Fig. 1
Human posterior vitreous cortex. Scanning electron microscopy showing a dense network of collagen fibrils. Bar = 10 microns (Reprinted with permission from Sebag [5], p. 266)
VMA and VMT influence the macula via different mechanisms. In the case of VMA, effects are molecular and physiologic, while untoward effects in VMT result from traction. Traction arising from anomalous posterior vitreous detachment (PVD) with persistent VMA between the posterior vitreous cortex and the inner limiting membrane (ILM) of the retina can be either tangential to the surface of the retina, axial, or both.
This chapter will briefly review vitreous biochemistry, structure, and physiology, as well as aging, PVD, and anomalous PVD causing VMT. The role of VMA as a comorbidity in exudative age-related macular degeneration and diabetic macular edema will be discussed. Lastly, the medical management of vitreo-maculopathies will be described, while surgical management will be covered elsewhere and only briefly addressed herein.
Vitreous Biochemistry and Structure
The main component of vitreous is water, which constitutes more than 98 % of the vitreous body. Roughly 15–20 % of this water is attached to glycosaminoglycans (GAGs), primarily hyaluronan. The other major structural macromolecule of vitreous is collagen. Variations in the concentrations of hyaluronan and collagen in different species account for differences in the rheologic (gel/liquid) state of the vitreous body [5, 6].
The three main GAGs of vitreous are hyaluronan, chondroitin sulfate, and heparan sulfate:
Hyaluronan (HA) is the major CAG of the human vitreous. HA synthesis begins after birth and plateaus in adulthood. As there is no extracellular degradation [7–10], the levels of HA tend to remain constant because HA molecules escape via the anterior segment of the eye [11]. It has previously been shown that HA acts like an ion-exchange resin in that an electrostatic interaction can occur between small charges of mobile ions in the tissue and the electrostatic envelope of this stationary polyelectrolyte [12]. This interaction explains the properties of HA in vitreous and its influences on ion transport and distribution, osmotic pressure, and electric potentials within the vitreous body.
Chondroitin sulfate (CS) is an important component of the extracellular matrix throughout the body. In vitreous, CS may serve as a link between HA and collagen. Versican, a vitreous CS, has been shown to house the pathogenic mutation in Wagner’s Vitreo-Retinal Dystrophy [13, 14].
Heparan sulfate (HS) is a renewable proteoglycan that has been found in small amounts in human vitreous. Its function is speculated to be the maintenance of an adequate distance between vitreous collagen fibrils to achieve transparency [15].
Type II collagen comprises 75 % of all vitreous collagens [16]. Stickler syndrome is a mutation that occurs in exon 2 of COL2A1, resulting in liquefied vitreous [16, 17] and an increased risk of retinal detachment. Type IX collagen comprises 10–15 % of vitreous collagen and interacts with collagen II fibrils and other collagens. It has been hypothesized that changes in the quantity and the location of type IX collagen are the reasons for many of the important phenomena in age-related vitreous changes [18, 19]. Lastly, a hybrid collagen type V/XI makes up about 10 % of all vitreous collagen [20]. Types II and V/XI often combine to form a heterotypic fibril that helps keep the distance between vitreous collagen fibrils to minimize light scattering [20].
Vitreous body structure consists primarily of interpenetrating networks of HA molecules and collagen fibrils. Collagen fibrils provide a rigid structure that is “filled” by the hydrophilic HA creating a solid gel in youth (Fig. 2). If collagen is removed from the vitreous body, HA forms a viscous solution, while removal of HA causes the gel to shrink [12]. Type II collagen is very rich in proteoglycans and has a strong collagen-proteoglycan interaction. It has also been shown that proteoglycans have a stabilizing effect upon collagen [21]. Swann et al. [22] postulated that HA-collagen interaction in the human vitreous might involve a third molecule that represents a “link” composed of either a glycoprotein or proteoglycans. This is consistent with previous studies that have shown HA to interact with link proteins [23], as well as an HA-binding glycoprotein, known as hyaluronectin [24]. Such organization to keep the vitreous collagen fibrils a critical distance apart minimizes light scattering [24] achieving transparency.


Fig. 2
Vitreous body of an eye obtained at autopsy from a 9-month-old child. The sclera choroid and retina were dissected off the vitreous body, which remains attached to the anterior segment. A band of gray tissue can be seen posterior to the ora serrata. This is the neural retina that was firmly adherent to the vitreous base and could not be dissected. Due to the young age of the donor, the vitreous body is almost entirely gel. Thus, it is solid and maintains its shape, although situated on a surgical towel exposed to room air (Reprinted with permission from Sebag [5], cover photo)
The vitreo-retinal interface is composed of the inner limiting membrane of the retina, the outer layer of the vitreous body, known as the posterior vitreous cortex (Fig. 1), and an intervening extracellular matrix containing laminin, fibronectin, and other constituents [25]. Opticin is a structural protein found at the vitreo-retinal interface, where it is speculated to play an important role in vitreo-retinal adhesion and the prevention of neovascularization [26, 27].
Aging and PVD
The vitreous body undergoes dramatic changes with age (Fig. 3) likely related to changes in collagen/proteoglycans composition and organization. In nondiabetic patients, the average half-life of vitreous collagen is estimated to be 15 years, similar to the skin [28]. In aging and especially in diabetes, vitreous pentosidine [25] and AGEs increase significantly [29, 30]. HA and CS, which are both attached to core proteins to form proteoglycans [20], are believed to play important roles in the structural changes with aging [30]. CS primarily interacts with type IX collagen and may be important in collagen aggregation [19, 31], while HS seems to play a role at the vitreo-retinal interface [20].


Fig. 3
Aging changes in human vitreous structure. Dark-field slit microscopy of fresh unfixed whole human vitreous body with the sclera, choroid, and retina dissected off the vitreous body, which remains attached to the anterior segment. A slit lamp beam illuminates from the side, creating a horizontal optical section with an illumination– observation angle of 90°, maximizing the Tyndall effect. The anterior segment is below and the posterior pole is above in all specimens. Top Panel: the vitreous bodies of an 11-year-old girl (left) and a 14-year-old boy (right) demonstrate a homogeneous structure with no significant light scattering within the vitreous body, only at the periphery where the vitreous cortex is comprised of a dense matrix of collagen fibrils. The posterior aspect of the lens is visible at the bottom of each image. Middle Panel: vitreous structure in a 56-year-old (left) and a 59-year-old (right) subject features macroscopic fi bers in the central vitreous body with an antero-posterior orientation. These form when hyaluronan molecules no longer separate collagen fibrils, allowing cross-linking and aggregation of collagen fibrils into visible fibers. Bottom Panel: in old age the fibers of the central vitreous become significantly thickened and tortuous, as demonstrated in the two eyes of an 88-year-old woman. Adjacent to these large fibers are areas of liquid vitreous, at times forming pockets, called lacunae. (Reprinted from Sebag et al. [224]).
After age 40 there is a significant decrease in gel volume and a concurrent increase in liquid vitreous, which mainly accumulates centrally [32, 33]. These changes within the vitreous body lead to pockets of liquid vitreous known as “lacunae.” Oksala [34] used ultrasonography to detect echoes from the gel/liquid interfaces in the aging vitreous body. Recent studies with swept source OCT imaging [35] have challenged the previous belief that the posterior lacuna is solely a manifestation of age-related liquefaction [35, 36]. Rather, this seems to represent an anatomic structure, most likely the bursa premacularis of Worst, since it is found in youth [37]. Nonetheless, with aging there is increasing liquefaction with additional lacuna formation, leading to destabilization of the vitreous body and ultimately collapse due to the currents and countercurrents of liquid vitreous occurring during head/eye movement. Posterior vitreous detachment (PVD) occurs when there is sufficient liquefaction to promote collapse of the vitreous body accompanied by sufficient weakening of vitreo-retinal adhesion to allow anterior separation of the posterior vitreous cortex away from the ILM of the retina. With adequate weakening of vitreo-retinal adhesion, PVD occurs without pathologic consequences [5, 38–40].
The most common cause of PVD is aging; however, myopia, diabetes, and several other conditions (Marfan syndrome, Ehlers-Danlos syndrome, Stickler syndrome [41]) are also predisposing factors. It has been shown that surgical removal of the crystalline lens results in a higher incidence of complete PVD [42]. Another study demonstrated the importance of posterior capsule integrity [43]. In a recent prospective study of 575 eyes without PVD prior to cataract surgery, 5 %, 8 %, 11 %, 18 %, and 30 % developed PVD at 3, 6, 12, 24, and 36 months postoperatively, respectively [44]. This is perhaps due to a reduction in the concentration of hyaluronan [45] resulting from increased diffusion into the anterior chamber, amplified by absence of an intact posterior capsule [45, 46]. As a result of HA loss, vitreous viscoelasticity decreases lowering shock-absorbing ability [47], resulting in increased force transmission to the remaining vitreo-retinal attachments during ocular saccades and head movement. Persistent vitreo-retinal adhesion during PVD can have serious untoward consequences.
Anomalous PVD
When gel liquefaction exceeds the degree of weakening in vitreo-retinal adherence, traction is exerted at the vitreo-retinal interface with a variety of potential ill effects, collectively known as anomalous PVD (APVD). Full-thickness APVD occurs when the entire (full-thickness) posterior vitreous cortex stays attached to the retina, such as in vitreo-macular traction syndrome or retinal tears/detachment. Partial-thickness APVD occurs when there is a split in the posterior vitreous cortex, called vitreoschisis (VS; Fig. 4). Figure 5 outlines the various possible consequences of APVD that vary depending upon the area(s) of greatest vitreous liquefaction and where the posterior vitreous cortex is most firmly adherent to the retina. While peripheral and optic disk APVD are important, this chapter will only consider vitreo-maculopathies. These will be discussed as either “primary,” where APVD is the cause, or “comorbid,” where vitreo-macular adhesion contributes to the underlying maculopathy, typically either exudative AMD or ME due to diabetes or retinal vein occlusions.



Fig. 4
Vitreoschisis. In vivo SD-OCT imaging of the human vitreo-retinal interface demonstrates a split in the posterior vitreous cortex, called vitreoschisis (Image courtesy of Jay Duker, MD)

Fig. 5
Mechanisms and clinical manifestations of anomalous PVD. The mechanisms and various clinical consequences of anomalous PVD are shown. The different manifestations depend upon the area(s) of greatest vitreous gel liquefaction and where the posterior vitreous cortex is most firmly adherent to the retina
Primary Vitreo-Maculopathies (APVD Is the Primary Cause)
Vitreo-Macular Traction (VMT)
VMT is defined as vitreo-macular adhesion with structural changes in the underlying macula [2]. At the International Vitreomacular Traction Study (IVTS) group conference in 2012, a new classification system of vitreo-macular traction was developed, based solely upon anatomic changes as found with OCT imaging. To diagnose VMT, the following changes should be observed: (1) evidence of perifoveal vitreous cortex detachment from the retinal surface, (2) macular attachment of the posterior vitreous cortex within a 3-mm radius of the fovea, and (3) distortion of the foveal surface, intraretinal structural changes, elevation of the fovea above the RPE, or a combination of these anatomical changes [2]. VMT is classified by the size of vitreous attachment: focal, if less than 1500 μm, or broad, if greater than 1500 μm.
In the classic form of VMT, the posterior vitreous cortex is separated from the retina in the peripheral fundus but remains attached posteriorly, resulting in antero-posterior traction on a broad, often dumbbell-shaped region encompassing the macular area and optic nerve [49–52] (Fig. 6). The zone of vitreo-macular attachment can be small as seen in Fig. 6 or measure several disk areas in size [51, 52]. Associated findings include a premacular membrane and varying degrees of macular edema with fluorescein leakage. If VMT is severe, traction macular detachment can result, as seen in Fig. 6. Symptoms tend to progress with time, but a full-thickness macular hole rarely develops [53]. Vitreo-retinal surgery to relieve VMT is often associated with anatomic and visual improvement [50, 51, 54–57]. It has been suggested that traction on the fovea arising from APVD is a contributing pathogenic factor in some cases of CME following cataract surgery [58].


Fig. 6
Anomalous PVD with vitreo-macular traction. Persistent vitreo-macular adhesion results in axial vitreo-macular traction with elevation of the central macula
Macular Holes (MH)
A full-thickness macular hole (FTMH) is defined as a foveal lesion with interruption of all retinal layers from the ILM to the RPE, usually diagnosed by OCT imaging. The exact pathophysiologic mechanism is unknown; however, many theories have been proposed, such as vitreous traction, trauma, foveal degeneration, high myopia, and involutional thinning with PVD. The IVTS differentiated FTMHs based on size (small ≤250 μm, medium >250 to <400 μm, large ≥400 μm), vitreous status (with or without VMA), and associated conditions (primary vs. secondary) [41]. This classification has been shown to be useful as it predicts the anatomic and functional success after treatment with pharmacologic vitreolysis or surgical intervention [2].
There is typically no macular edema in MH because the cysts surrounding the hole are tractional rather than exudative [59–62]. The tangential traction theory [63] suggests that shrinkage of the perifoveal vitreous cortex induces an FTMH in four stages [64]. The possible mechanisms that can cause tangential vitreous traction are: fluid vitreous movement and countercurrents, cellular remodeling of the cortical vitreous, and contraction of a cellular membrane on the tapered cortical vitreous after vitreoschisis [65–67]. Indeed, vitreoschisis has been found in half of the eyes with MH [67]. In addition, vitreo-papillary adhesion (VPA) may play a crucial role in the development of MH, since VPA is present in 88.2 % of MH eyes [68]. VPA is also more prevalent in eyes with intraretinal cystoid spaces in both lamellar macular holes and macular pucker [68, 69]. Thus, it has been suggested that VPA influences the vector of tangential forces on the macula causing outward (centrifugal) traction opening a central dehiscence in an FTMH, foveal splitting in lamellar MH, and the formation of intraretinal cysts. In contrast, macular puckers have a very low incidence of VPA, and the vector of tangential forces is believed to be inward (centripetal).
Macular Pucker (MP)
When anomalous PVD is associated with vitreoschisis, a vitreous membrane remains on the macular surface. The term epiretinal membrane (ERM) is often used in literature to refer to this membrane. However, this term is inaccurate for two reasons: The term “epi-” indicates a location next to or beside a structure, in this case, the retina. Thus, the term “epiretinal” could refer to a subretinal location, which is clearly not the case. In addition, all of the related conditions are maculopathies, not retinopathies. Therefore, the accurate term is “premacular membrane” (PMM), which will be used throughout this chapter. In addition, the term “idiopathic” ERM is no longer appropriate, as we know that the cause of this condition is vitreous.
Following APVD with vitreoschisis, premacular membranes (PMM) can contract and cause metamorphopsia and visual impairment. Light microscopic studies of these membranes have shown the presence of astrocytes and RPE cells. However, there are likely other cells that have a similar morphological appearance, namely, hyalocytes. Zhao et al. examined ILM from 79 patients with macular pucker or VMT and found that hyalocytes are one of the major cell types [70]. These cells can elicit the migration of monocytes from the circulation further increasing the population of mononuclear phagocytes in these membranes. Sebag hypothesized that MP results when VS splits the posterior vitreous cortex anterior to the hyalocytes, leaving a thick and cellular membrane attached to the macula. A recent study found that half of all eyes with MP have more than one site of retinal contraction leading to a higher incidence of intraretinal cysts and significantly more macular thickening [71]. It is not clear whether these cysts are the result of traction as in the case of MH, or exudation and macular edema, which is found in about 40 % of eyes with MP [72].
Premacular membranes (PMM) are associated with macular dysfunction most likely due to an impairment of the neural retinal layers, particularly disruption of the inner and outer segments of photoreceptors [73, 74]. With time, PMM contraction in MP can result in disruption of the blood-retinal barrier in the underlying retina with exudation, identifiable on fluorescein angiography (Fig. 7). The extracellular accumulation of fluid can lead to cystoid changes, particularly in chronic MP. Thus, in addition to the distortions induced by the PMM in MP, ME causes blurred vision subjectively and decreased visual acuity objectively [75].


Fig. 7
Macular pucker. (a) Color fundus photograph of the left eye in a 65-year-old man showing macular pucker. (b) Premacular membrane (PMM) contraction in macular pucker has resulted in the disruption of the blood-retinal barrier with leakage and macular edema as demonstrated on fluorescein angiography
Vitreous surgery is the definitive cure for MP, with reduction/elimination of distortions [76], resolution of ME, and restoration of normal macular thickness and function. Visual acuity improves in 87 % of cases with restoration of normal macular thickness in 94 % of patients [77, 78]. MP does not presently appear to be treatable by pharmacologic vitreolysis, as the presence of a PMM is a relative contraindication to this form of therapy for vitreo-maculopathies (see below).
Comorbid Vitreo-Maculopathies (Vitreous Contributes)
Vitreous in Exudative AMD
It has been shown that full-thickness vitreo-macular adhesion (VMA) and traction (VMT) play a role in exudative age-related macular degeneration (AMD). Anomalous PVD with persistent VMA is believed to promote or at least predispose to choroidal neovascularization, while a total PVD appears to be somewhat protective against wet AMD [79–82]. Clinical studies [79] found a twofold higher prevalence of PVD (diagnosed by ultrasound) in eyes with dry AMD compared to exudative AMD and a fivefold higher prevalence of VMA (diagnosed by OCT) in wet AMD compared to eyes with dry AMD. This was subsequently confirmed in two separate paired eye studies [79, 83] and was recently confirmed by a meta-analysis of 1,025 qualified articles, which found that wet AMD has double the prevalence of VMA and is less likely to have PVD [84]. Thus, although the exact mechanism is yet to be discovered, anomalous PVD seems to be a risk factor for exudative AMD [85–87]. A recent study [88] looked at 30 eyes of 25 patients with VMA surrounded by shallow detachment of the posterior vitreous cortex. The area of adhesion corresponded to a site of retinal angiomatous proliferation (RAP) in 88.2 %. In 73.3 % there was evidence of visible vitreo-retinal traction lines directed toward the choroidal neovascular membrane. Additionally, vitreo-papillary adhesion could be detected on the temporal margin of the optic disk in 83.3 %, about as often as in macular holes [68, 69], and the posterior vitreous cortex seemed to be split in the area of vitreo-papillary attachment, constituting vitreoschisis.
Vitreous in Diabetic Macular Edema (DME)
The biochemistry [30] and structure [89] of vitreous is altered by diabetes resulting in diabetic vitreopathy [48], which plays a role in the pathobiology of proliferative diabetic vitreo-retinopathy. Ultrasonography [90] and histopathology [91] have demonstrated vitreoschisis in proliferative diabetic retinopathy as well as in DME [92], which is the most common cause of vision loss in diabetic patients. In DME, cytokines and chemokines such as VEGF, fibroblast growth factor-2, and protein kinase C induce proliferation of hyalocytes and astrocytes in the posterior vitreous cortex [93]. These cells may strengthen adhesion of the posterior vitreous cortex to the ILM and perpetuate VMA, promoting VMT and contributing to DME [93]. Clinical studies have shown that the surgical removal of the posterior vitreous in DME patients contributes to less ischemia and decreased vasopermeability [94–96]. These findings may not only be due to relief of VMT by vitrectomy but also the removal of various molecules that promote DME, since vitreous appears to be a repository for various molecules that promote DME [97, 98]. Recent studies have shown that diabetic patients have more pro-inflammatory molecules in the vitreous body compared to nondiabetics [99]. In particular, IL-1B, caspase 1, and chemokines are increased significantly in vitreous, suggesting that chronic low-grade inflammation may be a factor in the pathogenesis of DME [99]. TNFα, which is also found in vitreous of patients with DME, is known to increase retinal endothelial permeability [100]. Other key molecules thought to promote DME by breakdown of the blood-retinal barrier are endothelial adhesion molecules, such as ICAM1, VCAM1, platelet-endothelial cell adhesion molecule 1 (PECAM-1), and P-selectin [101, 102].
Vitreous in Macular Edema due to Retinal Vein Occlusion (RVO)
Globally, an estimated 13.9 million people are affected by branch retinal vein occlusion (BRVO) and 2.5 million by central retinal vein occlusion (CRVO) [103], affecting males and females equally with a mean age of 65 years for CRVO. Five percent of RVO occurs in people over 80 years of age [104, 105]. The primary risk factor for RVO is atherosclerosis [105, 106], although arterial hypertension, cardiovascular disease, hyperlipidemia, diabetes mellitus, hypercoagulation, thrombophilia, and inflammatory disease have also been associated with RVO.
Retinal vein thrombosis causes elevation of intravascular pressure in vessels distal to the occlusion. The increased transmural hydrostatic pressure gradient in retinal capillaries results in transudation of fluid into the extracellular space. Associated hypoxia releases cytokines such as VEGF that break down the blood-retinal barrier, causing further leakage in both central and branch RVOs [107–110]. Although the exact etiology of RVO is not understood, vitreous seems to play a role [111]. Vitreo-papillary adhesion has been documented in patients with ischemic central retinal vein occlusion (CRVO), resulting in a secondary serous neurosensory detachment that usually involves the macula [112]. Thick peripapillary tissue may be due to the growth of fibrous tissue in the area of VPA following hemorrhagic CRVO.
Although the current mainstay in treating ME due to RVO is intravitreal anti-VEGF injection [113], vitrectomy has been shown to be relatively beneficial for non-ischemic CRVO [114–116] and BRVO [116–119]. In addition, pharmacologic vitreolysis with autologous plasmin enzyme in eyes with BRVO has resulted in PVD and significant reduction of ME and improvement of VA in 88 % of eyes [119]. These observations suggest a role for the cortical vitreous and PVD status in BRVO. Future studies should explore whether pharmacologic vitreolysis could provide a useful adjunct to other forms of therapy for ME due to RVO.
Therapy
Surgical
The surgical management of vitreo-maculopathies is covered elsewhere in this book. However, two aspects that deserve emphasis here are chromodissection and reoperations.
Chromodissection
Chromodissection is a term that refers to the use of dyes to stain the ILM so as to facilitate membrane peeling [120]. This technique is especially useful in macular hole surgery [121], achieving a primary anatomic closure rate of 94 % using indocyanine green (ICG) chromodissection and 89 % with trypan blue [122].
ILM removal by chromodissection remains a controversial topic in macular pucker (MP) surgery. The rationale for ILM removal relates to the high incidence of vitreoschisis in MP and the risk of leaving a layer behind if ILM removal is not performed. Further, removal of ILM may reduce the incidence of MP recurrence due to removal of a scaffold that would enable cells to re-proliferate.
Reoperations of Macular Holes (MH) and Macular Pucker (MP)
Failure of MH surgery results from the incomplete peeling of the posterior vitreous cortex, the presence of subclinical premacular membrane (PMM) causing a residual traction at the hole, or inadequate gliosis [123, 124]. After initial successful closure, there can also be reopening of an MH, sometimes even years later [122, 123, 125–128]. The presence and progression of PMM formation has been correlated with MH recurrence, the mechanism thought to be that the PMM exerts tangential traction causing foveal dehiscence [123, 124].
Macular edema (ME) is associated with a sevenfold increase in the reopening of MH [129], with inflammatory fibrinolysis considered to be the causative process [122, 128]. Post-vitrectomy cataracts occur in 76 % of cases after 2 years [130–134], and cataract surgery after MH surgery has been associated with the reopening of the hole within 6 months of cataract extraction [122, 125, 128]. The proposed hypothesis for this event is the development of ME and/or PMM formation after cataract surgery due to breakdown of the blood-retinal barrier introducing postoperative inflammatory mediators. In order to avoid these complications, some surgeons have elected to combine MH surgery with phacoemulsification [130–132]. Lastly, YAG capsulotomy of posterior capsular opacification has been implicated in MH reformation [133], with the mechanism thought to be a perifoveal vitreous contraction related to the laser pulse, although it is not clear how this could occur.
Reoperation for recurrent/persistent MH and MP is successful if the cause is incomplete removal of the PMM. In this instance, ILM peeling with chromodissection can be performed with a number of stains such as ICG, trypan blue, triamcinolone acetonide, and Brilliant Blue G [67, 135–138]. In cases where adequate PMM and ILM peeling have been performed in the central macula, it has been suggested that more extensive ILM removal toward the vascular arcades may be an option [139]. However, reoperations can at times be associated with profound loss of vision [140]. Patients usually describe the sudden onset of a scotoma that occurs soon after surgery. There is significant loss of visual acuity, an afferent pupillary defect (APD), and altitudinal visual field loss. This presentation has been identified as resulting from vitrectomy with membrane peeling that has injured the retinal nerve fiber layer, resulting in an inner retinal optic neuropathy (IRON) [141]. Unlike AION, the visual field loss in IRON is not altitudinal, and no disk edema is present [141]. Studies [141] have determined that the risk of IRON is markedly diminished if reoperations are undertaken at least 6 months after the initial surgery. This is presumably due to the protection of the inner retina provided by resynthesis of the ILM, a process that takes many months [142].
Medical Management
Macular Edema (ME) in Retinal Vein Occlusion (RVO)
The current mainstay of treating ME from RVO is VEGF inhibition with ranibizumab, bevacizumab, or aflibercept. Frequent injections are usually needed to show benefit [143, 144], and rebound edema is sometimes worse than pretreatment edema [145]. In addition, the possible neurotoxic effect of chronic pan-VEGF suppression in an ischemic retina has raised concerns [146]. Other therapies have been advocated such as laser-induced chorio-retinal anastomosis, retinal intravascular tPA infusion, and surgery by radial optic neurotomy or arteriovenous sheathotomy [147]. Considering the aforementioned putative role of vitreous in RVO, there may be value in performing only vitrectomy with surgical induction of PVD or pharmacologic vitreolysis to induce PVD as an adjunct to the medical management of ME from RVO.
Diabetic Macular Edema (DME)
DME is the leading cause of vision loss in patients with both types 1 and 2 diabetes. Corticosteroids have been used to suppress inflammatory cell proliferation and migration and block the upregulation and/or activity of many pro-inflammatory cytokines involved in leukocyte stasis and breakdown of the blood-retinal barrier [148], but there are complications that follow repeated steroid injections. VEGF inhibition is an FDA-approved pharmacologic approach for the management of DME. Studies using pegaptanib [149], ranibizumab [150, 151], and bevacizumab [152] have demonstrated favorable clinical responses. It has recently been shown that aflibercept is superior to ranibizumab, which is in turn superior to bevacizumab in the treatment of DME [153].
Vitreous seems to play an important role in DME, both via VMA (see above) and VMT [154, 155]. There is also evidence that even subclinical perifoveal vitreous detachment may play a role in DME [156]. Thus, it would be reasonable to consider that the induction of PVD by pharmacologic vitreolysis could provide a useful adjunct to the medical therapy of DME.
Macular Edema in Age-Related Macular Degeneration (AMD)
Leakage from choroidal neovascularization (CNV) in neovascular age-related macular degeneration (AMD) results in intraretinal, as well as subretinal, fluid accumulation [157–159]. Studies have found that in exudative AMD, decreased visual acuity correlates with increased macular thickness [159, 160]. Anti-VEGF treatment with intravitreous ranibizumab has resulted in significant reduction of central retinal thickness (which includes both intraretinal and subretinal fluid components) as early as 1 day after the first injection [160]. Clinical trials have shown a significant reduction in central retinal thickness and improvement in visual acuity with anti-VEGF therapy [160]. It is important to note that reduction of macular edema overlying the CNV is not always accompanied by visual gain, most likely due to the atrophy of photoreceptors, loss of RPE, fibrotic scarring, and other irreversible alterations.
The importance of vitreous in the pathophysiology of exudative AMD was described above. Vitreous also appears to play a role in the therapy of exudative AMD [161]. In eyes with VMA, the attached posterior vitreous cortex might create a barrier effect interfering with the influx of nutrients and oxygen to, as well as the efflux of pro-angiogenic cytokines from, the macula [84]. A study in 110 eyes with wet AMD but no VMA found that there was significant improvement in VA after treatment with anti-VEGF injections (P < 0.05) [162], while eyes with VMA did not have a significant improvement. This was confirmed by another study [163] of 255 treatment-naïve subjects, also finding that eyes with PVD improved with fewer injections. Thus, inducing PVD may not only eliminate any contribution of vitreous to the pathophysiology of exudative AMD but may be a way to enhance the pharmacotherapy of AMD as well. This was recently achieved via pharmacologic vitreolysis using ocriplasmin in 100 patients with exudative AMD and VMA. Those patients who achieved VMA resolution required fewer anti-VEGF injections [164]. These findings were recently confirmed by a study of p.r.n. dosing with ranibizumab in 34 treatment-naïve eyes with VMT, compared to 29 treatment-naïve eyes without VMT, as well as to other variable dosing studies (CATT, HARBOR, PrONTO, SUSTAIN). The findings showed that after 1 year of treatment, eyes with VMT had no significant improvement in visual acuity or central macular thickness, while eyes without VMT had significant improvements in both, and the degree of improvement was comparable to those of previous large studies [165].
Given the apparent role of vitreous in both the pathophysiology [166] and therapy [84] of exudative AMD, there may be benefit in eliminating the contribution of vitreous through adjunctive therapy to separate vitreous from the macula. While this can be effectively achieved with vitrectomy, a less invasive approach is preferable, such as with pharmacologic vitreolysis.
Pharmacologic Vitreolysis
Vitrectomy surgery is the mainstay of therapy to eliminate the role of vitreous in various retinal diseases, but it is considerably invasive, expensive, and can lead to complications [141, 167, 168]. Thus, alternative methods for the induction of PVD have been investigated, in particular, pharmacologic vitreolysis [169]. To this end, various agents have been tested over the past 20 years. According to their mechanism of action, these agents can be categorized as “enzymatic” or “nonezymatic.” Enzymatic agents include tissue plasminogen activator (tPA) [170], plasmin [171], microplasmin [172], nattokinase [173], chondroitinase [174], dispase [175], and hyaluronidase [176]. Nonenzymatic agents include urea/Vitreosolve and arginine-glycine-aspartate peptides [177].
Recently, Sebag [177] proposed a classification system in which pharmacologic vitreolysis agents are categorized based on their biologic effect: those that induce vitreous liquefaction (liquefactants) and those that induce dehiscence at the vitreo-retinal interface (interfactants). Table 1 classifies agents based on these biologic activities. Of note is that there have been seven agents tested to date. Five projects have failed or been disbanded, one agent is currently under continuing investigation, and only one agent has been approved for clinical pharmacologic vitreolysis.
Table 1
Pharmacologic vitreolysis classification based on biologic action
Liquefactants (agents that liquefy the gel vitreous) |
Nonspecific: |
tPA, plasmin, ocriplasmin, nattokinase, Vitreosolvea |
Substrate specific: |
Hyaluronidase |
Interfactants (agents that weaken/lyse vitreo-retinal adhesion) |
Nonspecific: |
tPA, plasmin, ocriplasmin, nattokinase, Vitreosolvea |
Substrate specific: |
Dispase, chondroitinase, RGD-peptidesa |
Tissue Plasminogen Activator (tPA)
tPA is a serine protease that converts plasminogen into plasmin, the main enzyme responsible for blot clot lysis [178]. A randomized study [179] compared an intravitreal injection of 25 μg tPA to balanced salt solution (BSS) in ten patients before vitrectomy for proliferative diabetic vitreo-retinopathy. The use of tPA purportedly resulted in disintegration of the vitreo-retinal interface by PVD, thus facilitating vitrectomy without severe side effects [179]. In early studies the use of intravitreal tPA injection to treat subretinal hemorrhage encountered complications such as vitreous hemorrhage related to the fibrinolytic activity of tPA, which has been a concern [180, 181]. This is not frequent, however, and intraocular tPA is used routinely to lyse and displace submacular hemorrhage [182].
Plasmin
This nonspecific protease plays an active role in biologic processes such as fibrinolysis, neovascularization, and the activation of enzymes such as matrix metalloproteinases [183, 184]. Plasmin acts directly on fibronectin and laminin, which are in part responsible for adhesion between the posterior vitreous cortex and the ILM [171, 185]. Plasmin has been used to induce PVD in several animal models [171, 183, 185–189]. Intravitreal plasmin injection before or during vitrectomy has been studied in case series for DME with an adherent thick posterior vitreous cortex [190], macular holes [191, 192], proliferative diabetic retinopathy [193], diabetic tractional retinal detachment [194], VMT syndromes [195], and retinopathy of prematurity [196, 197]. Studies evaluating the use of plasmin during macular hole surgery suggest that plasmin facilitates membrane peeling [191, 192, 198, 199], especially in pediatric macular holes [197]. Other studies evaluated the use of plasmin during vitrectomy in six patients with bilateral proliferative diabetic retinopathy [193]. Surgical time was reduced (68 vs 89 min, p = 0.04) and the incidence of retinal tears (0 vs. 3 eyes) was lower in the plasmin group than untreated fellow eyes, although there were no significant differences in vision outcomes [193]. Tsukhara et al. [196] successfully employed plasmin in six eyes with stage five ROP, four with a closed-funnel retinal detachment.
The intraoperative use of plasmin in eyes with DME associated with posterior vitreous cortex contraction found no significant difference in final retinal thickness, but plasmin-treated eyes had significantly better visual acuity than controls [190]. Another study evaluated the use of intravitreal plasmin as an adjunct to vitrectomy for 16 patients with refractory (to laser) DME [200]. One month post injection, central macular thickness was reduced and visual acuity was significantly increased in the plasmin-treated eyes [200]. The investigators concluded that pharmacologic vitreolysis effectively reduces central macular thickness and improves visual acuity in DME patients refractory to conventional laser treatment. However, because plasmin is derived preoperatively from the patient’s blood by affinity chromatography, followed by a complicated process of sterilization and refrigeration before use [201], this technique is time consuming, cumbersome, and difficult. Further, plasmin is not commercially available for clinical use, and the enzyme activity is dependent on several variables. Thus, plasmin has not gained widespread use [202].
Ocriplasmin
The development of recombinant ocriplasmin has solved many of the problems with plasmin. Ocriplasmin contains only the enzymatic portion of the plasmin molecule [203] and is thus smaller (28 vs. 80 KD) [204]. Research with experimental models demonstrated that in both feline and human cadaver eyes there is dose- and time-dependent cleavage between the posterior vitreous cortex and the ILM without adverse effects on retinal structure [205]. Gandorfer et al. found that doses of 125 μg (the current clinical dose) or more caused a complete PVD with bare ILM in human eyes as demonstrated by electron microscopy. These findings were confirmed by DeSmet et al. [206] in porcine eyes. Their findings were corroborated by Sakuma et al. [172] in rabbit eyes using doses of ocriplasmin from 12.5 to 250 μg; although doses of 125 μg of ocriplasmin or greater induced a complete PVD, lower doses induced a partial PVD. Recently, Chen et al. [203] used immunofluorescent histochemistry in rat eyes to determine that ocriplasmin causes degradation of fibronectin and laminin not only at the vitreo-retinal interface but also at the photoreceptor layer in the outer retina, a potentially untoward effect.
Clinically, ocriplasmin has been found to induce PVD and vitreous liquefaction without evidence of retinal toxicity [172]. In addition to salubrious mechanical effects, there may be physiologic benefits to ocriplasmin pharmacologic vitreolysis. Sebag et al. [207] reported that pharmacologic vitreolysis with ocriplasmin increases vitreous diffusion coefficients as determined by dynamic light scattering. Another study [208] showed that PVD induction by ocriplasmin increases oxygen levels in the vitreous body, perhaps assisting in the management of ischemic retinopathies with exudative maculopathy like DME and ME from RVOs (see above).
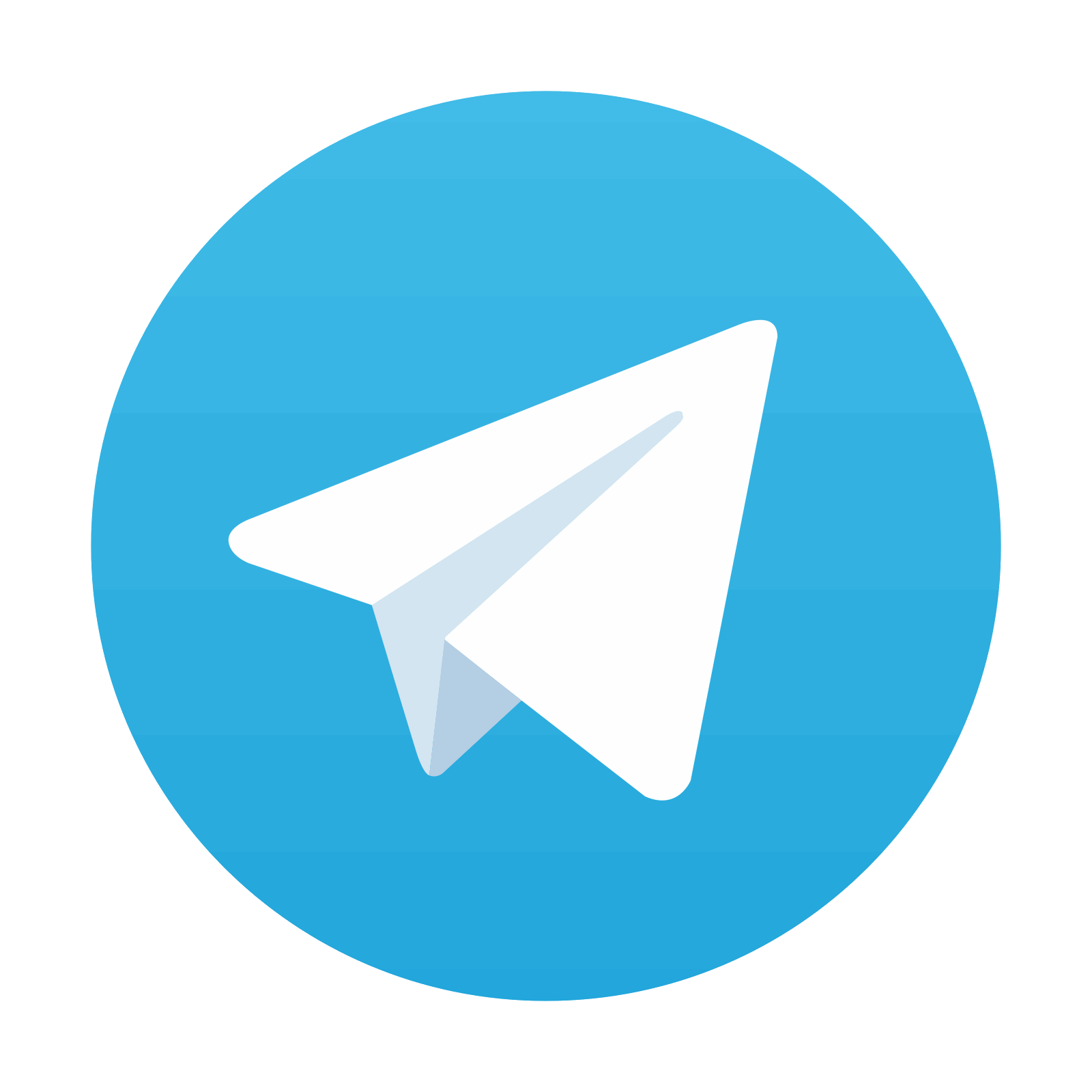
Stay updated, free articles. Join our Telegram channel

Full access? Get Clinical Tree
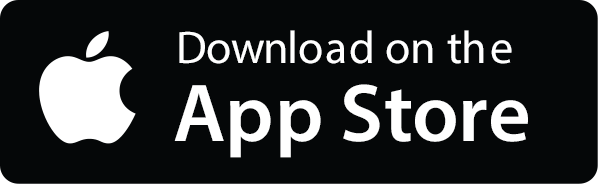
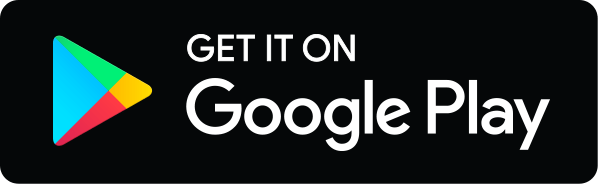
