home or concept of a “universal medical bus” for medications to communicate |
Cost and insurance coverage | Generic drugs are available | More on the way |
Tolerability and safety also are important aspects for choosing and continuing glaucoma medications. While tolerability is often evident based on patent symptoms (e.g., hyperemia, itching), potential safety issues may be substantially more subtle. Often new and established patients complete medical history questionnaires that also contain a “review of systems.” These documents are helpful because they suggest to the treating physician areas of possible systemic side effects with potential glaucoma medications, but they cannot entirely substitute for a thorough interview with the patient by the eye doctor. The danger of increasingly high clinic volumes of patients and shorter patient interviews is that important systemic medical issues will be missed that may result in the patient sustaining side effects. As glaucoma consultants, we have too often seen referred patients who come into our offices on topical beta-blockers, started by their community ophthalmologists who had not inquired about any history of asthma or chronic obstructive pulmonary disease (COPD). While a detailed medical history questionnaire is very helpful, the personal interaction between the patient and the physician results in a better likelihood of uncovering potential medication/medical history interactions and adverse effects.
Choice of the Initial Agent
As explained earlier in this chapter, the rationale for treatment of glaucoma is based on the assumption that lowering intraocular pressure currently is the most important thing we can do for the patient. At the present time, benefits such as improved blood flow or direct neuroprotection of retinal ganglion cells are secondary considerations when choosing primary monotherapy for patients with ocular hypertension or open-angle glaucoma. Pilocarpine was introduced in the late nineteenth century to treat glaucoma. Figure 23.6 shows the timeline of development for glaucoma medications used in the USA.
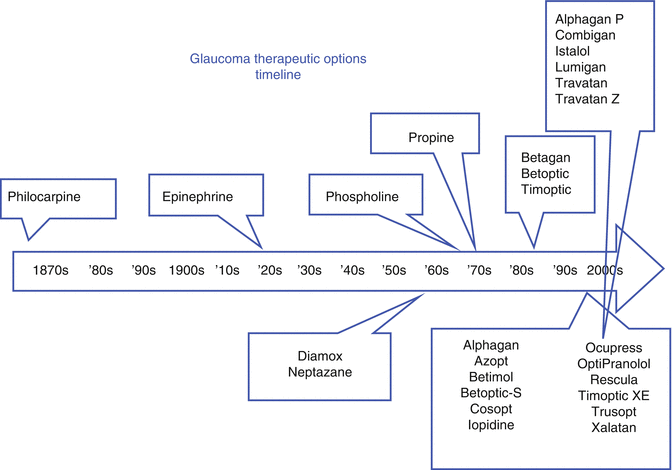
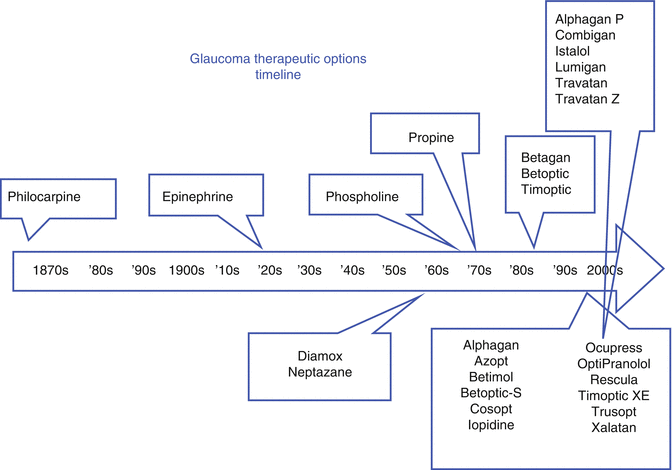
Fig. 23.6
Glaucoma therapeutic option timeline
Medications for the treatment of glaucoma may be divided into six classes (seven classes if we include fixed combinations of products) as shown in Table 23.4. Figure 23.7 depicts the relative potencies of glaucoma agents with respect to lowering intraocular pressure.
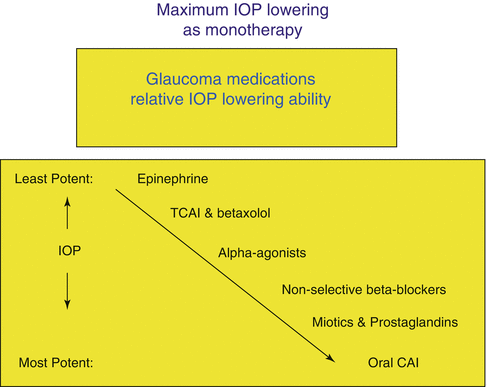
Table 23.4
Glaucoma drug treatment options
Beta-adrenergic antagonists |
Hypotensive lipids |
Carbonic anhydrase inhibitors |
Topical, oral |
Sympathomimetic agonists |
Nonselective, selective |
Parasympathomimetic agents |
Miotics |
Fixed combinations |
Hyperosmotic agents |
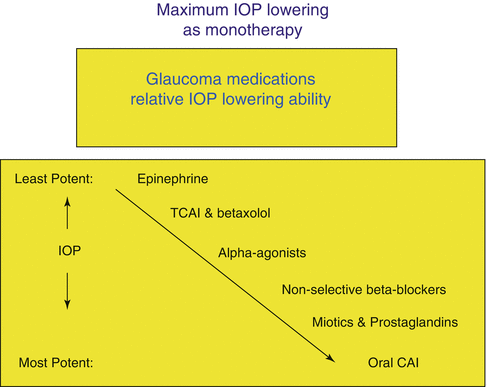
Fig 23.7
Maximum IOP lowering as monotherapy
A meta-analysis of 28 randomized clinical trials [95] summarizing the relative effectiveness of the more commonly used glaucoma medications for lowering IOP is shown in Table 23.5.
Table 23.5
Intraocular pressure-lowering effects of glaucoma medications based on a meta-analysis of 28 randomized clinical trials through 2003
IOP-lowering effects of common glaucoma medications | ||
---|---|---|
Peak IOP (%) | Trough IOP (%) | |
Betaxolol | −23 | −20 |
Timolol | −27 | −26 |
Dorzolamide | −22 | −17 |
Brinzolamide | −17 | −17 |
Brimonidine | −25 | −18 |
Latanoprost | −31 | −28 |
Travoprost | −31 | −29 |
Bimatoprost | −33 | −28 |
Beta-Adrenergic Antagonists
As early as 1967, it was found that the nonselective beta-blocker propranolol reduced IOP when administered intravenously [96]. A paper by Katz et al. in 1976 demonstrated that topical timolol reduced IOP in normal volunteers [97]. From the late 1970s through the beginning of the twenty-first century, beta-adrenergic antagonists (“beta-blockers”) became the mainstay of initial clinical treatment for lowering intraocular pressure in glaucoma patients. Timoptic was introduced by Merck in 1978. This and other nonselective beta-blockers reduce IOP by about 20–25 % by suppression of aqueous humor formation. These drops inhibit cyclic adenosine monophosphate (cAMP) in ciliary epithelium. Carteolol has “intrinsic sympathomimetic activity” (ISA) and subsequently may have less effect on lipid profiles than other nonselective beta-blockers [98–100]. Only the generic version of carteolol is sold in the USA. There have been reports of anterior uveitis in patients using metipranolol, persisting even after its reformulation [101–108]. This drug has fallen out of favor in the USA.
Beta-blockers are available in solutions, gel-forming solutions, and suspensions. In the USA, the following brands are available:
Generic/Brand Names
Nonselective (B1–B2 blockade) – timolol maleate (Timoptic, Timoptic-XE, Timoptic Ocudose, Timolol GFS, Istalol); timolol hemihydrate (Betimol), levobunolol (Betagan); metipranolol (OptiPranolol 0.3 %), carteolol HCl (Ocupress 1 %)
Selective (B1 >> B2 blockade) – Betoptic S .25 %
All are available in both 0.25 and 0.50 % concentrations except as noted. Most of the pressure reduction activity of these drugs is mediated through their action on the beta-2 receptors. Nonselective beta-blockers lower IOP on average 20–25 %. Although most ophthalmologists prescribe the 0.50 % concentration of the nonselective beta-blockers, adequate aqueous concentrations are achieved with lesser amounts of drug, and the 0.25 % commercial preparations are close to the peak of the dose–response curve [109]. These high aqueous concentrations of drug, even with the 0.25 % dose, explain why betaxolol, which is highly selective for the beta-1 receptor, reaches a sufficient concentration to lower intraocular pressure, which is mediated through the beta-2 receptor [110–113]. Betaxolol lowers IOP 15–20 %, but it does not lower pressure as much as the nonselective beta-blockers (20–25 %) [114–117].
All of the listed medications use benzalkonium chloride as the preservative, except Timoptic-XE and Timolol GFS, which use benzododecinium bromide 0.012 % (also a quaternary ammonium compound), and Timolol Ocudose, which is supplied in preservative-free unit-dose 0.2 ml containers. Approved dosing per the package inserts is every 12 h, except for once-per-day dosing (usually in the morning) for Timoptic-XE and Timolol GFS and for Istalol, which contains sorbic acid and is more lipophilic. The greatest activity of the medications occurs between 2 and 4 h after instillation.84 Beta-blockers take about 4 weeks to fully leave the body.
Beta-blockers have little activity during the evening and nighttime hours, when aqueous production by the ciliary body is reduced because of natural circadian factors [38, 63, 118]. Circulating catecholamine concentration (especially epinephrine) is lowest at night, resulting in a deceased sympathetic tone that appears necessary for the production of aqueous. It has been hypothesized that if there is a low sympathetic tone, little aqueous is being produced above a basal level, and thus there is little for the beta-antagonist drugs to “block.” [119] Thus, beta-blockers do not provide effective 24-h control of intraocular pressure [63]. Therefore, drugs that affect aqueous outflow (e.g., hypotensive lipids), rather than aqueous production, may be more effective agents at reducing nocturnal intraocular pressure.
While many beta-blockers in solution form are approved for twice-a-day dosing, most of the benefit of this class of medication can be achieved by a single morning dose [120–122]. Because beta-blockers do not appear to work as well at night, the evening dose is superfluous and increases the risk of side effects. Also, there is some concern that nocturnal effects of beta-blockers may lower ocular perfusion pressure [71, 79, 123–135]. This would be especially worrisome in patients with normal-tension glaucoma. There is some evidence that betaxolol may increase retinal and choroidal blood flow by acting on voltage-dependent calcium channels [132, 136–145]. It may thus have some neuroprotective effect separate from its IOP-lowering properties [146, 147]. Levobunolol has an active metabolite (dihydrobunolol) that may contribute to its longer duration of activity when compared with timolol [148, 149].
Topical beta-blockers have few ocular side effects [150, 151]. Istalol and Betimol sting more than other products, and Timoptic-XE and timolol gel-forming solution (Timolol GFS) may blur vision upon installation. Betoptic S comes as a suspension that helps minimize stinging [152]. All topical beta-blockers cause some corneal anesthesia and punctuate staining [153]. Although their ocular side effects may just be a nuisance, their systemic side effects may be dramatic and even life-threatening in some instances. As described earlier in this chapter, there are two major forms of beta-agonist activity: beta-1, which largely affects cardiac muscle, and beta-2, which largely affects pulmonary function. Well-known systemic side effects of beta-blockers limit their use in glaucoma patients who suffer from respiratory ailments. Betaxolol is a relatively selective beta-1 antagonist and is thought to be safer than the nonselective beta-blockers for use in patients with pulmonary conditions. However, no beta-blocker is safe enough to use in patients with severe reactive airway disease. Fortunately, for these patients, we have a number of good alternative preparations for both primary and adjunctive glaucoma therapies.
Recently, congestive heart failure (CHF) has become only a relative contraindication to the use of oral beta-blockers systemically [154–158] and similarly for the use of beta-blocker eye drops. Nonetheless, it is prudent to check with the patient’s family physician or cardiologist before instituting such therapy in patients with known CHF. Steps taken to limit the systemic side effects of beta-blockers include reduced concentrations (0.25 % compared to 0.5 %) and beta-1-selective preparations (e.g., betaxolol), along with nasolacrimal duct occlusion. Topical beta-blockers used to treat glaucoma are less effective at lowering IOP in patients already taking oral beta-blockers [159, 160]. This “double dosing” by topical and oral routes significantly increases the risk of systemic side effects. In most cases, patients using oral beta-blockers should not receive topical beta-blockers as their initial glaucoma therapy.
Because of these scientific issues and medical–legal concerns, when prescribing beta-blocker eye drops, physicians must be acutely aware of their potential interaction with the patient’s general medical problems. Before initiating therapy with a topical beta-blocker, the patient’s pulse rate and systemic blood pressure should be checked and recorded in the medical record. This should be repeated at subsequent office visits. Ask about a history of heart block, CHF, bradycardia, and any incidences of fainting [161]. Patients also should be asked about smoking, asthma, and COPD [161]. Patients using topical beta-blockers may exhibit decreased exercise tolerance, less so with beta-1-selective agents [162–167]. As with oral beta-blockers, lipid profiles may be affected unfavorably with decreased high-density lipoprotein (HDL) and increased triglycerides [168]. The patient’s lipid profile may be less affected with Ocupress, which has intrinsic sympathetic activity (ISA) [98]. Topical beta-blockers can produce central nervous system effects including depression, anxiety, confusion, hallucinations, drowsiness, weakness, memory loss, and impotence, and frail elderly patients can become “weak and dizzy.” [169] Finally, alopecia has been reported with topical beta-blockers (package insert, Timoptic Ophthalmic Solution, Merck & Co., West Point, Pennsylvania).
Beta-blockade from topical preparations may mask symptoms of diabetic-related hypoglycemia [170] and cause impaired glucose tolerance (inhibit lipolysis and glycogenolysis) [171–179]. Beta-blockade can mask symptoms of thyrotoxicosis and abrupt cessation may cause it [180–184].
Patients with dark irises, including African-Americans, may have less intraocular lowering pressure effect from beta-blockers because of binding of the medication to melanin [185–188]. (This is also true for pilocarpine [189] and adrenergic agonists [190] used to treat glaucoma.)
Topical beta-blockers applied unilaterally may exhibit some “crossover” of IOP lowering in the contralateral eye [191–194]. This makes a “one-eyed” trial of a beta-blocker somewhat difficult to evaluate.
These agents generally do not exhibit tachyphylaxis or short-term “escape,” although one early report [66] suggests tachyphylaxis does occur. There is more controversy, however, about long-term drift of IOP with topical beta-blockers [67, 68, 195–199]. A recent well-designed study suggests that long-term drift of beta-blockers is an urban myth among ophthalmologists [69].
Hypotensive Lipids
Hypotensive lipids (HLs) for treating OAG fall into three subcategories. The prostaglandin analogues (PGAs) include latanoprost (Xalatan 0.005 %) and travoprost (Travatan and Travatan Z, both 0.004 %) and tafluprost (Zioptan 0.0015 %); there is one prostamide – bimatoprost (Lumigan 0.03 %); the docosanoid class is represented by unoprostone isopropyl (Rescula 0.15 %). They are all derivatives of prostaglandin F2α, based on pioneering work by Bito, Stjernschantz, and Camras [200–203]. This class of glaucoma medications increases both trabecular-meshwork and uveoscleral outflow [204–206] and is less affected by circadian variations in aqueous production than the beta-blockers [91, 207]. Although these drugs have this dual mechanism of action, most of the increased outflow facility can be attributed to their effects on the pressure-independent uveoscleral outflow pathway [206].
Bimatoprost, latanoprost, travoprost, and tafluprost are approved as first-line agents by the FDA in the USA. Unoprostone isopropyl is very infrequently used as either first-line or adjunctive therapy in the USA, although it is popular in Japan where it was developed. It requires twice-per-day dosing (the other hypotensive lipids are dosed once per day) and lowers IOP to a much lesser extent than the other products of this class. Unoprostone was removed from the US market for several years but has been recently (2012) reintroduced. According to the package insert, “Unoprostone also lowers IOP by its effect on FP receptors and may have a local effect on BK (Big Potassium) channels and ClC-2 chloride channels, but the exact mechanism is unknown.”
Unoprostone has a very limited market share in the USA, likely because of its lesser ability to lower IOP and its increased (BID) dosing interval compared to the other hypotensive lipids.
Unless noted otherwise, the phrase “hypotensive lipids” (HLs) subsequently used in this chapter shall refer only to bimatoprost, latanoprost, travoprost (with or without BAK), and tafluprost.
The hypotensive lipids have become accepted by ophthalmologists as first-line therapy in the USA, Europe, and South America for most forms of open-angle glaucoma. Cost considerations and later introductions have limited their use in Asia and Africa, where beta-blockers and miotics are still popular.
Early drugs developed to control glaucoma either decreased aqueous production (beta-blockers) or increased conventional, trabecular-meshwork-mediated outflow (miotics). The major innovation of the HL medications is their ability to increase uveoscleral outflow (with a small to moderate effect on TM function as well). The prototype molecule, prostaglandin F2α, does itself lower IOP, but it was found to produce too much inflammation and ocular symptoms intolerable to patients and was thus never developed into a commercial product [201]. Molecular engineering has led to derivatives of this parent compound with acceptable efficacy and safety profiles.
Most scientists agree that the mechanism of action for increased uveoscleral outflow involves activation of the FP class of prostaglandin receptors found at the iris root and ciliary body. Stimulation of these receptors results in the upregulation of matrix metalloproteinases (which are similar to collagenases) that in turn result in a remodeling of extracellular matrix and widening of intermuscular spaces in the ciliary body. Aqueous may then more easily exit the eye around the cellular structures [208–211]. A study in FP-receptor-deficient mice, with presumed functional uveoscleral outflow pathways, showed no affect of any of the four hypotensive lipids on reduction of intraocular pressure [212].
How do the commercial preparations differ? As described earlier in this chapter, all of the HLs function as prodrugs. Latanoprost, travoprost, tafluprost, and unoprostone are esterified derivatives of prostaglandin F2α, while bimatoprost contains an amide group in the same position as the isopropyl ester of the other molecules. The chemical structures of the compounds (except for tafluprost) are shown in Fig. 23.8.
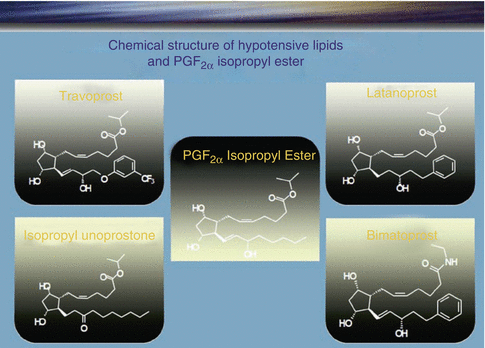
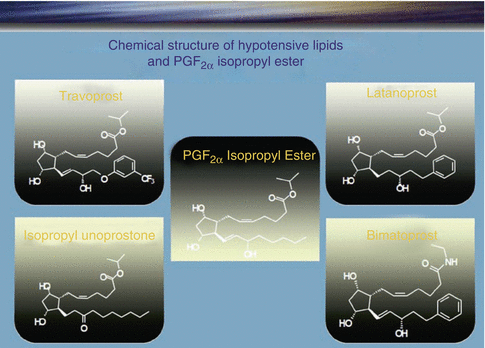
Fig. 23.8
Structure of the hypotensive lipids (Courtesy of Alcon Labs, Fort Worth, Texas)
The ester or amide portions of the molecules are cleaved by abundant corneal enzymes, with the free acid forms of the lipids entering into the anterior chamber in sufficient concentration to activate the FP prostaglandin receptors of the ciliary body that is bathed in aqueous. The ester moiety is easily cleaved, allowing the drugs to be manufactured at relatively low concentrations (latanoprost 0.005 %, travoprost 0.004 %, tafluprost 0.0015 %; unoprostone has a higher concentration 0.15 % but is a relatively ineffective drug – we will not consider it in subsequent discussion). The amide group on the bimatoprost molecule is not as easily separated from the free acid [213]. The clinical product used to treat glaucoma has a concentration (0.03 %) six to eight times greater than the other HLs. This increased concentration allows sufficient free acid to enter the eye to effectively activate the FP receptors [214]. Thus, it would appear that bimatoprost lowers IOP by uveoscleral outflow in the same manner as the other HLs.50 [215], However, the manufacturer (Allergan, Irvine, California) has sponsored research suggesting that the entire bimatoprost molecule acts primarily by passing through intact sclera to activate “prostamide” receptors on the ciliary body with subsequent increase in uveoscleral outflow facility [216]. There is one study using a preparation of feline iris sphincter that demonstrates binding of the full bimatoprost molecule to receptors that appear to be distinct from prostaglandin FP receptors [217]. (And recall the murine study described above that showed no reduction in IOP when bimatoprost was applied to the eyes of FP-receptor-deficient mice.186) No published studies have found these receptors in the ciliary body nor has a prostamide receptor been “cloned” using standard biological techniques. Bimatoprost has been used clinically since 2001. While prostamide receptors may exist, clinically bimatoprost seems to function as a prostaglandin analogue, with increased activity of both the conventional and nonconventional outflow pathways. The authors look forward to a research that may demonstrate the presence of the receptors in the relevant tissues and their binding and activation by the nonhydrolyzed version of bimatoprost. From a clinical perspective, in our practices, we consider the three major HLs as belonging to the same class of medication (Fig. 23.9).
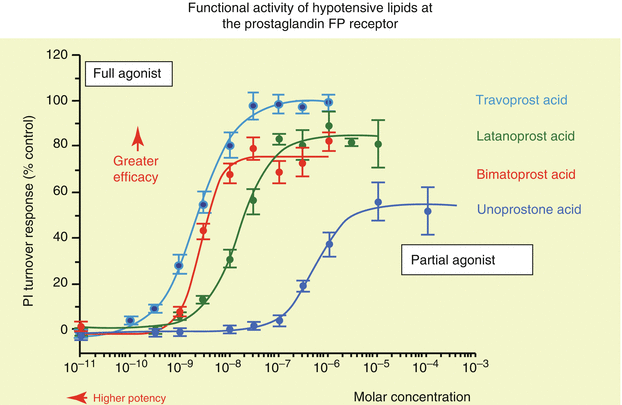
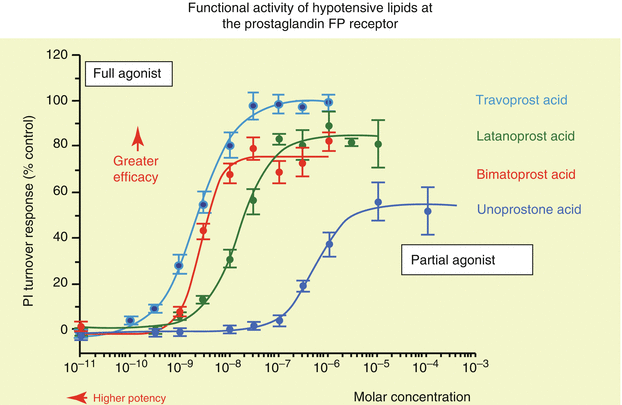
Fig. 23.9
Functional activity of hypotensive lipids at PG FP receptor (Courtesy of Alcon Labs, Fort Worth, Texas)
Variations in the PGF2α molecule result in changes in potency and side effects. Latanoprost was the first HL to be developed commercially (by Pharmacia, now Pfizer). It became available in the USA in 1996. In order to reduce the hyperemia associated with PGF2α, the unsaturated (double) bond between carbons 13 and 14 was saturated. This resulted in some loss of potency but by reducing hyperemia made the drug acceptable to real-world patients. Because there is no major clinical difference in IOP-lowering efficacy whether this class of drugs is dosed daytime or nighttime, it has become customary to prescribe the HLs at bedtime, so that the majority of the immediate hyperemia associated with drug dosing occurs while the patient is asleep. These drugs do have some “chronic” hyperemia that tends to subside over several months of use. Occasionally, patients prefer morning dosing, which is acceptable from an efficacy perspective. Clinical IOP-lowering efficacy of the HLs is better with QD dosing (at the commercially available concentrations) rather than BID dosing [218–220]. Systemic half-life of the drugs is brief (e.g., latanoprost 17 min). The peak plasma concentration of latanoprost is about 10−10 M or less, and there is little if any effect of the drugs on the IOP of the contralateral eye when dosed unilaterally [221].
Both bimatoprost and travoprost came to the US market in March 2001. Recently, two others were approved. Tafluprost (Zioptan) is available in a single-use, preservative-free form. Unoprostone (Rescula) which had been withdrawn from the US market but reintroduced recently (2012) is a significantly weaker drug in the same class of FP-receptor agonists. It is purported to have additional mechanisms of action which may distinguish it from its more powerful competitors in this class of agents. To improve efficacy, Alcon Laboratories modified the PGF2α molecule to create travoprost by adding a CF3 on the unsaturated benzene ring. This allows for a tighter bonding of the travoprost free acid to the FP receptors [222, 223]. This results in a longer-duration, clinically useful, IOP-lowering effect of both original travoprost and the BAK-free version [91, 92, 224]. This could be important in patients who occasionally miss doses.
Alcon kept the double bond between carbons 13 and 14, increasing efficacy but resulting in more hyperemia for travoprost than found in the commercial latanoprost preparation (the newer travoprost without BAK has slightly less hyperemia than the original product, but both versions produce more clinical hyperemia than latanoprost) [48, 225]. Most of the hyperemia associated with the HLs results from dilated conjunctival vessels in response to direct activation of FP receptors found in the vasculature muscle walls [226]. This is not a toxic or allergic response but is, however, of cosmetic concern to the patient. Allergan, when designing bimatoprost, also kept the unsaturated double bond between carbons 13 and 14 and added the amide group, where the other products have the isopropyl ester. As discussed earlier, bimatoprost has a six- to eightfold greater concentration than the other HLs. This may be related to the clinical observation that bimatoprost causes more red eye than the other two products [225, 227, 228]. A newer version of bimatoprost [229] lowers the IOP with a decreased concentration (by one-third) and increased BAK resulting in less hyperemia likely due to decreased concentration of drug (0.01 % compared to 0.03 for the original concentration) while increasing the penetration of the drug by increasing the BAK fourfold. This is an example of a logical, obvious trade-off to get less side effect with less drug, but it occurs at the expense of using BAK (see Sidebar 23.4). (Latanoprost causes the least conjunctival hyperemia, a bit more with travoprost.) [230]
Additionally, the HLs activate more than just the FP class of prostaglandin receptors. The EP class of prostaglandin receptors mediates inflammatory by-products. Funk in a recent (2001) review [231] of eicosanoid biology said, “Prostaglandins act both at peripheral sensory neurons and at central sites…to evoke hyperalgesia. Recent data support involvement of … EP1 receptors in pain” [232–234]. Figure 23.10 shows the relative binding affinities for the HLs at both FP and EP1 receptors. It is desirable for glaucoma drugs to have high binding affinities for the FP receptors (to lower IOP) and low binding affinities to the EP1 receptors (to minimize inflammation). Here, we see that bimatoprost is a better agonist at the EP1 receptor than the three other products. Thus, the increased hyperemia seen with bimatoprost may result from both its affinity for EP1 receptors (inflammatory hyperemia) and its relatively higher concentration (vasodilatory hyperemia in response to FP-receptor activation). From a clinical perspective, most patients find that the hyperemia of all the HLs decreases sufficiently over a month or two to become cosmetically acceptable [235, 236]. We have found that prospectively discussing conjunctival hyperemia with patients before initiating HL therapy greatly decreases their emergent phone calls and “drop-in” office visits.
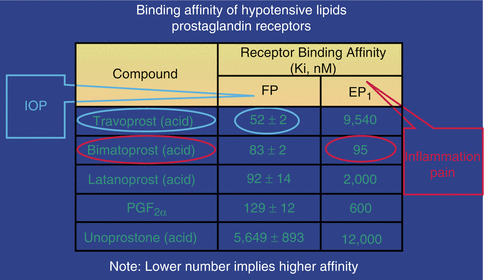
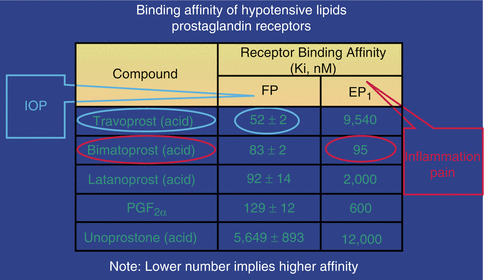
Fig 23.10
Binding affinity of hypotensive lipids at prostaglandin receptors (Courtesy of Alcon Labs, Fort Worth, Texas)
While conjunctival hyperemia is clinically apparent to the patients, their companions, and the treating physician, other aspects of ocular surface disorders may be less obvious but even more important to chronic disease management. As noted earlier in this chapter, the BAK found in most glaucoma medications may play an important role in causing the signs and symptoms of ocular surface disease and subclinical inflammation (see Sidebar 23.4). The newer formulation of travoprost without BAK, with the preservative SofZia, seems equally effective as the original product but with improved ocular surface health [11, 48]. New glaucoma products without BAK are under development by the pharmaceutical industry.
The three hypotensive lipids lower intraocular pressure on average between 25 and 30 %. This is greater than the nonselective beta-blockers, which lower IOP between 20 and 25 %. The only randomized controlled clinical trial directly comparing the three agents found them to be on average about the same in IOP-lowering efficacy (with a slight, 0.5 mmHg, advantage to bimatoprost) and confirmed the clinical impression that latanoprost had the lowest rate of conjunctival hyperemia [61]. The previously mentioned meta-analysis by van der Valk of 28 clinical studies also shows the HLs to be roughly equivalent in their ability to lower intraocular pressure [95]. A more recent meta-analysis of nine studies that had to compare at least two prostaglandin analogues as monotherapy found that travoprost and bimatoprost lowered IOP −0.98 mmHg (95 % Cl: –2.08; 0.13; p = 0.08) and −1.04 mmHg (95 % Cl: –2.11; 0.04; p = 0.06), respectively, when compared with latanoprost [237].
The HLs have relatively flat IOP curves over 24 h, demonstrating both low circadian IOP fluctuation and, unlike the beta-blockers, effective diurnal and nocturnal IOP control [63, 91, 92, 207, 228]. They do not evidence short-term escape or long-term drift [238–240].
HLs have few systemic side effects. HLs should generally be avoided in women of child-bearing age without adequate birth control or who are pregnant, because although plasma concentrations of topical HLs are low, there is a theoretical risk of abortion due to the action of prostaglandin molecules on uterine smooth muscle [241–243]. There are no references in the ophthalmic literature that this has ever happened. There are some reports of muscle aches, gastrointestinal upset, and influenza-like symptoms with the use of topical HLs to treat glaucoma [244, 245].
There have been case reports of reactivation of clinically dormant herpes simplex virus (HSV) keratitis by patients who begin HLs, confirmed in some cases upon rechallenging the eyes involved. We generally avoid using HLs in patients with a history of HSV keratitis [246–252].
The use of HLs has been associated with cystoid macular edema (CME) especially in patients who have undergone cataract surgery and intraocular lens implantation, more often if the posterior capsule is ruptured [253–255] (some researchers feel that postcataract CME may be related to eye drop preservatives [256]). Sometimes, this CME is clinically apparent with complaints of reduced visual acuity and contrast sensitivity; sometimes, this CME is picked up by fluorescein angiography or optical coherence tomography. In our experience, this HL-related CME almost always improves by withdrawal of the agent and treatment with topical steroids and nonsteroidal anti-inflammatory drugs (NSAIDs). In our practices, we generally try to discontinue HLs several weeks before cataract surgery and try to avoid reinstituting them for about 3 months after surgery. Fortunately, many open-angle glaucoma patients undergoing phacoemulsification cataract surgery have a “honeymoon” period of reduced intraocular pressure lasting several months or more and may require less glaucoma medical therapy during that time [257–259]. Thus, they suffer no harm by temporarily suspending the HL postoperatively. If the posterior capsule is broken, we may avoid the further use of HLs at all. To attempt to prevent CME, many anterior segment surgeons pretreat all patients about to undergo cataract surgery with topical steroids and NSAIDs, especially if they have been using hypotensive lipids [260]. Some cataract surgeons continue to use previously prescribed HLs throughout the perioperative period [261]. The authors do not advise doing so unless IOP control is lost without the use of HLs in the immediate postoperative time frame.
There have been several studies reporting an association of HLs with the development of anterior uveitis or the worsening of preexisting uveitis [262–270]. Because prostaglandins are proinflammatory compounds, most glaucoma experts do not recommend their use in patients with uveitis, uveitic glaucoma, or neovascular glaucoma. There appears to be little role for HLs in managing an attack of acute angle-closure glaucoma, but these agents do appear useful for various forms of chronic angle-closure glaucoma [271, 272]. Finally, the three hypotensive lipids are not additive in their effects, that is, using, for example, bimatoprost in the AM and latanoprost in the PM will result in slightly worse lowering of IOP than using either drug alone [273].
There are several ocular-related side effects of HLs that may be described as cosmetic. Hyperemia, discussed previously, may be intolerable to some patients. Proactive discussion of this issue may improve patient adherence with HLs. A small to moderate percentage of patients (<20 %) using the HLs develop permanent, clinically noticeable changes in iris color, with increasing plumpness of melanocytes (stimulation of melanogenesis) perhaps through an effect on tyrosinase, but no increase in their number. This change in iris color reflects no mutagenesis but is of cosmetic concern to some patients [274] (not unreasonably, many elderly glaucoma patients are unconcerned about eye color changes if the medications will help save their sight). Purely blue eyes are rarely affected, and fully darker brown eyes show no visibly detectable changes. It is the hazel and green eyes that may become noticeably browner. These color changes may occur with greater frequency than generally supposed, as Teus et al. have shown in a 2002 study comparing patients who received latanoprost in only one eye [275].
These color changes are more frequent with latanoprost than bimatoprost or travoprost (per the package inserts). Again, proactive conversation with the patient about this possibility before starting a hypotensive lipid will avoid unhappiness and potential medical–legal complications. Because of concerns about iris heterochromia (and possible lash growth described below), we almost never prescribe the use of HLs when glaucoma medication is needed only in one eye [275]. Figure 23.11 depicts a patient whose irises are different colors after using bimatoprost in only one eye.
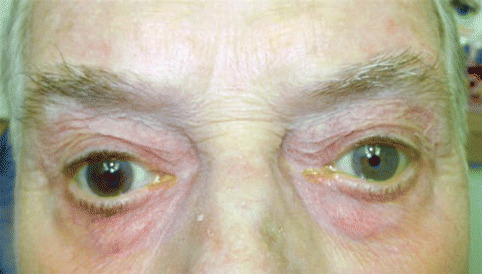
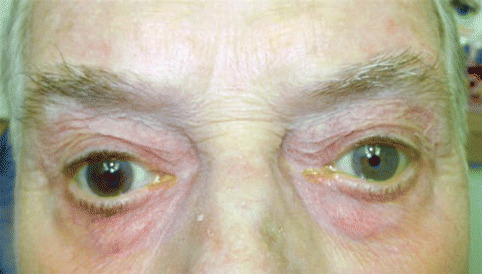
Fig. 23.11
Only the right eye was treated with bimatoprost. The patient had similar hazel irises before treatment. The iris of the right eye is now much darker than the left one (Courtesy of Drs. Tarek Shazly and Mark Latina)
Both hypertrichosis and periorbital skin hyperpigmentation are common side effects for patients who use the topical HLs [276–286]. The lashes may become thicker and longer and increase in quantity (Fig. 23.12). They may become darker although poliosis has been reported [287]. Many women find the lash effects desirable [288], and Allergan now has a commercial product (Latisse) for lash enhancement using bimatoprost 0.03 % as the active ingredient. For those patients who are bothered by the changed lashes, there is good news. Lashes naturally fall out and are replaced about every 12 weeks, so once the medication is stopped, the situation reverses itself [289]. (Recall that induced iris color changes are not reversible.) There is a report of hyperpigmentation and hypertrichosis of the vellus hair on the malar region after unilateral treatment with bimatoprost in a Hispanic patient. These phenomena reversed 2 months after the discontinuation of bimatoprost [290]. Hyperpigmentation of the lids and periorbital skin is more common in people with darker skin (Asian, Hispanics, and African-Americans) than very pale skin. The skin pigmentation changes appear to only affect the epidermis, which, similar to eye lashes, is periodically renewed [283]. Most patients find that undesirable skin pigmentation reverses with cessation of the drug. In our clinical experience, bimatoprost is more likely to affect the lashes and periorbital skin than the other two HLs. Some patients find that using petroleum jelly to coat the lash margins and skin below the lower lids, just before taking their HL eye drops, helps reduce these side effects. In addition to pigmentation, periorbital fat loss has now been reported with this class of agents [291, 292]. It remains a curiosity that it took so long to notice this important problem.
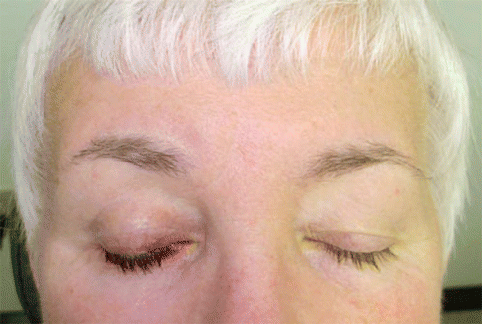
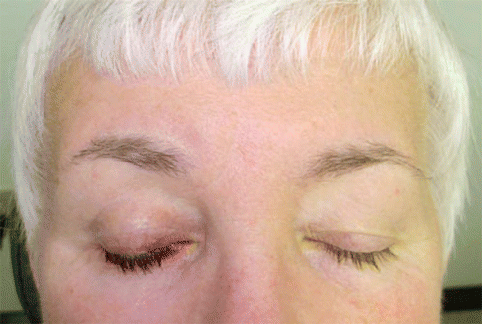
Fig. 23.12
Lash changes with bimatoprost. Only the right eye was treated (Courtesy of Drs. Tarek Shazly and Mark Latina)
Carbonic Anhydrase Inhibitors
The zinc enzymes classified as carbonic anhydrases (CAs) are widespread through the bacteria and plant and animal kingdoms. There are at least 14 known varieties of the alpha-CAs (α-CA) whose main function is the hydration of CO2 to bicarbonate (HCO–). Two of these enzymes are important for the production of aqueous humor by the epithelium of the ciliary processes, cytoplasmic CA II and membrane-bound CA IV [293, 294]. Part of aqueous production involving active secretion relies on the formation of bicarbonate by these enzymes to correct the imbalance caused by the ATPase-fueled transport of sodium into the space between the nonpigmented ciliary epithelial cells. More than 99 % of the activity of these enzymes must be inhibited to successfully decrease aqueous production [295].
In 1954, Becker demonstrated that acetazolamide, a sulfonamide-based carbonic anhydrase inhibitor (CAI), could lower intraocular pressure in human subjects [296]. The IOP-lowering effect of CAIs may be enhanced by the metabolic acidosis they can cause [297]. Acetazolamide is more likely to cause clinically relevant systemic acidosis than methazolamide at therapeutic doses [298]. This may be one of the reasons that acetazolamide at full doses lowers IOP better than full doses of methazolamide. CA isoenzymes are present in many tissues in addition to ciliary processes, including corneal endothelium, iris, retinal pigment epithelium, red blood cells, brain, and kidney. Oral CAIs, while extremely effective in lowering IOP, may have profound medicinal and side effects on the body [295]. For this reason, chronic use of oral CAIs is generally reserved as therapy of last resort for patients who have tried topical medications (and perhaps laser treatments) without adequate control and who for one reason or another are not good candidates for glaucoma surgical intervention. Oral CAIs are also used on a temporary basis for emergent situations with acute rise of IOP. Examples of these situations include early postretinal and early postcataract surgeries and acute angle-closure glaucoma until laser therapy becomes available [299, 300].
Before initiating treatment with oral or topical CAIs, the physician must inquire about sulfonamide allergies. Many patients have been treated with sulfonamide-based antibiotics for bladder infections and may consider any gastrointestinal insult caused by these antibiotics as allergic symptoms. Patients should be specifically asked about breathing difficulties and skin reactions [301, 302], which are the most common form of allergic manifestations to sulfonamide antibiotics. Stevens–Johnson syndrome (erythema multiforme major) has been reported with the oral CAIs [303]. This may be more common in Japanese and Korean patients [304, 305]. Fortunately, there are significant structural differences between sulfonamide antibiotics and the CAIs used to treat glaucoma, but prudence suggests avoiding oral CAIs in any patients with true allergies to any sulfonamide medication [306]. On occasion, we have cautiously used topical CAIs with patients who have an ambiguous history of sulfonamide allergy symptoms. We explain the possible risks, have them use the drops while in the office, keep them around for several hours, and tell them that in the rare event of an allergic reaction, we will call for emergency medical services. In our practices, we have never had a patient who has had an acute event under these circumstances.
Oral CAIs are powerful agents for lowering IOP (between 25 and 30 %) [307] and may do so very well when other medical therapies are unable to reach the target IOP in chronic glaucomas or to temporarily bring IOP to safe levels in acute emergent situations. But their systemic side effects are legion and often serious, in addition to the allergic respiratory and skin problems discussed previously. The limited data available suggest that the topical CAIs (TCAIs), while theoretically capable of causing all the same systemic mischief as the oral agents, have mostly ocular and periorbital side effects.
The two common oral CAIs are acetazolamide (Diamox) and methazolamide (Neptazane).
Diamox is available as “regular” tablets (62.5, 125, and 250 mg) and in timed release, 500 mg “Sequels.” Diamox regular is generally prescribed QID as 250-mg tablets for chronic use or better as Diamox Sequels 500 mg Q 12 h. The Sequels produce less GI symptoms and less fluctuation in IOP over 24 h but are considerably more expensive than the standard tablets that are also generically available. Neptazane tablets are prescribed Q 12 h or TID in either 25- or 50-mg dosages. They do come in generic varieties as well.
In a situation that requires acute lowering of IOP, we often prescribe one regular 250-mg Diamox tablet, with relatively quicker onset of action, and at the same time, we have the patient take a Diamox Sequel for its sustained effect. Patients who may have nausea or vomiting from acutely high IOP, as in angle-closure situations, may not tolerate the oral preparations. Acetazolamide is excreted unchanged by the renal system. Methazolamide undergoes first-pass metabolism by the liver. CAs in the kidney promote the absorption of bicarbonate through the renal tubules. CAIs cause alkalinization of the urine along with increased micturition, both day and night, and potassium excretion. Patients prescribed chronic oral CAIs should have their electrolytes monitored, perhaps with the assistance of their family physician, especially if taking other potassium-wasting drugs such as thiazide diuretics and oral corticosteroids [308, 309]. This is especially important in patients taking cardiac glycosides, such as digitalis, because hypokalemia can be lethal in the presence of digitalis. Systemic side effects are generally dose related except for the blood dyscrasias, which will be discussed later [310]. Kidney stone occurrence may be up to 50 times greater in patients taking oral CAIs than prevalent in the general population [311]. Systemic CAIs cause the kidney to secrete less magnesium and citrate and an alkaline urine. This contributes to the formation of calcium phosphate- and oxalate-based stones.
Methazolamide at lower doses is less likely to cause urolithiasis because of less acidosis and no change in concentrations of urinary citrate [298, 312, 313]. If it is necessary to prescribe oral CAIs, methazolamide is preferred to acetazolamide in patients with a history of kidney stones.
Salicylates interact with oral CAIs. Patients taking high-dose aspirin can get tinnitus, increased respiratory rate, and even confusion and coma [314–317]. They should be warned of these symptoms and told to avoid high doses of aspirin if oral CAIs are prescribed. The low dose of 81 mg of aspirin often prescribed to prevent cardiac events by its antiplatelet effects is probably not of significance with respect to interacting with oral CAIs.
Paresthesias of the fingers, toes, and nose are common with oral CAIs, less so with methazolamide at lower doses [318]. Paresthesias may diminish with time [298]. Patients are less likely to be concerned about these symptoms if they are discussed before the drugs are prescribed.
Gastrointestinal symptoms are also common with oral CAIs, more so with QID regular Diamox than Diamox Sequels or methazolamide [319]. Patients may suffer from abdominal cramps, nausea, and in some cases severe diarrhea [320]. Symptoms may improve as time passes, but some patients must discontinue oral CAIs because of these difficulties. The oral CAIs cause a strange metallic taste with foods and carbonated beverages – patients should be warned this is likely to occur [321]. We have had more than one patient return a case of “bad” beer to the store after initiating acetazolamide therapy [322]. (We apologized to them for our lapse in not discussing this side effect of hypogeusia!) Also note that topical CAIs, when drained onto the tongue via the nasolacrimal duct, may produce “funny” taste sensations for a short time after contact. Lower doses of oral CAIs disrupt taste sensations to a lesser degree than do higher doses.
Patients taking oral CAIs, usually after several months, can have an unexpected onset of a malaise-syndrome complex involving – to varying degrees – tiredness, lack of appetite (which may be accompanied by weight loss), and even severe depression [320]. Generalized apathy may ensue. Patients with mild dementia may seem to get worse. Often, neither the patient nor family members attribute the slow onset of this malaise syndrome to oral CAIs that may have been started several months earlier. The symptoms resolve, slowly, with reduced drug dosage or more often with discontinuation of the offending drug. Similarly, decreased libido and even impotency can result from oral CAIs, again often not attributed to the drugs [323, 324]. Patients and physicians must be aware of these possibilities.
Blood dyscrasias from oral CAIs (none has been reported in the literature from topical CAIs as of mid-2009) are extremely serious events. They fall into two categories: one that is sudden and is irreversible, with a high mortality rate (about 50 % for patients with aplastic anemia), and another that is drug dose dependent and may be reversible with termination of the CAI. Fortunately, the incidence of aplastic anemia associated with oral CAIs is low. Keisu et al. in 1990 [325] estimated it is “approximately one in 18,000 patient years.” Patients present with a constellation of symptoms, including fever, easily bruised skin, purpura, sore throat, paleness, nosebleeds, petechiae, and perhaps jaundice [325, 326]. The use of blood tests to monitor for blood dyscrasias is controversial [327]. In an editorial in 2000 [328], Fraunfelder and Bagby weigh the pros and cons of doing routine, periodic blood tests on patients taking oral CAIs, hopefully to catch early any abnormalities and to be able to cease the drug and treat the reversible cases. Because of the serious nature of these dyscrasias and the fact that only about half of the patients have an irreversible, potentially fatal variation, they recommend that “a standard CBC, consisting of white blood count with differential, hemoglobin, hematocrit, and platelet count, should be repeated every 1–2 months during the first 6-month period and then at 6-month intervals.” In our experience, few ophthalmologists ever get diagnostic blood tests on patients beginning or continuing oral CAI therapy [329]. By custom we do not, but we are fortunate not to have had any patients so affected in our practices. Luckily, the odds against this happening seem to have been in our favor. Physicians may feel the inconvenience and cost of these tests outweigh the probability of finding and successfully treating affected patients. Patients should be aware of the untoward symptoms and report them on an emergent basis. Given all of the above problems with oral CAIs, they should generally be used only temporarily in acute situations of very high IOP or when all other means of treatment have failed and surgery is not a good option.
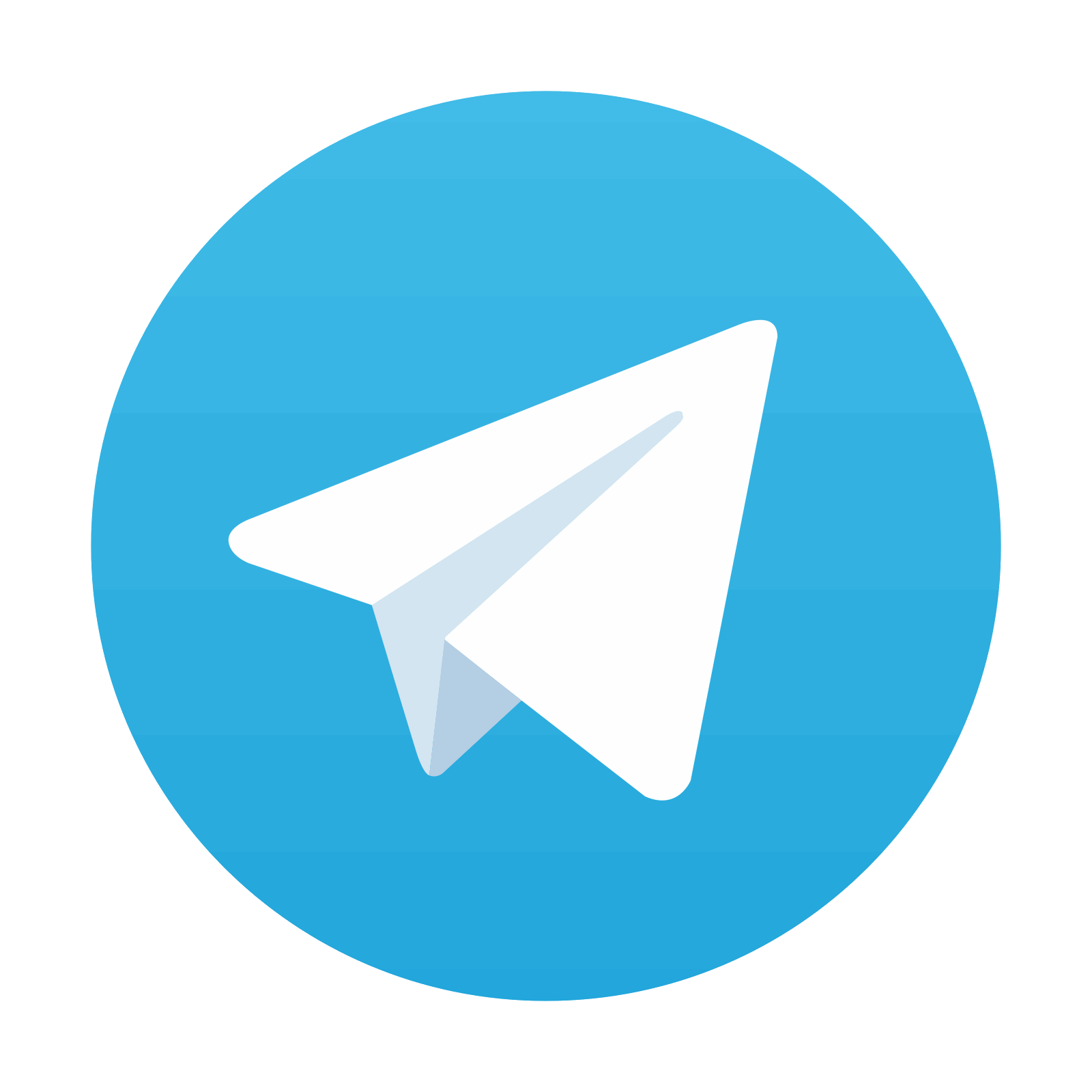
Stay updated, free articles. Join our Telegram channel

Full access? Get Clinical Tree
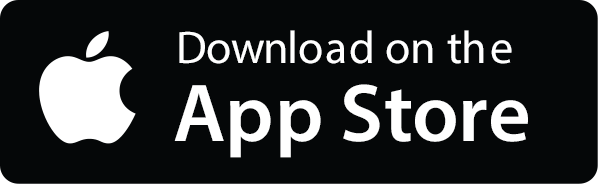
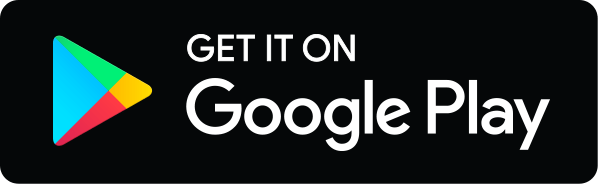