Fig. 16.1
Surgical technique of the first successful composite total laryngeal transplant. Vascular anastomoses included the donor right internal jugular vein to the recipient right facial vein, donor left middle thyroid vein to recipient left internal jugular vein, donor superior thyroid arteries to the recipient superior thyroid arteries. Nerve anastomoses included both superior laryngeal nerves and the only the right recurrent laryngeal nerve. [Reprinted with permission, Cleveland Clinic Center for Medical Art & Photography© 2015. All Rights Reserved.]
The recipient received cyclosporine, azathioprine, and methylprednisolone prior to surgery. Perfusion was re-established to the donor organ as the first step of the transplant via the donor’s right superior thyroid artery anastomosed to the same artery of the recipient. The proximal end of the donor’s right internal jugular vein was anastomosed to the patient’s right common facial vein. Blood flow through the transplanted thyroid gland, six tracheal rings, larynx, and pharynx was observed within 30 min of clamp release.
Once perfusion was established, a narrow field laryngectomy was performed on the recipient. His thyroid lobes were kept in situ but lateralized and the hyoid bone was left in place. A majority of the donor’s pharynx was utilized to widen the patient’s stenotic pharyngoesophageal complex. The donor laryngeal cartilage was sutured to the hyoid bone to create fixed, laryngeal elevation. Five of the donor’s tracheal rings were sutured to the recipient tracheostoma. The stoma was left open given that bilateral vocal fold paralysis in the cadaveric position was anticipated.
The left-sided vascular anastomoses were then completed, including the donor’s superior thyroid artery to the recipient’s superior thyroid artery and the donor middle thyroid vein to the recipient internal jugular vein. Both superior laryngeal nerves were located and reanastomosed. Only the recipient’s right recurrent laryngeal nerve could be located for reinnervation to the donor’s right recurrent laryngeal nerve. The donor’s left recurrent laryngeal nerve was allowed to remain free within the soft tissues of the neck, and not connected to a recipient nerve. A gastrostomy tube was placed for perioperative nutrition.
Postoperatively, the patient was maintained on muromonab-CD3, cyclosporine, methylprednisolone, and mycophenolate mofetil. Initial aspiration was controlled with glycopyrrolate and atropine, which were later discontinued. After observation in the hospital for 1 month, the patient’s transplanted trachea was noted to be slightly edematous on endoscopy but showed no signs of rejection on biopsy. Three months post-transplant, the supraglottis and vocal folds became sensitive to touch. Purposeful swallowing, taste, and smell returned. Subsequent barium swallows revealed no aspiration. To evaluate thyroid function, a 4 h uptake of iodine-123 demonstrated 83% activity in the transplanted thyroid lobes, as well as 17% in the patient’s native thyroid.
The first post-transplant voicing occurred on the third postoperative day. Endoscopy at 1 month revealed that both true vocal folds were in a lateral (cadaveric) position, creating a breathy voice. The right fold (the side of the recurrent nerve anastomosis) was midline at the 4 month endoscopy. By 6 months post-transplant, the left had medialized. Neither vocal fold demonstrated abduction, but rather appeared to be synkinetic. Electromyographic (EMG) measurements confirmed reinnervation of both thyroarytenoid muscles and bilateral volitional cricothyroid function [12] (Figs. 16.2a,b). It was postulated that the innervation on the left was likely due to “field-reinnervation,” in which the left thyroarytenoid muscle is supplied by surrounding motor nerves. Subjective and objective measures of phonation including pitch, jitter, intensity, and maximal phonation time were within the normal range at 36 months post-transplant. As a vocation, the patient became a motivational speaker and stated his quality of life improved “immeasurably” subsequent to the transplantation.


Fig. 16.2
EMG results in laryngeal transplant patient at 3 years. (a) EMG findings at rest, including bilateral thyroarytenoid muscles (TA) and cricothyroid (CT) muscles. (b) EMG findings during sustained/ee/phonation (TA) and raising and lowering of pitch (CT). Upon volitional movement, the left TA and CT muscles demonstrated moderately reduced recruitment patterns, whereas the right muscle groups showed mild reductions in recruitment. All 4 muscles demonstrated increased numbers of polyphasic motor unit potentials of mildly increased duration and normal amplitude, firing at moderate to rapid rates
Postoperative complications included three early episodes of tracheobronchitis which were successfully treated with oral amoxicillin clavulanate. At 16 weeks post-transplant, the patient inadvertently stopped his trimethoprim sulfamethoxazole and developed Pneumocystis carinii pneumonia, which cleared rapidly with intravenous antibiotics. Fifteen months post-transplant, the patient presented with a decrease in voice quality. He was noted to have an episode of acute rejection. After three daily doses of methylprednisolone 1gm/day, his larynx and voice returned to normal. A second episode of rejection occurred about 5 years later due to laboratory error in tacrolimus values measuring levels falsely high. As a result the patient’s medication became below therapeutic levels. Laryngeal edema was observed during the acute rejection episode, but returned to normal once medication levels returned to the therapeutic range.
16.3 Immunosuppression Reduction
As previously discussed, the primary limitation to laryngeal transplantation, and transplantation in general, is immunosuppression. Our lab and others have investigated possible ways to reduce the amount of immunosuppression given to a patient while still preventing rejection.
A variety of animal models have been developed to lessen the necessary immunosuppression for laryngeal transplantation including dog [1–3], mouse [13, 14], and pig [15]. All offer complementary information forming the basis on which to build further understanding into laryngeal transplantation. The two authors’ work focused on the rat and mouse models since they are well-established transplant models, significant information is known about their immune systems, and are relatively inexpensive. This model places the transplanted organ in tandem with the native larynx (Fig. 16.3a–c). In 2002, the rat model’s revisions were published, as well as a revised grading scale of rejection [16]. The mouse model [14] and a mouse rejection grading scale [17] were published later. Over time and more than 3000 transplants, these models have shown overall nearly 100% survivability, and a greater than 90% graft evaluability. Furthermore, it was demonstrated that performing a total parathyroidectomy on the recipient during transplantation can utilize the production of parathormone from the rat transplanted larynx as a marker of graft viability rather than having to sacrifice the animal for histologic evaluation [18]. This has allowed for a new generation of studies examining the ability to “pulse” immunosuppressives and “salvage” the organ if parathormone levels drop.


Fig. 16.3
(a–c) Mouse and rat laryngeal transplantation diagram. Arterial inflow is achieved through the donor common carotid artery anastomosed to recipient common carotid artery. Direct graft inflow and outflow is based on superior thyroid artery pedicled via the external carotid artery. Venous outflow continues through the donor contralateral common carotid artery anastomosed to recipient internal jugular vein. (a) [Reprinted with permission, Cleveland Clinic Center for Medical Art & Photography© 2015. All Rights Reserved]
In early studies on immunosuppression for laryngeal transplantation, Haug et al. [19] have correlated the laryngeal rejection grade, cyclosporine A (CSA) concentration, and CSA intramuscular dosing. They found that significant pathologic allograft rejection correlated with CSA concentrations below 250 ng/cc. This study helped to develop the minimum level of CSA required to obtain optimum graft survival when used as the sole agent for immunosuppression.
Lorenz et al. [20] studied in rats the ability to decrease the amount of CSA by adding prednisone to cyclosporine. In a multiarm study containing 220 transplantations, multiple doses of both cyclosporine and prednisone were administered. The transplanted organs were evaluated at both 15 days and 30 days post-transplantation. It was demonstrated that the addition of 1.0 mg/kg/day of prednisone allowed CSA doses to be reduced to 2.0 mg/kg and still demonstrate no significant rejection at 30 days post-transplantation. Although the combination of low-dose CSA and prednisone significantly improved graft survival when compared to CSA alone at the equivalent dose, prednisone monotherapy demonstrated rates of rejection similar to no immunosuppression at all.
Additional studies further demonstrated that combination therapy of other immunosuppressives would also allow for decreased levels while maintaining graft viability [21, 22]. Akst et al. [22] treated transplanted rats with tacrolimus and mouse anti-rat alpha beta T-cell receptor monoclonal antibodies (αβ-TCR) for only 7 days following the transplant. All grafts demonstrated viability at 100 days. Skin grafting, mixed lymphocyte reaction, and flow cytometry revealed that tolerance was neither donor specific nor related to prolonged depletion of T-cell populations.
16.4 Immunosuppression in the Cancer Patient
The ability to induce tolerance with very low doses of immunosuppression and subsequent reduction of the undesirable side effects facilitates the ability to transplant more patients. However, that degree of immunosuppression is still prohibitive in cancer patients, which represents the vast majority of laryngeal transplantation candidates. It may be allowable to transplant patients with large benign or low-grade malignant laryngeal tumors or those with laryngeal cancer who are already on a post-transplant immunosuppression regimen. A patient with a combination of laryngotracheal stenosis who was post kidney-pancreas transplant underwent laryngeal transplantation under such circumstances in 2010 [23], but these patients are relatively rare.
The recipient pool may be further expanded by transplanting patients who have already undergone laryngectomy for cancer and there is no sign of recurrent cancer after 5 years. Ultimately, the largest group of patients who stand to benefit are those presenting with locally advanced laryngeal cancer. There are approximately 7000 new cases of advanced laryngeal cancer per year in the United States [24]. To date, two transplants have been performed for locally advanced head and neck cancer reconstruction—the non-revascularized partial laryngeal transplant in 19694 and a tongue transplant in 2003. Unfortunately, both patients rapidly succumbed to recurrent disease. There are at least three areas of investigation that are promising for possible transplantation in malignancy. The first is to add an antineoplastic agent to the immunosuppression regimen. The second is to directly modify immune cells to maintain immunocompetence while accepting the transplant. Lastly, bioengineering of an organ with the patients own cells should bypass the need for immunosuppression altogether. The second and third areas will be discussed later in the chapter.
Everolimus (SDZ-RAD) is an intriguing immunosuppressive option for transplantation in cancer patients [25]. It is a derivative of rapamycin and belongs to the mTOR (mammalian target of rapamycin) class of immunosuppressants. It blocks the translation of mRNA in critical cell cycle regulatory proteins, thereby inhibiting proliferation of intimal cells, lymphocytes, and tumor cells. Everolimus has shown both immunosuppressive and antitumor properties. The antitumor properties have been demonstrated in several animal and human tumor cell lines, including squamous cell carcinoma [26–28], which is the predominant laryngeal cancer type. In addition, it has been shown to inhibit the development of post transplant lymphoproliferative disorders [29].
Data from our laryngeal transplant models have provided support for the use of everolimus as an effective immunosuppressive and antineoplastic agent in laryngeal transplantation [30, 31]. A mouse squamous cell carcinoma cell-line was used to determine the effect of everolimus on the growth of both intradermal tumors and pulmonary metastases [28]. Tumor inhibition occurred in both the intradermal primary tumors and pulmonary metastasis models studied (Figures 16.4a–d). The authors concluded that everolimus provides potent tumor inhibition in animals inoculated with squamous cell cancer cells by decreasing local spread of disease as well as distant metastases. Everolimus was then studied as a single agent immunosuppressive in the mouse transplantation model [31]. The study showed that everolimus monotherapy successfully prevented laryngeal allograft rejection up to the study endpoint (60 days post-transplantation). (Fig. 16.5) It appeared to increase the production of regulatory T-cells while decreasing the cytotoxic T-cell and dendritic cell response. This is a favorable environment for transplantation.





Fig. 16.4
Results of everolimus therapy in mouse squamous cell carcinoma. (a) Intradermal tumor in untreated animal. (b) Intradermal tumor in treated animal. (c) Pulmonary metastasis in untreated animal. (d) Pulmonary metastasis in treated animal

Fig. 16.5
Preservation of transplanted larynx with everolimus monotherapy. Hematoxylin-eosinophil analysis of laryngeal allograft at 60 days post-transplant. Note moderate lymphocytic infiltration with normal structures including ciliated epithelium. (1) epithelium; (2) minor salivary glands; (3) fat; (4) thyroid gland; (5) muscle; (6) perichondrium; (7) cartilage; (8) lymphocytic infiltration
Once everolimus demonstrated efficacy for both immunosuppression and antineoplastic activity in our mouse transplantation model, our lab investigated the feasibility of using it as a combination therapy (everolimus and αβ-TCR) in a pulsed-dose regimen to reduce the total amount of immunosuppression and induce tolerance [32]. This study had the remarkable finding that tolerance can be achieved via a pulsed-dose combination therapy for 10 months with just a total of 15 days of immunosuppression. The promising immunosuppressive and antitumor profile of everolimus continues to suggest a prominent role for this agent in the future of laryngeal transplantation, and may 1 day serve to enable transplantation in the setting of malignancy.
16.5 Immunomodulation
An ideal immunosuppression regimen would be metabolically inert and induce donor-specific tolerance while leaving the remainder of the recipient’s immune system functional. One way to achieve this would be to directly alter immune cells. This concept was supported by Genden et al. [33] when they demonstrated that a single injection of UV-B irradiated donor splenocytes was sufficient to prevent rejection in a rat tracheal graft model.
To further investigate immunomodulation as a viable immunosuppressive regimen for laryngeal transplantation, several studies were undertaken to better understand the immunological environment of the larynx and trachea. A dense, organized network of immunologically active cells has been identified in both pig and human laryngeal mucosa [34, 35]. The morphology of the cells in these early studies was suggestive of dendritic cells. These dendritic cells were more closely analyzed by Friedman et al. [36] in the rat model. In that study, rat larynges were stained for dendritic cells and enumerated by a combination of immunofluorescence, confocal microscopy, and imaging software. They found that the vast majority of dendritic cells were located in epithelium and subepithelium when compared to other laryngeal subsites. The epithelium was 11 times more highly populated than the subepithelium. Interestingly, Govindaraj et al. [37] studied the role of tracheal epithelium in the rejection process. Their studies of mouse tracheal grafts showed that replacement of the epithelium by host epithelial cells prevents rejection after withdrawal of immunosuppression.
The vastly increased density of dendritic cells in the epithelium coupled with the knowledge of the important role the epithelium has in graft rejection suggested an important role of dendritic cells in transplant tolerance. Dendritic cells are the primary antigen presenting cells in the body and have a large role in determining graft rejection or survival. In the immature state (lack of a T-cell costimulatory molecule), they work to induce tolerance through T-cell anergy and apoptosis. Once maturation occurs through the presentation of a T-cell costimulatory molecule, dendritic cells facilitate rejection.
Lott et al. [38] were able to modify dendritic cells to become maturation resistant through the addition of nuclear factor kappa B (NF-κB) decoy oligodeoxyribonucleotides. This results in the suppression of costimulatory molecule expression causing resistance to maturation even after contact with activated allogenic T-cells, lipopolysaccharide [39], and antigen [40]. In their study, a single injection of immature dendritic cells (iDC) were injected into mice 7 days before transplantation. iDC-treated mice had significantly less rejection at all time points compared to non-immunosuppressed mice. Flow cytometry showed inhibition of cytotoxic T-cell infiltration and expansion of regulatory T-cells at the allograft site. The authors postulated that iDC immunotherapy may facilitate graft tolerance as a monotherapy or favorably affect concurrent immunosuppressive dose sequencing.
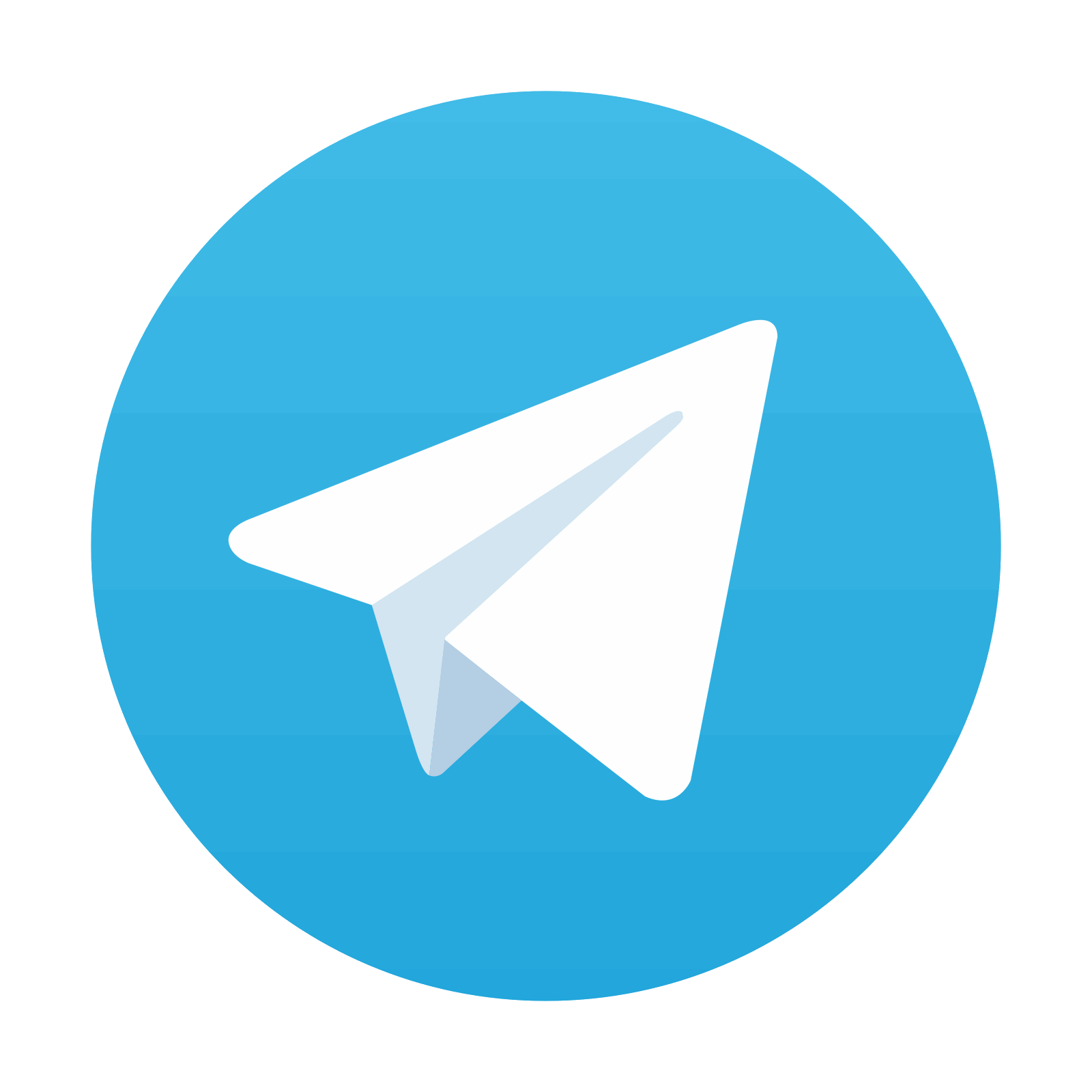
Stay updated, free articles. Join our Telegram channel

Full access? Get Clinical Tree
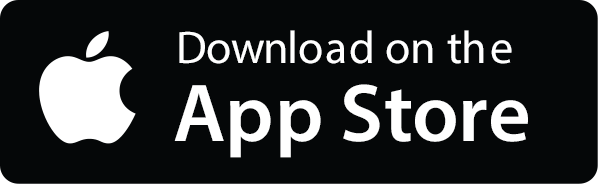
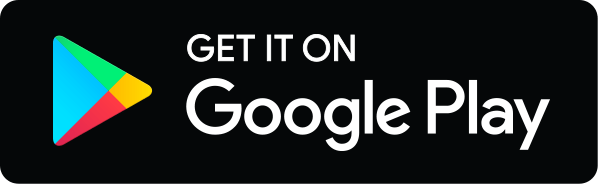