Fig. 46.1
Two principal components were extracted, each consisting of a single frequency profile (left) and corresponding spatial maps for both subject groups (right). The first component (PC1, top) summarized the overall sound-evoked activation, with positive responses to all frequencies (falling off towards the highest frequencies) at all locations in auditory cortex (falling off towards the edges of the activation clusters). Interestingly, the second component (PC2, bottom) summarized preferential activation to low- or high-frequency stimuli, with a monotonic frequency profile and negative/positive coefficients in lateral/medial cortical locations, indicating low- (L) and high- (H) frequency preferences, respectively
The first principal component summarizes the most typical frequency profile and the corresponding magnitudes in all voxels. This component revealed a uniformly positive response to all frequencies, with some roll-off towards high frequencies that indicates a smaller overall responsiveness to high frequencies compared to low frequencies. The corresponding map showed positive coefficients for all voxels, peaking in the general vicinity of the diagonally running HG and declining towards the clusters’ edges. These panels show similar behavior to what would be obtained by straightforward averaging (over all voxels to obtain a mean response profile or over all frequencies to obtain a mean response map).
More interestingly, the second component summarizes the residual after the first component is removed from the activation data. In other words, it shows how voxels most typically tend to deviate from the average behavior that is captured by the first component. This component’s frequency profile shows a monotonic increase, ranging from negative values for low frequencies to positive values for high frequencies. The corresponding map shows negative coefficients on the crest of lateral HG and positive coefficients on the rostral and caudal banks of medial HG. This indicates that the most medially located voxels display a disproportionately large response to high frequencies and a disproportionately weak response to low frequencies and can therefore be classified as “high-frequency voxels”; conversely, the lateral crest of HG contains “low-frequency voxels.” Therefore, this component’s map can be interpreted to represent gradual variations in frequency tuning as a function of cortical position and thus reflects the tonotopic organization in human auditory cortex.
Although we currently report group averages only due to space constraints, we observed that the reported behavior could be traced back to similar tonotopic mappings in individuals quite consistently (Langers et al. 2012).
In Fig. 46.2, the direction of the tonotopic gradient is shown by means of a color code. For the purpose of this plot, frequency tuning was not quantified by the second component’s map; instead, the ratio of a voxel’s coefficient in the second and first component was used, which is more representative of the shape of the response profile, regardless of the overall level of responsiveness to sound.
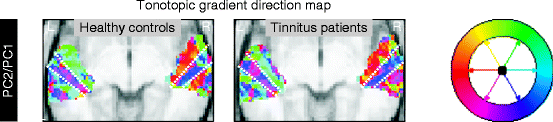
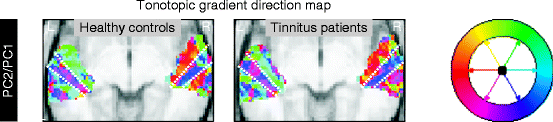
Fig. 46.2
The direction of the tonotopic progression’s gradient in the axial x,y-plane was color-coded, revealing the existence of multiple strips of cortex that run diagonally parallel to HG. Within each such strip, the tonotopic gradient direction is reasonably homogenous, but it abruptly changes when crossing from one strip to the next (dotted white lines)
The gradient can be seen to be oriented in a more or less homogeneous direction inside a number of “strips” that run more or less parallel to HG. At the same time, gradient direction abruptly switches from one strip to the next. For example, on the rostral bank of HG, the low-to-high tonotopic gradient is oriented in a medial direction, whereas on its caudal bank, it points caudally. On the planum temporale, further gradient reversals are visible.
We checked for differences in the tinnitus group by analyzing the histograms of voxel values that could be extracted from the first and second component’s maps, as well as their ratios, and comparing them to bootstrapped null distributions. No significant differences were found to exist compared to healthy controls. These analyses showed, in particular, that auditory cortex as a whole did not display elevated responsiveness to sound in tinnitus patients nor did it show an overrepresentation of high frequencies. The details of these comparisons are reported in Langers et al. (2012).
4 Discussion
In the present study, robust tonotopic maps were demonstrated in response to minimally salient tone stimuli. The tonotopic gradient was found to remain similarly oriented within parallel strips of cortex but abruptly changed direction from one strip to the next. Extrapolating results from primates, in which primary cortical fields were found to show separate tonotopic progressions with gradient directions that were set under a pronounced angle, we surmise that the locations of these reversals correspond with borders between neighboring cortical subfields of human auditory cortex. Our results suggest the presence of at least two distinct subfields on the rostral and caudal banks of HG, rHG, and cHG (Fig. 46.3). We hypothesize that these contain the human homologues of the core fields R and AI in primates.
Our results are in good agreement with the large number of studies that positioned high-frequency areas on medial HG and low-frequency areas on lateral HG, if the limited spatial resolution of these studies is taken into account: because the two medial high-frequency endpoints are positioned relatively closely together, the gradients in rHG and cHG may have been indistinguishable and apparently merged into a single gradient that well aligns with the axis of HG. Given the present detail, however, our results suggest that these supposed primary auditory fields actually line up across HG instead of along HG, while at the same time the gradients within a field are diagonally oriented and set at a pronounced angle. This general arrangement is in close correspondence with the results of various recent studies (Humphries et al. 2010; Striem-Amit et al. 2011; Da Costa et al. 2011) and well fits the organization in primates (Kaas and Hackett 2000; Bendor and Wang 2008). Moreover, the proposed fields reasonably agree with at least a subset of gradients that were proposed by Talavage et al. (2004). At first sight, our results are more difficult to reconcile with a prevalent view in which two abutting gradients span a pair of fields that extend along HG, as demonstrated by Formisano et al. (2003), for instance. This interpretation was based on the frequency profile as obtained along a curve that ran diagonally along HG. When consulting their original maps, evidence for a second high-frequency endpoint on the medial aspect of rHG is visible, however.
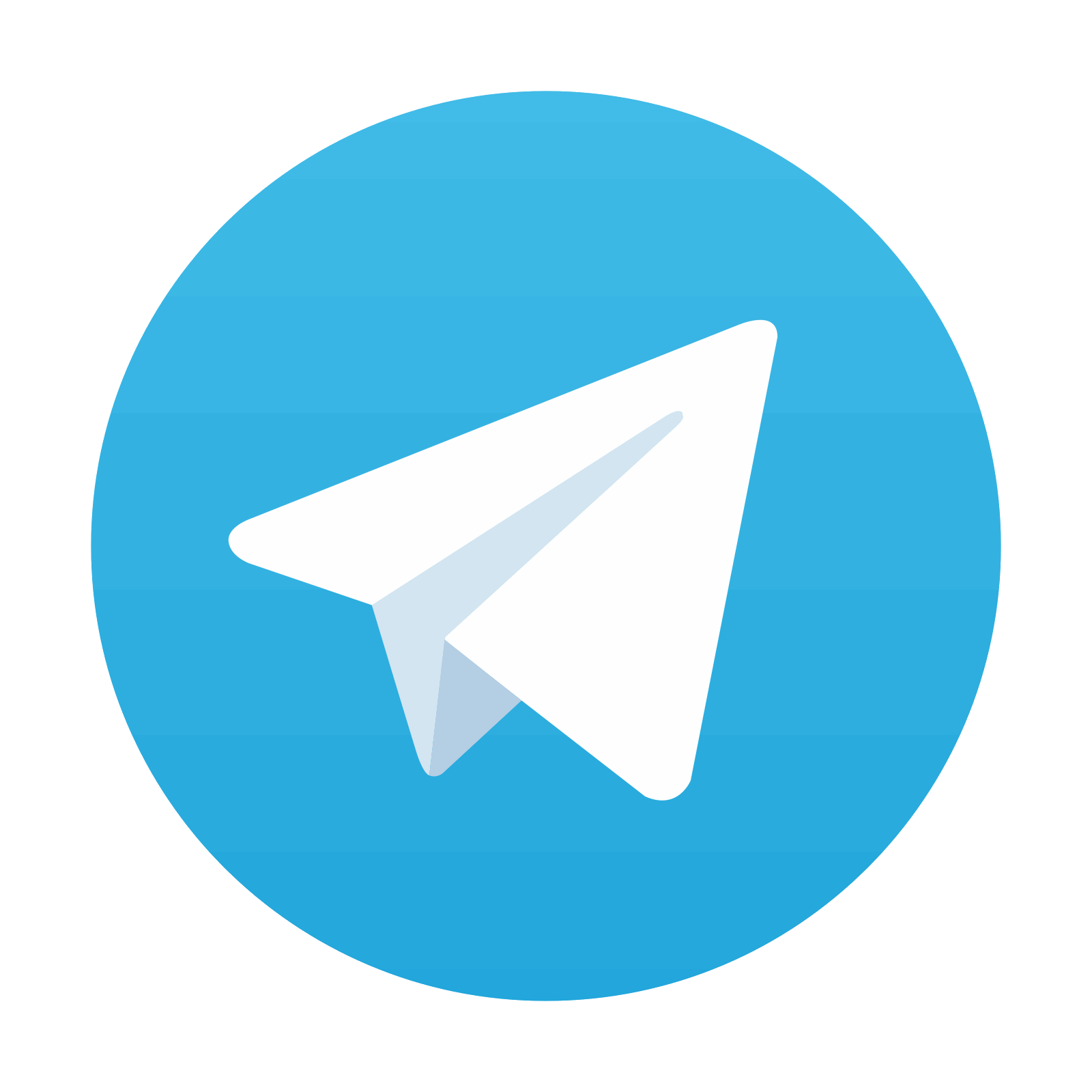
Stay updated, free articles. Join our Telegram channel

Full access? Get Clinical Tree
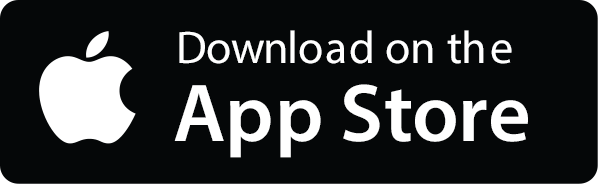
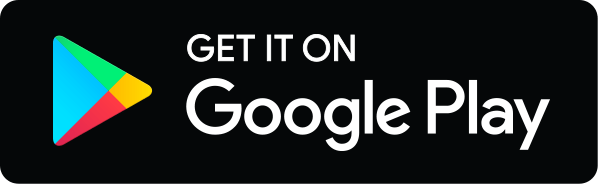