Recent magnetic resonance imaging (MRI) techniques have made it possible to examine the compartments of the cochlea using gadolidium-chelate (GdC) as a contrast agent. As GdC loads into the perilymph space without entering the endolymph in healthy inner ears, the technique provides possibilities to visualize the different cochlear compartments and evaluate the integrity of the inner ear barriers. This critical review presents the recent advancements in the inner ear MRI technology, contrast agent application and the correlated ototoxicity study, and the uptake dynamics of GdC in the inner ear. GdC causes inflammation of the mucosa of the middle ear, but there are no reports or evidence of toxicity-related changes in vivo either in animals or in humans. Intravenously administered GdC reached the guinea pig cochlea about 10 minutes after administration and loaded the scala tympani and scala vestibuli with the peak at 60 minutes. However, the perilymphatic loading peak was 80 to 100 minutes in mice after intravenous administration of GdC. In healthy animals the scala media did not load GdC. In mice in which GdC was administered topically onto the round window, loading of the cochlea peaked at 4 hours, at which time it reached the apex. The initial portions of the organ to be filled were the basal turn of the cochlea and vestibule. In animal models with endolymphatic hydrops (EH), bulging of the Reissner’s membrane was observed as deficit of GdC in the scala vestibuli. Histologically the degree of bulging correlated with the MR images. In animals with immune reaction-induced EH, MRI showed that EH could be limited to restricted regions of the inner ear, and in the same inner ear both EH and leakage of GdC into the scala media were visualized. More than 100 inner ear MRI scans have been performed to date in humans. Loading of GdC followed the pattern seen in animals, but the time frame was different. In intravenous delivery of double-dose GdC, the inner ear compartments were visualized after 4 hours. The uptake pattern of GdC in the perilymph of humans between 2 hours and 7 hours after local delivery needs to be clarified. In almost all patients with probable or suspected Ménière’s disease, EH was verified. Specific algorithms with a 12-pole coil using fluid attenuation inversion recovery sequences are recommended for initial imaging in humans.
Magnetic resonance imaging (MRI) of the delicate structures of the inner ear has been addressed with the use of high field strength magnets and various contrast agents in recent years. The inner ear is housed in dense bone and subdivided into different fluid-filled compartments that make imaging challenging. Methodological development in imaging techniques that has allowed separation of bone from fluid and contrast agent and increase of the magnetic field strength has improved spectral resolution, signal-to-noise and contrast-to-noise ratios and reduced scan acquisition times. These properties are particularly helpful in the attempt to resolve details between the minute fluid-filled spaces within the cochlea. Contrast agents to enhance or darken fluid or tissue signal help to visualize regions of interest and efforts are now being made to create biologic tags using these agents for molecular imaging at the level of cellular processes.
Visualization of endolymphatic hydrops (EH) in Meniere’s disease has always been an important goal for clinicians, but hitherto has been limited to postmortem histology. Histologic quantification of hydrops has been accomplished using a 3-step grading system to record the position of the Reissner’s membrane. Scientific reports have not yet been capable of systematically quantifying the extent of vestibular hydrops in vivo and establishing a correlation with symptoms, degree of hearing loss, and cochlear fluid dynamics. These difficulties are probably due in part to the variation in symptoms over time, including fluctuations in hearing level. This loose time dependency of symptoms is reflected in the grading of Meniere’s disease based on the American Academy of Otolaryngology-Head and Neck Surgery (AAO-HNS) classification scale. Requirements of AAO-HNS leave the distinction of certain elements of Meniere’s disease for histologic evaluation made by post mortem. In addition, other diseases that may be related to EH are not officially recognized, although in many instances the fluctuant hearing loss and recurrent attacks of rotatory vertigo occur, such as subtypes of sudden deafness and autoimmune disorders.
MRI diagnosis of Meniere’s disease was challenging until recent years. In humans, Mark and colleagues observed labyrinthine signal changes in gadolinium chelate (GdC)-enhanced MRI only in patients with sudden deafness and vertigo, but not in normal inner ears. In another human study, no uptake of GdC was detected in the inner ear fluids, despite using triple-dose intravenous administration and a 1.5-T scanner. In rodents, Counter and colleagues studied guinea pigs and visualized abundant uptake of Gd in the perilymph of both cochlea and vestibule after intravenous administration of 5 times the human dose of GdC (by weight) imaged with a 4.7-T scanner. Zou and colleagues performed mouse inner ear MRI studies (4.7 T) and observed efficient uptake of GdC with loading peak of 80 to 100 minutes after intravenous delivery. Direct visualization of EH in guinea pig was first reported by Zou and colleagues in 2000 and followed by Niyazov and colleagues in 2001. The controversial reports in the human studies compared with animal results raised 3 questions: Was the magnetic field too low in the human study? Was the concentration of GdC applied in humans too low? Was the observation time after intravenous administration of GdC in humans too short?
Alternatively, intratympanic administration of GdC provided efficient loading of the contrast agent in the inner ear perilymph and reduced the risk for systemic toxicity. MRI showed clear uptake of GdC in the perilymph of both rodents and humans after intratympanic delivery. Naganawa and colleagues improved the image quality and showed EH in humans using a three-dimensional (3D) fluid-attenuated inversion recovery (FLAIR) sequence in a 3-T machine.
This article describes the novel approaches that have been recently undertaken to visualize the inner ear. These approaches allow assessment of disease within the inner ear and also to follow dynamics regarding either recovery or deterioration of the diseases. Five major steps have been taken in the development of the ability to visualize enhancement within the human inner ear. These steps represent examples of translational research (ie, the development of a method in animal studies followed by application of the method to the benefit of human beings). The first step was to show that inner ear compartments could be distinguished using GdC-enhanced MRI. In normal inner ears of guinea pigs, it was observed that intravenously administered high doses (Omniscan 0.5 mmol/mL, 3 mL/kg intravenously) of GdC loaded the perilymph, but not the endolymph. The second step was to show that visualization of EH was possible in animal models with intravenously administered GdC. In animals with experimental EH, bulging of the Reissner’s membrane could be visualized and quantified. The third step was to show that when GdC was placed into the middle ear, the contrast agent passed through the middle-inner ear barriers (the round-window membrane) and the inner ear could be visualized. For the fourth, the optimal imaging time for humans was determined and was shown to be about 22 hours after application of the contrast agent into the tympanic cavity. However, more details were missing regarding the time points between 24 hours. For the fifth, modification of the sequences was necessary to increase the contrast between endolymph and perilymph with the lowest concentration of GdC in the perilymph. These sequences included 3D real inversion recovery (3D-real IR), rapid acquisition with relaxation enhancement (RARE), and FLAIR.
To date, more than 100 patients have been evaluated and the identification of EH is broadening into other disease entities besides Meniere’s syndrome, including recurrent vertigo, sudden deafness, and even superior canal dehiscence syndrome among others. The current challenges in inner ear imaging are to improve the delivery of contrast agent so that the concentration of GdC in the inner ear exceeds the detecting limit and to develop more sensitive sequences. Combination of intravenous and local deliveries might produce maximum delivery of contrast agents into the inner ear by passing through both the blood-perilymph barrier and middle-inner ear barriers with minimum systemic toxicity. Another approach would be the development of additional contrast agents, such as the more recently available super paramagnetic iron nanoparticles (SPION).
Contrast agents and their possible toxicity to the inner ear
MRI contrast agents are a group of contrast media used to improve the visibility of internal body structures in MRI. Most MRI contrast agents work through shortening the T1 (eg, Gd) or T2 (eg, iron oxide) relaxation time of protons located nearby. Reduction of T1 relaxation time results in a hypersignal, whereas reduced T2 relaxation time reduces both T2 and T2 ∗ signals. Contrast agents interact with adjacent protons to influence their signal characteristics and the effects are recorded as the longitudinal relaxivity (r1) and transverse relaxivity (r2). Higher r1 values result in brightening or enhancement of tissue signal and higher r2 values result in darkening. In T1 (longitudinal relaxation time)-weighted sequences, the high r1 values of GdC produce desirable bright positive contrast effects, but the r1 decreases rapidly in higher field strengths. Another disadvantage of GdC is that they require micromolar concentrations for visualization, whereas molecular imaging requires sensitivities in the nanomolar range. For these reasons, efforts are being made to synthesize nanoparticulate contrast agents with high relaxivities that when tagged with biologic markers allow for imaging of cellular process in high-strength magnetic fields. Some examples of these novel nanoparticles are liposomes or micelles that contain paramagnetic GdC, nanoparticles created from GdC, and SPIONs.
GdC Contrast Agents
The most commonly used clinical contrast agents are paramagnetic GdC that have longitudinal relaxivity (r1) values ranging from 10 to 20/s/mM. However, the commonly encountered hexahydrate GdCl 3 6H 2 O cannot be used as an MRI contrast agent because of its low solubility in water at the near neutral pH of the body. Free Gd (Gd 3+ ions), for example, GdCl 2 (H 2 O) 6 ] + , is toxic. Chelating the Gd is essential for biomedical applications. One representative chelating agent is H 5 DTPA (diethylenetriaminepentaacetic acid). Chelation to the conjugate base of this ligand increases the solubility of the Gd 3+ at the neutral pH of the body and still allows for the paramagnetic effect required for an MRI contrast agent. The DTPA 5- ligand binds to Gd through 5 oxygen atoms of the carboxylates and 3 nitrogen atoms of the amines. A ninth binding site remains, which is occupied by a water molecule. The rapid exchange of this water ligand with bulk water is a major reason for the signal-enhancing properties of the chelate. Two structurally distinct categories of GdC are currently marketed: (1) macrocyclic chelates (gadoterate, gadoteridol, or gadobutrol), in which the Gd 3+ ion is caged in the reorganized cavity of the ligand, and (2) linear chelates (gadopentetate, gadobenate, gadodiamide, gadoversetamide, gadofosveset/MS325, and gadoxetate). GdC can also be nonionic (or neutral), in which the number of carboxyl groups is reduced to 3, neutralizing the 3 positive charges of the Gd 3+ , or ionic, in which the remaining carboxyl groups are salified with meglumine or sodium.
Table 1 shows the GdC contrast agents in general use.
General Name | Commercial Name | Structure |
---|---|---|
Ionic contrast agents | ||
Gadopentetate dimeglumine | Magnevist | Gd-DTPA |
Gadobenate dimeglumine | Multihance | Gd-BOPTA |
Gadfosveset trisodium | Vasovist | Gd-DTPA-DPCP |
Gadoxetate disodium | Primovist | Gd-EOB-DTPA |
Gadoterate meglumine | Dotarem/Magnescope | Gd-DOTA |
Nonionic contrast agents | ||
Gadobtrol | Gadovist | Gd-BT-DO3A |
Gadodiamide | Omniscan | Gd-DTPA-BMA |
Gadoteridol | Prohance | Gd-HP-DO3A |
Gadoversetamide | Optimark | Gd-DTPA-BMEA |
Gadopentetate dimeglumine (Magnevist) is a formation composed of Gd 3+ complexed with DTPA (Gd-DTPA). DTPA is a chelator that surrounds Gd 3+ . It is reported that Gd-DTPA exists as free Gd in concentration of 10 −22 mol/L if the number of free Gd and the chelator (DTPA) are maintained at the same concentrations. Gadodiamide is a formulation composed of Gd 3+ complexed with DTPA bis-methylamide (Gd-DTPA-BMA). The DTPA remains a chelator that surrounds Gd 3+ and BMA maintains the contrast medium in a nonionized state. It is reported that of Gd-DTPA-BMA, 10 −17 mol/L exists as free Gd ions if the number of free Gd and the chelator (DTPA-BMA) are maintained at the same concentrations.
SPION
Two commercially available classes of iron oxide particles are used in MRI for detection of malignant tumors and metastatic disease. SPIONs are used to visualize liver metastases. The particles are phagocytized by hepatic macrophages in healthy tissue, but not in metastases, which are shown as bright lesions against the darkened normal background liver. An increase in signal intensity indicates altered capillary permeability in tumor. These specific signal changes are valuable for differentiating benign from malignant enlarged lymph nodes.
SPIONs currently in clinical use have r2 values ranging from 50 to more than 600/s/mM and the values are stable with increasing magnetic field strengths. Similar to GdC, the r1 values decrease with increasing field strength. Thus, in high magnetic fields of 3.0 T or more, SPIONs retain their strong darkening effect on T2 (transverse relaxation time)-weighted sequences, which would be seen in contrast to the bright signal ordinarily detected in fluid spaces. SPIONs targeted for specific cell types may be useful for differential imaging of the cochlea cell types.
SPIONs incorporated into cationic liposomes decorated with antitransferrin receptor single-chain antibody fragment for lung metastasis showed bright enhancement in lung nodules on T2 MR imaging. It seems that complexed nanoparticle contrast agents may have significant alterations in relaxivity that could offer promising new opportunities for cellular imaging.
Toxicity of GdC contrast agent
GdC administered intratympanically distributes in the whole perilymph in 12 hours but disappears within 1 week. It is important to evaluate how much GdC enters the perilymphatic space after intratympanic Gd administration. It is estimated that the concentration of GdC within the perilymph after the intratympanic administration of gadodiamide (Omniscan) diluted 8 times is 10 -4 mol/L, which is a 5000-times dilution of the original gadodiamide.
Intravenously applied GdC moves into cochlear fluid space, especially into the perilymph. Following intravenous GdC administration, at the peak GdC concentration, the cochlear fluid is enhanced to a level similar to that in the cerebellum, which is estimated to be an 8000- to 16,000-times dilution of the injected GdC solution. It is estimated that the maximum GdC concentration in the inner ear achieved with the intratympanic administration of the 8-times dilution is similar to that achieved by the threefold GdC intravenous injection. Even after 2-fold GdC intravenous injection, it was possible to show EH in patients with Meniere’s disease, although the GdC concentration in the perilymph was lower compared with that obtained after intratympanic GdC administration.
In cell cultures free Gd was toxic to isolated hair cells at a concentration of 10 −5 mol/L (Jing Zou and Colleagues, unpublished data). Kakigi and colleagues reported that intratympanically administered Gd-DTPA-BMA had adverse effects on the stria vascularis in guinea pigs. When Gd-DTPA-BMA was diluted 8 times, no adverse effect on the stria vascularis was observed. Kakigi and colleagues also recorded reduction of endolymph potential and enlarged intercellular gap of intermediate cells in the cochleae at 60 minutes after receiving intratympanic administration of nondiluted Gd-DTPA-BMA, but not in the cochleae subjected to 8-times dilution of the contrast agent. Kimitsuki and colleagues observed that free Gd blocked mechanoelectric transducer current in chick cochlear hair cells by decreasing the inward going mechanoelectric transducer currents, specifically the Ca 2+ component. There were reports of Ca 2+ channel activities and transient receptor potential channel vanilloid subfamily 4 expression in the stria vascularis. However, Gd in Gd-DTPA-BMA behaves differently from free Gd ion.
The amount of GdC administered by intratympanic injection is less than 0.1% of that given by ordinary intravenous injection. Accordingly systemic adverse effects of the intratympanically delivered agent may be negligible, but adverse effects on the inner ear must be evaluated carefully. The mechanism is unknown regarding the interference of Gd-DTPA-BMA with endocochlear potential. Our preliminary study with 9 healthy guinea pigs after intratympanic administration of nondiluted Gd-DTPA-BMA did not show threshold shift in tone auditory brainstem response (Ilmari Pyykkö, MD and Jing Zou, MD, unpublished data acquired in Stockholm). However, the middle ear mucosa showed inflammatory changes. In humans, Fukuoka and colleagues injected 8-fold dilutions of Gd-DTPA-BMA intratympanically into the ears of the diseased side and the contralateral healthy side, but no adverse effects were observed, even in the healthy ears.
To avoid any complications with free Gd it is recommended to use GdC immediately after the package is opened. Once the package is opened, the free Gd concentration may increase, even if the solution is kept in a refrigerator.
To our knowledge, there has been no report of any adverse effect on the inner ear in humans after any GdC administration, even in patients with nephrogenic systemic fibrosis. Nephrogenic systemic fibrosis is the most serious complication caused by intravenous GdC administration. When GdC remains in the body for a long time, the chelator surrounding the Gd gradually separates and the toxicity of free Gd becomes apparent. Nephrogenic systemic fibrosis occurs in relation to renal diseases that prevent free Gd excretion from the body, therefore its use is not recommended in patients with impaired kidney function. The incidence of nephrogenic systemic fibrosis seems to depend on the type of the GdC contrast agent. Altun and colleagues reported that nephrogenic systemic fibrosis was not observed with the application of Gd-DTPA or Gd-DOTA rather than Gd-DTPA-BMA. Gd-based contrast media may be nephrotoxic even at approved doses. The toxicity of free Gd to the inner ear should be further investigated from various perspectives.
Toxicity of GdC contrast agent
GdC administered intratympanically distributes in the whole perilymph in 12 hours but disappears within 1 week. It is important to evaluate how much GdC enters the perilymphatic space after intratympanic Gd administration. It is estimated that the concentration of GdC within the perilymph after the intratympanic administration of gadodiamide (Omniscan) diluted 8 times is 10 -4 mol/L, which is a 5000-times dilution of the original gadodiamide.
Intravenously applied GdC moves into cochlear fluid space, especially into the perilymph. Following intravenous GdC administration, at the peak GdC concentration, the cochlear fluid is enhanced to a level similar to that in the cerebellum, which is estimated to be an 8000- to 16,000-times dilution of the injected GdC solution. It is estimated that the maximum GdC concentration in the inner ear achieved with the intratympanic administration of the 8-times dilution is similar to that achieved by the threefold GdC intravenous injection. Even after 2-fold GdC intravenous injection, it was possible to show EH in patients with Meniere’s disease, although the GdC concentration in the perilymph was lower compared with that obtained after intratympanic GdC administration.
In cell cultures free Gd was toxic to isolated hair cells at a concentration of 10 −5 mol/L (Jing Zou and Colleagues, unpublished data). Kakigi and colleagues reported that intratympanically administered Gd-DTPA-BMA had adverse effects on the stria vascularis in guinea pigs. When Gd-DTPA-BMA was diluted 8 times, no adverse effect on the stria vascularis was observed. Kakigi and colleagues also recorded reduction of endolymph potential and enlarged intercellular gap of intermediate cells in the cochleae at 60 minutes after receiving intratympanic administration of nondiluted Gd-DTPA-BMA, but not in the cochleae subjected to 8-times dilution of the contrast agent. Kimitsuki and colleagues observed that free Gd blocked mechanoelectric transducer current in chick cochlear hair cells by decreasing the inward going mechanoelectric transducer currents, specifically the Ca 2+ component. There were reports of Ca 2+ channel activities and transient receptor potential channel vanilloid subfamily 4 expression in the stria vascularis. However, Gd in Gd-DTPA-BMA behaves differently from free Gd ion.
The amount of GdC administered by intratympanic injection is less than 0.1% of that given by ordinary intravenous injection. Accordingly systemic adverse effects of the intratympanically delivered agent may be negligible, but adverse effects on the inner ear must be evaluated carefully. The mechanism is unknown regarding the interference of Gd-DTPA-BMA with endocochlear potential. Our preliminary study with 9 healthy guinea pigs after intratympanic administration of nondiluted Gd-DTPA-BMA did not show threshold shift in tone auditory brainstem response (Ilmari Pyykkö, MD and Jing Zou, MD, unpublished data acquired in Stockholm). However, the middle ear mucosa showed inflammatory changes. In humans, Fukuoka and colleagues injected 8-fold dilutions of Gd-DTPA-BMA intratympanically into the ears of the diseased side and the contralateral healthy side, but no adverse effects were observed, even in the healthy ears.
To avoid any complications with free Gd it is recommended to use GdC immediately after the package is opened. Once the package is opened, the free Gd concentration may increase, even if the solution is kept in a refrigerator.
To our knowledge, there has been no report of any adverse effect on the inner ear in humans after any GdC administration, even in patients with nephrogenic systemic fibrosis. Nephrogenic systemic fibrosis is the most serious complication caused by intravenous GdC administration. When GdC remains in the body for a long time, the chelator surrounding the Gd gradually separates and the toxicity of free Gd becomes apparent. Nephrogenic systemic fibrosis occurs in relation to renal diseases that prevent free Gd excretion from the body, therefore its use is not recommended in patients with impaired kidney function. The incidence of nephrogenic systemic fibrosis seems to depend on the type of the GdC contrast agent. Altun and colleagues reported that nephrogenic systemic fibrosis was not observed with the application of Gd-DTPA or Gd-DOTA rather than Gd-DTPA-BMA. Gd-based contrast media may be nephrotoxic even at approved doses. The toxicity of free Gd to the inner ear should be further investigated from various perspectives.
Application of contrast agents
Intravenous Delivery of GdC
To deliver the contrast agent, the most extensively applied approach is intravenous injection. Because of its molecular size, GdC does not pass through the normal blood-brain barrier, thus making it valuable by visualizing the leakage of GdC through a damaged blood-brain barrier in diseases. The inner ear biologic barrier system is similar to the brain in that GdC does not pass through the normal blood-endolymph barrier and causes enhancement when lesions to the barrier occur as a result of mechanical force and inflammation. Because the cochlear compartments are separated by the Reissner’s membrane and the basilar membrane, which limit the passage of GdC, the efficient passage of GdC through the blood-perilymph barrier highlights the scala tympani and scala vestibuli, but not the scala media. A shift of the border between the dark scala media and bright scala vestibuli or scala tympani indicates a volume change in the endolymph. EH was first shown by such alterations in GdC enhancement within the scala vestibuli by MRI in both animal models and Meniere’s disease. The dosage applied in animals was 1.5 mmol/kg and 0.2 mmol/kg to 0.3 mmol/kg in humans. At lower doses, the concentration of contrast was insufficient to clearly separate endolymph from perilymph, even using 3D FLAIR sequences with an 8-channel coil and a 3-T magnet. Some patients have been studied using double-dose intravenous Gd contrast and imaging with a 32-channel head coil at 3 T. In those patients with Meniere’s disease, EH was successfully visualized, despite the concentration of Gd-DTPA tending to be lower than with an intratympanic injection of 8-fold diluted Gd ( Fig. 1 ). In comparison, the routine dosage of GdC in the clinic is 0.1 mmol/kg and the maximum dose approved by the US Food and Drug Administration is 0.3 mmol/kg.
Intratympanic Administration of GdC
Intratympanic administration of GdC can potentially induce higher concentrations of contrast agent into the perilymph than by intravenous administration. There are 2 potential pathways for the MRI contrast agents to enter the inner ear from the tympanic cavity. One is the round-window membrane and the other is the annular ligament of the stapediovestibular joint. The transport efficacy of substances through these barriers may vary and depends on the size and surface characteristic of the particles and charge of the compounds.
Compounds may diffuse through the round-window membrane via paracellular pathways and extracellular matrix or by internalization via endocytosis (the process of uptake of macromolecules into cells by enclosing them in membrane vesicles). Three mechanisms are involved in pinocytosis: macropinocytosis, clathrin-mediated endocytosis, and caveolin-mediated endocytosis. In general, particles greater than 1 μm are internalized by macropinocytosis, particles with the size of around 120 nm are taken up by clathrin-mediated endocytosis, and 50- to 60-nm particles are internalized by caveolin-mediated endocytosis. Small and nonpolar molecules such as O 2 and CO 2 can readily diffuse across the lipid bilayer. However, polar molecules such as Gd 3+ ions are incapable of crossing the plasma membrane on their own. Gd 3+ ions can be transported across the lipid bilayer through specialized membrane-transport Ca 2+ channel by mimicking the properties of calcium. Most other nanoscale molecular assemblies are internalized through endocytosis on contact with the cell membrane. Ultrastructural studies of the round-window membrane of the cat showed that cationic ferritin, placed for 2 hours in the round-window niche of 4 normal cats, was observed to traverse the round-window membrane through pinocytotic vesicles into the connective tissue layer. Evidence of exocytosis of tracer by the inner epithelial layer into the scala tympani was presented. When placed in perilymph, this same tracer was incorporated by inner epithelial cells, suggesting transmembrane trafficking properties of the round-window membrane.
Intratympanic delivery of GdC allows for efficient visualization of the cochlear compartments in both animals and humans. GdC, diluted 5-fold, was optimal for imaging the animal cochlear compartments and diluted 8-fold was reasonable for imaging the cochlear scalae in humans. GdC has been delivered to the round-window membrane by injection through the tympanic membrane, by topical application of gelatin sponge, or by installation of a cannula in the middle ear or bulla. Transtympanic injection is the least traumatic and is as efficient as the gelatin sponge technique, making it more practical for clinical application.
In humans GdC has been delivered into the middle ear by transtympanic injection. Two tiny spots (1 mm) on the tympanic membrane (one in the hypotympanic part, the other facing the Eustachian tube orifice) were topically anesthetized with 90% phenol solution ( Fig. 2 ). A small hole in the tympanic membrane was made by the needle in the anesthetized anterior-superior spot to vent air during the injection. Through a 22-G needle, 0.5 mL of Gd contrast agent was injected through the hypotympanic spot into the middle ear cavity. The patient was kept recumbent with the treated diseased ear up for 15 to 30 minutes. Thereafter, the patient was allowed to move freely.
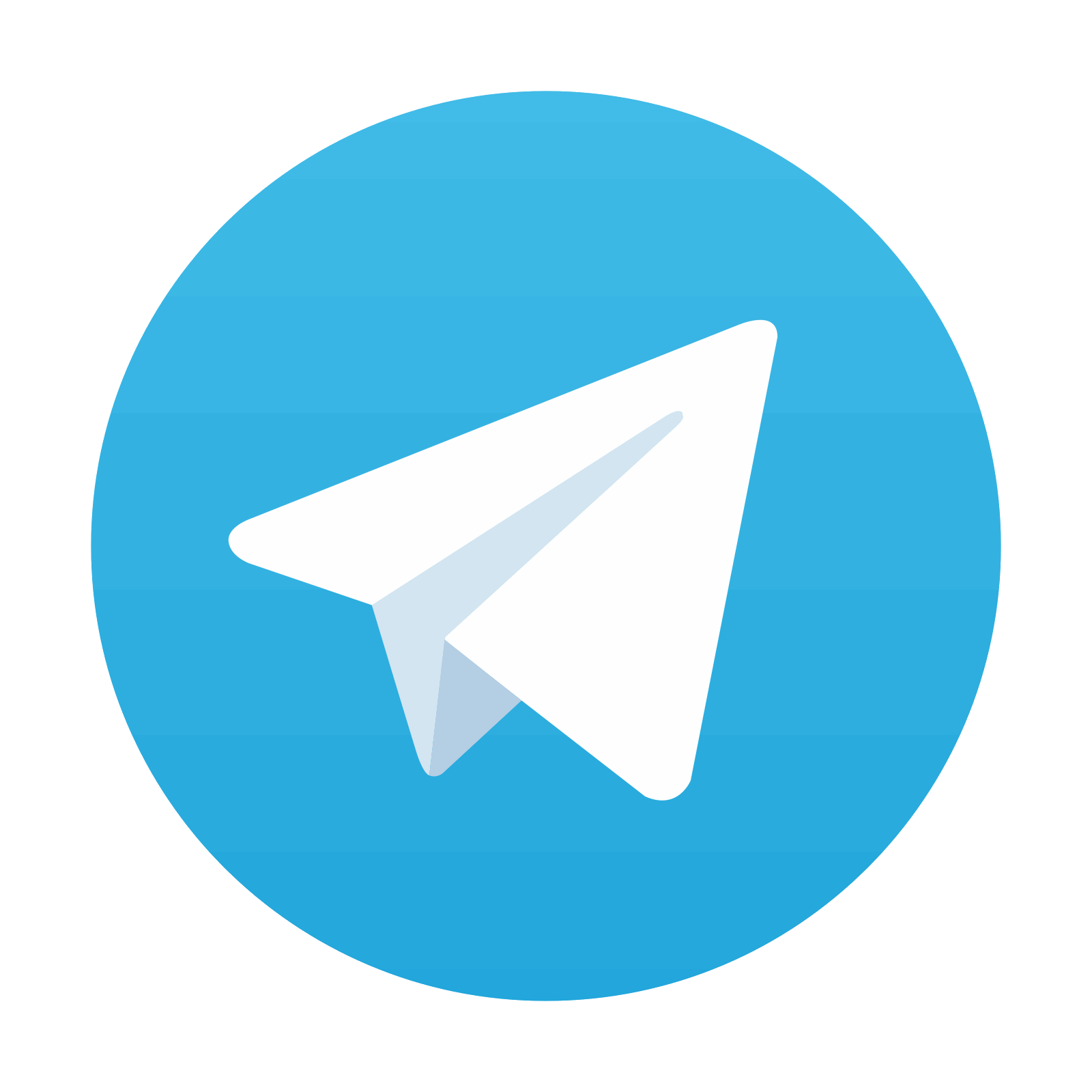
Stay updated, free articles. Join our Telegram channel

Full access? Get Clinical Tree
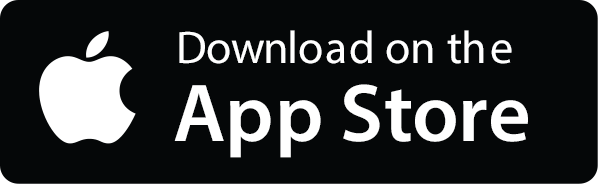
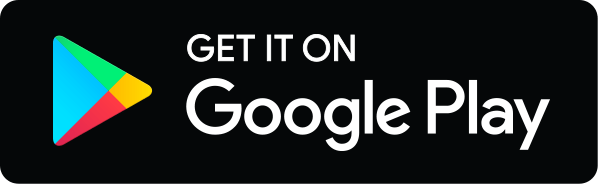
