Fig. 5.1
Family 1: two affected daughters, (a–d) mother (e, f), and their 64-year-old mother. (a) Fundus color the left eye (LE) of 36-year-old female: juxtapapillary drusen and some drusen around the fovea. Her visual acuity was 0.8; she was myopic (−10 D). Six years earlier, only the juxtapapillary drusen were visible. (b) Fundus autofluorescence (FAF) image LE of same patient: some macular and juxtapapillary drusen were hyperautofluorescent. (c) Fundus color LE of her 39-year-old sister: juxta- and peripapillary drusen, as well as confluent drusen at posterior pole with hyperpigmented area fibrosis and area of atrophy. The visual acuity: 0.1. (d) FAF LE of the same patient: hypofluorescence corresponding to atrophy and hyperautofluorescent dots corresponding to drusen. (e) Fundus color LE of their 64-year-old mother: some juxtapapillary drusen, confluent peripapillary, and around macula drusen; central atrophy. Visual acuity: hand movements. (f) FAF LE: hypofluorescence corresponding to atrophy, hyperautofluorescence corresponding to confluent and isolated drusen

Fig. 5.2
Family 2: 79-year-old father (a, b) and his 49-year-old daughter (c, d). (a) Fundus color of the right eye (RE) of the father: central atrophy, optic nerve within normal limits, inferior of temporal artery scars post laser. Visual acuity: 0.1 corrected. (b) Fundus color of the left eye (LE) of the father: central atrophy, small hemorrhage at the superotemporal edge. Visual acuity: 0.16 corrected. (c) Fundus color of RE of the daughter: confluent large macular drusen, radial small drusen, and areas of hyperpigmentation. Visual acuity 1.0 corrected. (d) Fundus color of LE of the daughter: confluent large drusen and radial drusen at the macula, central atrophy, peripapillary drusen. Visual acuity 0.4 corrected

Fig. 5.3
Family 3: two affected daughters (30 and 32 years old) (a–d) and their 55-year-old mother (e, f). (a) Fundus color of the left eye (LE) of the 30-year-old affected daughter: juxtapapillary drusen and macular drusen, predominantly in the inferior part. Visual acuity 1.25. (b) Fundus autofluorescence (FAF) of the same patient: some macular drusen were hyperautofluorescent. (c) Fundus color of LE of the 32-year-old affected daughter: only nasal juxtapapillary drusen (arrow) are visible, absence of macular drusen. Visual acuity 1.25. (d) FAF of the same patient: absence of hyperautofluorescent areas at macula. (e) Fundus color of the 55-year-old affected mother: presence of drusen at the macula. Visual acuity 1.0. (f) FAF: some drusen were hyperautofluorescent

Fig. 5.4
Family 4: one patient (47 years old) from a large autosomal dominant pedigree. (a) Fundus color of the right eye: juxtapapillary drusen, some confluent drusen around fovea, central scar following neovascular membrane (previously treated with anti-VEGF), and small radial drusen. Visual acuity 0.9. (b) Fundus autofluorescence: hyperautofluorescence (corresponding to the drusen) and hypoautofluorescence (corresponding to the scar) around fovea. Hyperautofluorescent patches around optic nerve

Fig. 5.5
Family 5: one patient (25 years old) with a de novo heterozygote R345W mutation in the EFEMP1 gene. (a, b) Fundus color of the right eye (RE) and the left eye (LE): large, confluent drusen at posterior pole and nasal of the optic disc, small radial drusen. One juxtapapillary drusen RE, otherwise sparing (normal retina) around the optic discs. Visual acuity: 0.5 corrected, both eyes. (c–e) Fundus autofluorescence (FAF) images of RE and LE: hyperautofluorescence of drusen, area of hypofluorescence at the fovea in both eyes. (d–f) Spectral-domain optical coherence tomography of RE, LE: diffuse deposition of hyperreflective material underneath the retinal pigment epithelium (RPE) – diffuse, dome-shaped elevation of the RPE (large drusen). Hyperreflective sawtooth elevations (small drusen). Intact appearance of inner retina
The onset of symptoms is typically at age of 30–50 years; however, childhood cases with severe visual loss have also been reported [10]. Good visual acuities in the seventh decade of life and one case of non-penetrance in a 62-year-old asymptomatic and mutation-positive patient with normal fundus appearance have been reported [15, 16], illustrating the variability of disease phenotypic expressivity. Disease severity varies with evidence of interocular, intrafamilial (see Figs. 5.1 and 5.3), and interfamilial variability in visual loss and natural history, as reported in more recent reports of British, Japanese, Chinese, and Indian pedigrees [15, 17–19].
Early visual symptoms include reduced central vision, photophobia, slow dark adaptation, paracentral scotomas, and metamorphopsias [15, 20, 21]. Color vision loss is a later finding [15]. Symptomatic patients report gradual deterioration over many years. Mild cases are characterized by normal visual acuity and the presence of small, discrete drusen at the macula (see Fig. 5.3); in some cases, the macula is normal and only some drusen deposited at the optic disc margin are observed [15, 19]. In the later stages of the disease and in severe cases, typically at the age of 50 years, central vision deteriorates and absolute scotomas develop predominantly as result of retinal pigment epithelium (RPE) atrophy [10, 22]. Subretinal neovascular membrane can develop (see Fig. 5.4a) but is a very uncommon complication [15, 18, 19, 23], sometimes associated with a subretinal hemorrhage as the presenting symptom of the disease [24]. A neovascular membrane can lead to a severe vision loss in younger patients in their 30–40s [19, 23, 25, 26] and can be bilateral [19]. In some cases, hyperplasia of the RPE and subretinal fibrous metaplasia can occur [26, 27].
5.3 Paraclinic Testing and Their Use in the Diagnosis of Malattia Leventinese
Fundus autofluorescence (FAF) imaging allows the visualization of the RPE health by taking advantage of the fluorescent properties of lipofuscin [28]. The increased autofluorescence associated with drusen in monogenic macular dystrophies has been reported [29]. In recent report in patients with a confirmed fibulin-3 mutation status, all increased foci of autofluorescence correlated to drusen, but some drusen displayed reduced or absent autofluorescence. The autofluorescence was absent in areas of retinal atrophy, seen ophthalmoscopically, and reduced in some areas, suggesting widespread macular dysfunction [15]. Querques et al. [25] observed increased and intense autofluorescence only in large drusen, but not in small radial drusen. However, the main highlight of FAF imaging was to detect hyperfluorescent dots at the optic disc margin, confirming peripapillary drusen, which were not obvious on routine fundus examination [15, 19].
Time-domain and spectral-domain (SD) optical coherence tomography (OCT) have provided insight into structural retinal changes in patients with autosomal dominant drusen due to EFEMP1 mutation. The first reports using a time-domain OCT described a diffuse hyperreflective thickening of RPE–Bruch’s membrane complex, associated with dome-shaped elevations, corresponding to large drusen [30, 31]. A remarkable preservation of the neurosensory retina was observed, both in perimacular and foveal areas [31]. Later reports, using an SD OCT, provided a better understanding of the two types of drusen: large round drusen appeared as diffuse or focal deposition of hyperreflective material underneath the RPE – determining a diffuse RPE elevation or a focal dome-shaped elevation. The small drusen, described as located above the RPE, had a sawtooth elevation appearance [25, 32]. The preservation of intact inner retina and preserved inner/outer segment junction was noted over small drusen, while the inner/outer segment junction was disrupted over large drusen [25]. In addition some intraretinal cyst and retinal tubulation were noted [25, 33]. Similarities between small, radial drusen in ML patients and cuticular drusen (also known as basolaminar drusen) have been reported [34, 35].
Additional insight into morphological and functional differences between the two types of drusen is given by reports of fluorescein angiography (FA) and indocyanine green angiography (ICG) features in ML. On both FA and ICG, large round drusen are hypofluorescent in early phases and turn into hyperfluorescent in late phase, with a dark halo on late ICG frames. The small drusen hyperfluoresce in early frames and decrease their fluorescence toward the later phase [25, 36]. Therefore, while small drusen share similitudes with cuticular drusen [35], the large drusen behave at FA and ICG more like age-related macular degeneration (AMD) drusen with a late hyperfluorescence [37].
Full-field electroretinogram (ERG), electrooculogram, and multifocal ERG data are available. The full-field ERG showed a normal rod b-wave responses, normal standard combined (mixed rod plus cone) response, and reduced 30-Hz flicker responses in both eyes in a 42-year-old patient from the Japanese pedigree [18]. Similar findings were described by the British group [21], who found that the scotopic sensitivity was reduced and dark adaptation kinetics were prolonged over the macular deposits only, but were normal elsewhere in patients affected by ML. Those findings were in keeping with a hypothesis that ML is a focal disease. A Swiss group [20] described “low-normal” amplitudes of the b-wave of rod and cone response, suggesting discrete but widespread functional abnormalities, while the b-wave amplitudes were subnormal in more advanced cases.
5.4 Molecular Genetics: Mapping
Linkage studies in families ML and DHRD mapped the disease locus to chromosome 2p16–21 [13, 22, 38, 39]. The first investigation was performed on 5 families (a large American kindred with 2 extensively affected branches and 3 kindreds from the Leventinese valley) with the ML phenotype with a total of 56 affected patients. They demonstrated linkage of ML to chromosome 2p. The maximum two-point LOD score observed in all families combined was 10.5 and was obtained with the marker D2S378. To further narrow the genetic interval containing the ML/DHRD gene, 63 members from a large nine-generation DHRD British pedigree, originally by O’Neill [1] and Pearce [14], were assessed for molecular genetic linkage study [13]. Two-point analysis showed a significant linkage of the DHRD to nine markers on the short arm of chromosome 2, a region overlapping the one recently reported to be linked to ML. A maximum LOD score of 7.29 was obtained at marker locus D2S2251. Haplotype analysis of recombination events localized the disease to a 5 cM interval between D2S2316 and D2S378. These results established that DHRD maps to chromosome 2p16, a region included within the 14-kb region to which ML was recently mapped.
A combination of positional and candidate gene methods in 39 families from Switzerland, the United States, and Australia helped identifying a single nonconservative arginine 345 to tryptophan mutation (Arg345Trp) in the gene EFEMP1 (EGF-containing fibrillin-like extracellular matrix protein 1) coding for fibulin-3 in all families studied, inclusive of both phenotypes, ML and DHRD. The change was not present in 477 control individuals or in 494 patients with AMD [12]. In this original study, the authors found that among all families studied, there was a complete sharing of alleles of intragenic EFEMP1 polymorphisms (SNPs). The same haplotype, and the absence of de novo R345W mutation in different ethnicities, was highly suggestive that the R345W mutation occurred only once in a common ancestor of every affected patient [12]. This study also confirmed that ML and DHRD are the same disorder.
A few years later, a genetic study revealed a novel disease haplotype with R345W mutation in an Indian pedigree, suggesting an independent origin [17]. A novel, different haplotype with the R345W mutation was identified in a Japanese pedigree [18], suggesting that the disease might have occurred independently in a common Japanese founder. In addition to those three different haplotypes, our group recently found a de novo R345W mutation in a young Portuguese patient with ML (unpublished data) with proven paternity, confirming that the EFEMP1 R345W mutation is not a founder mutation, but more likely a hot spot mutation.
5.5 Histopathological Studies
Immunohistochemistry analysis, using monoclonal and polyclonal antibodies, of seven human donor eyes showed that, in normal retina, fibulin-3 is predominantly present in photoreceptor’s inner and outer segment region and the nerve fiber layer, but not in the RPE, Bruch’s membrane, or choroid [40]. In a donor eye from an 86-year-old ML patient carrying a homozygote R345W mutation, fibulin-3 was found to accumulate beneath the RPE, overlying drusen, and in Bruch’s membrane, in addition to its presence in the interphotoreceptor matrix and the nerve fiber layer. EFEMP1 accumulation was also detected within some RPE cells and a few drusen. The data suggested that EFEMP1 was not a major component of drusen associated with ML [40].
In situ hybridization of human retinas was performed to study the pattern and level of expression of EFEMP1 in ML donor eye and human control eyes [41]. An 80-year-old ML donor patient, heterozygote for R345W mutation in EFEMP1 gene, was available in that study. In normal eye, EFEMP1 transcripts were localized mainly in the retinal ganglion cells, and the photoreceptor’s outer segments showed very similar distribution to that of the ML eye. Even at the level of RPE, very similar patterning of EFEMP1 expression was observed between a normal donor and ML eye. Surprisingly, in the ML eye, there was a 20-fold difference in EFEMP1 expression in the ciliary body, as compared to the EFEMP1 expression in the RPE, while there was a complete lack of EFEMP1 expression in ciliary body of normal donor. No histological abnormality was detected in the ciliary body of the ML donor. The robust upregulation of EFEMP1 in the ciliary body is an ML-specific feature [42].
A recent morphologic and histochemical analysis of drusen in an ML donor and seven AMD donors showed that drusen from both diseases shared many major constituents. However, drusen from the ML donor had a unique onion-skin-like lamination and possessed collagen type IV, which was absent in age-related drusen [43].
5.6 Hypothesis on R345W Fibulin-3 Function
Human EFEMP1 cDNA encodes a putative protein of 387–493 amino acids, with a predicted molecular mass of 43–54.6 kDa. EFEMP1 gene consists of 12 exons and spans approximately 60 kb of genomic DNA [44]. There are five predicted splice variants, but only the shortest (43.1 kDa) and the longest (54.6 kDa) are expressed in substantial amounts at the protein level [45]. The EFEMP1 protein is a broadly expressed extracellular matrix molecule: in tissues from adult mice, EFEMP1 expression was abundant in the eye and lung and was moderate in the brain, heart, spleen, and kidney [12]. It is one of the seven members of the fibulin family of proteins. The protein sequence contains a signal peptide, five to six calcium-binding epidermal growth factor (cbEGF) domains, a predicted N-glycosylation site at Asn249, and a highly hydrophobic C-terminal fibulin-type domain [45, 46]. The protein is highly conserved among humans and rodents, but its homology is restricted to the EGF-like domains. The single Arg-Trp mutation alters the last calcium-binding EGF-like domain of EFEMP1 and is similar to the number of fibrillin mutations [47].
EFEMP1 has been renamed FIBULIN 3 (FBLN3) [48] and qualified to the family of fibulins, extracellular matrix proteins [49]. Six known members from mammalian species share an elongated structure and many calcium-binding sites and are hypothesized to function as intramolecular bridges that stabilize the structure of extracellular matrix [49].
A normal fibulin-3 interacts with several other proteins: a basement membrane protein (extracellular matrix protein-1), tissue inhibitor of metalloproteinase-3 (TIMP3), collagen XVIII/endostatin, hepatitis B virus-encoded X antigen, and elastin monomer tropoelastin. These interactions likely contribute to the integrity of basement membrane zone. Fibulin-3 colocalizes with fine elastic fibers and is involved in the formation of the extracellular matrix [50].
In vitro biochemical analysis indicates that mutant fibulin-3 protein containing the R345W mutation is misfolded. This change in conformation causes the mutant fibulin-3 to be secreted inefficiently and accumulate within cells [40]. In both ML and AMD eyes, fibulin-3 accumulates along drusen or other basal deposits [50]. Some cell culture studies suggested that mutant R345W fibulin-3 leads to macular degeneration through its misfolding and accumulation in the endoplasmic reticulum [51], causing cell death by activating the unfolded protein response [52]. However, the unfolded protein response has not been detected in in vivo studies [17]. A recent in vitro study suggested that R345W fibulin-3, but not the wild-type fibulin-3, required N-glycosylation to acquire a stable, native-like structure, enhancing the role of N-linked glycosylation in regulating the folding, secretion, and intracellular levels of fibulin-3 [53].
5.7 Animal Model
EFEMP1 −/− knockout mice, although appearing normal at birth, exhibited early-onset aging and developed multiple hernias, including inguinal hernias, pelvic prolapse, and protrusions of the xiphoid [54]. It showed reduced fertility and generalized fat, muscle, and organ atrophy. Histologic analysis revealed reduced and disrupted fine elastic fibers in fascia, adventitia, small blood vessels, and vaginal walls. There was no apparent retinal degeneration in the knockout mice [54].
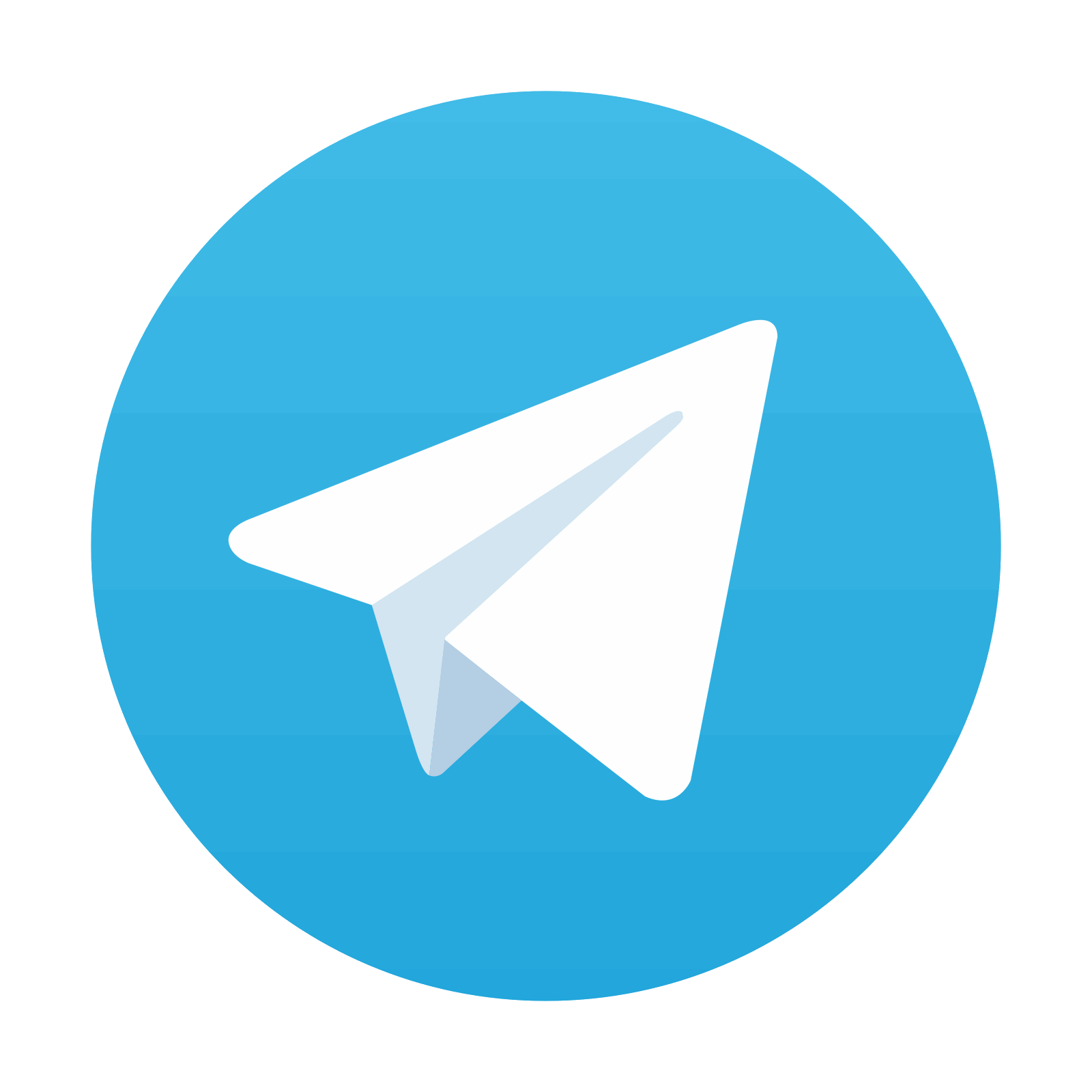
Stay updated, free articles. Join our Telegram channel

Full access? Get Clinical Tree
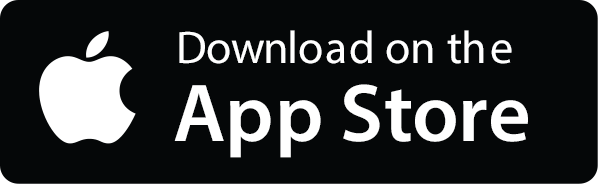
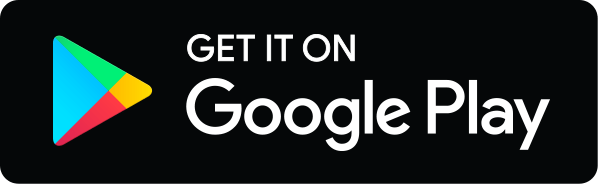