Fig. 14.1
(a) Synkinetic Reinnervation right side. Abduction inhibition right side (green marked RLN) because of co-stimulation of the PCA and LCA muscles, due to sprouting of abductor axons into adductor RLN fibers after trauma (T). (b) Adduction inhibition right side (red marked RLN) because of co-stimulation of the LCA and PCA muscles, due to sprouting of adductor axons into abductor RLN fibers after trauma (T)
This phenomenon, known from facial nerve paresis, causing an autoparalytic reinnervation (synchronous activation of agonist and antagonist muscles), is called “laryngeal synkinesis” and was described for the first time by Crumley in 1982 [1, 2]. Crumley distinguished four types of laryngeal synkinesis [3]. Type I does not involve any relevant symptoms concerning the respiration or the voice quality in case of a unilateral paresis (UVFP) because the vocal folds muscles are well preserved, allowing an appropriate closure of the glottal gap during phonation. In the event of bilateral vocal fold paresis (BVFP), voice quality is generally unaffected and due to residual vocal fold mobility, the respiratory function is restricted only during physical exertion, but not at rest. Type II synkinesis is characterized by involuntary vocal fold jerks, twitches, or jumps. It is dominated by moderate-to-severe adductor spasmodic-like dysphonia with unpredictable changes in the voice quality. In case of bilateral paralysis, respiration is affected at times. In particular, patients may experience short-term dyspnea caused by minimal saliva aspiration. In type III synkinesis, the adductor rather than the abductor reinnervation is predominant, in particular in regards to the m. cricoarytenoideus lateralis (LCA). As a result the vocal fold assumes a tight, almost median position and loses any relevant residual mobility. This can cause respiratory problems even in unilateral paralyses. If there is a BVFP with this type of synkinesis, a tracheotomy or an early glottis enlargement is often necessary. Voice quality is generally only slightly hampered or nearly unaffected. In contrast to type III, type IV synkinesis displays a predominance of reinnervated abductor muscles with vocal folds immobilized in a more lateral position. In this case respiration is only slightly affected, but voice quality is strongly impaired.
High-grade nerve lesions accompanied by foreseeable wrong reinnervation may be predicted via larynx-EMG (LEMG), a diagnostic tool that may also offer a suitable assistance for timing of the patient’s therapy. Patients with BVFP should also receive speech-language therapy, which should help limit compensatory supraglottic constriction and adapting the breathing technique to that of a stenotic airway [4].
At present, basic research is being undertaken to investigate to what extent the process of reinnervation and the level of synkinesis can be influenced therapeutically. Animal models (rats) have shown that wrapping of RLN-anastomoses with olfactory ensheathing cells, expressing trophic and nerve growth factors, induce an improved synchronization to the respiratory rhythm of the laryngeal muscles after reinnervation [5]. Stem cells derived from bone marrow failed to improve facial nerve reinnervation. Further basic research in animal models is exploring whether continuous electrical stimulation during the reinnervation process could improve the reinnervation of the laryngeal muscles [6, 7]. Hydman first examined the impact of the regeneration-promoting agent nimodipine on reinnervation and neuromuscular function following RLN crush injury [8]. Recent clinical trials showed that through the systemic administration of nimodipine it was possible to achieve a tripled vocal fold motion recovery rate in patients with an acute RLN paresis, in contrast to the untreated reinnervation [9].
14.2 Neurostimulation
In the 1950s and 1960s of the last century researchers made several attempts [10, 11] to inhibit atrophies in denervated muscles by means of therapies based on transcutaneous electrical stimulation. More than 60 years later, there is no clear evidence to confirm that electrostimulation therapy of denervated muscles is truly able to prevent fibrosis and maintain muscle flexibility after peripheral nerve paralysis. This is also the recent status in pareses with a high chance of spontaneous recovery, like in Bell’s facial nerve paresis [12]. The possible effects of the electrostimulation therapy are regarded as depending on the frequency range of the electric currents. For instance, frequencies above 100 kHz have no stimulation effects. They are used to warm up the tissue in thermotherapy. In low frequency ranges, up to 1 kHz, a single pulse with sufficient intensity and duration can generate muscle contraction. The current intensities, required to generate a contraction, may cause skin irritations, pain, and an undesired current spreading to other muscles [13]. In the future, optogenetic stimulation could be an alternative to selective muscle stimulation. An experiment with transgenic mice has shown that vocal fold abduction could be induced via optical stimulation [14]. However, in this case, the stimulation is effective only if, along with a direct contact between the optical fibers and the muscle, a specific gene mutation of the muscle fibers is present or induced. These requirements make the clinical application of such a therapy a distant prospect.
For muscles underwent reinnervation during the course of disease, indirect muscle stimulation known as neuromuscular electrical stimulation (NMES) may be applied. Since this method is based on nerve rather than muscle stimulation, a 10- to 100-fold lower stimulation intensity is needed than that used for direct muscle stimulation. In otolaryngology, NMES is used for surface stimulation with adhesive electrodes, for example in post-stroke dysphagia and unilateral vocal fold paresis [15]. If NMES is combined with voluntary activity, a strengthening of the weakened muscle tissue seems to be achievable [16]. According to Ptok [17] and Garcia Perez [18], at least in UVFP patients, voice therapy combined with NMES has a higher rate of success than voice therapy alone. Sometimes patients may report that there is a carry-over effect of voice improvement after NMES therapy. However, there is still a lack of knowledge regarding the adaptive mechanisms in cortical connectivity after peripheral nerve stimulation, that enhances the recovery of some amount of voluntary vocal fold movement [19]. In general, neuromuscular stimulation is based on the delivery of peripheral electrical stimuli. Transcranial magnetic stimulation (TMS) may be an alternative, but within a very limited range of indications. To our knowledge TMS is exclusively used for diagnostic purposes [20].
14.3 Early Attempts of Laryngeal Pacing
In 1977 Zealear and Dedo introduced the concept of using electrical stimulation to restore the function of unilaterally paralyzed laryngeal muscles. They used a canine model [21], in which the cricothyroid muscle was electrically stimulated on one side. The stimulation was paced by the activation of the cricothyroid muscle on the other side.
Bergmann et al. implanted electrodes into artificially paralyzed posterior cricoarytenoid muscles (PCA) in guinea pigs, rabbits, and dogs. In all these cases, the stimulation was paced by the respiratory rhythm, detected by means of a chest belt sensor [22]. The stimulation system was chronically implanted and was found stable in 4/6 dogs for a post-implantation follow-up period of 3–11 months. In 2/6 cases over the same follow-up period, the cables broke [23].
Obert et al. showed in a canine model that it is possible to completely restore abduction in bilaterally denervated PCAs by means of unilateral implantation of a bipolar single-stranded Teflon electrode [24]. Otto et al. implanted electrodes into the artificially paralyzed PCA in a canine model and used the EMG signal of the diaphragm muscle in order to use inspiratory diaphragm contraction as stimulation trigger [25].
Zrunek et al. used a sheep model to show that long-term low-frequency direct electrical stimulation of chronically denervated PCA muscles causes a switch of the muscle fibers from type-2 (fast) to type-1 (slow) [26].
Kano and Sasaki developed a canine model in which they used a pair of platinum-tungsten coiled electrodes. The experiment was specifically designed to evaluate the biphasic stimulation of the PCA abduction. They inserted the electrode pair at a distance of 2 mm from each other and delivered biphasic, short pulse duration (2 msec) stimuli to induce PCA abduction. One of the aims of this trial was to determine the effects of the electrode material and of the stimulation parameters on the reduction of electrical charge accumulation on the electrode and consequent risks of corrosion [27].
Sanders systematically evaluated the belly structure of the canine PCA, the abduction effect of different stimulation parameters, and the effects that the electrode insertion site and orientation in respect to the PCA fibers have on the stimulation. He also evaluated the histopathologic changes after long-term stimulation [28].
What we can learn from these animal studies is that residual or recovering reinnervation of the PCA is preferable to a complete denervation in terms of laryngeal pacing. If these results turn out to be reproducible and transferrable into clinical practice, selective neurostimulation of the PCA abductor branches might be considered as the future of laryngeal pacing. The surgical procedure used to place the electrode, the electrode design, the stimulation parameters, and the characteristics of the stimulator shall be carefully adapted to this condition. In the best case, the laryngeal pacing devices should be able to synchronize the PCA stimulation with inspiration.
In 1989 Bergmann received a positive vote from the relevant ethics committee in East Germany for the first clinical trial aiming to evaluate laryngeal pacing in human. However, he did not enroll any patients because he was highly concerned about the long-term stability of the implant and because of the ongoing political changes in Germany at that time [29]. Thus, the first clinical trial was started in 1995 by Zealear in cooperation with an international team, on tracheotomized patients who suffered from BVFP, using a preexisting implantable pulse generator (Itrel II, Medtronic Inc.) with 3- or 4-channel spinal cord electrodes [30]. This proof of principle trial was the first clinical investigation to evaluate the performance of laryngeal pacing in BVFP patients. However, this preliminary study showed issues with the adopted system, e.g. electrode corrosion, and was not followed by further developments of the system or larger cohort studies.
In contrast, innovative technologies are now available that may support a more efficacious implementation of laryngeal pacing. This increases the interest of the scientific and medical community for the potential clinical applications of laryngeal pacing.
14.4 New Laryngeal Pacing Concepts
Three insights from numerous anatomical and clinical studies concerning the innervation patterns of the intrinsic larynx muscles have to be considered.
First, there is a great variability of the natural RLN branching. Between 1 and 6 terminal branches of the RLN innervate the PCA with an asymmetric pattern when the left and the right side are compared. The innervating branches of the RLN enter the PCA with different orientations and through different layers [31].
Second, the anatomic variability and prevalence of intralaryngeal nerve anastomosis (Galen’s) between the superior and recurrent laryngeal nerve and their clinical impact are not finally defined. These inconstant neural connection patterns seem to be responsible for the remaining innervation of the laryngeal muscles after RLN lesions, a finding supported by the variability of LEMG results and vocal fold positions [32].
Third, the typical synkinetic reinnervation patterns after RLN lesions have a main impact on newer laryngeal pacing concepts. In cases of BVFP with relevant vocal fold motion impairment, the PCA muscles are mainly reinnervated, but the established reinnervation is generally only partial and misdirected, often synkinetic. Thus, the vocal fold abduction is strongly impaired and asynchronic with respect to the respiration cycle, particularly because of the typical and aberrant reinnervation of abductor axons into adductor muscle fibers. It is important to note, however, that although aberrant, this type of reinnervation still enables the reinnervated muscle fibers to respond to neurostimulation.
Because BVFP is the most suitable indication for such investigational therapy, the stimulation of the terminal abductor branches of the RLN has become one of the most promising targets for laryngeal pacing. In summary, it can be assumed that due to the variability of RLN branching, intralaryngeal neural connection patterns and synkinetic reinnervation status, the position of the spots with the best response to the stimulation may differ in individual PCAs.Accordingly, when Zealear et al. mapped the total surface of chronically denervated/reinnervated canine PCA muscles with plane array multi-electrodes, they were able to identify surface spots located in the lower medial quadrant of the central region of the PCA, that, when electrically stimulated, triggered an effective abduction. The said spots are known as “hot-spots” and their distribution pattern varies from PCA to PCA [33].
In all cases, it is necessary to consider the medical procedure that is used to expose the PCA for electrode implantation. For instance, the use of open surgery may increase the risk of laryngeal immobility following scar formation. Another challenge is the electrode anchoring into the PCA and the mechanical stress on the electrode due to head and neck movement. In this regard, our animal studies showed that there is a risk of electrode dislocation depending on the procedure used to position and anchor the electrode into the PCA. Current research focuses on minimally invasive procedures for the PCA hot-spot detection and consequent electrode placement [34]. In particular, the design of the stimulation electrode should consider the effects of current distribution, electrical charge accumulation, and potential electrode corrosion in order to avoid the occurrence of the same problems observed by Zealear [30]. Furthermore, the design of the stimulator should consider the need of delivering biphasic stimulation with amplitudes, pulse length, and frequencies that support the most efficient nerve stimulation in a pathologically reinnervated PCA, potentially showing a high rate of synkinesis. Ideally, the stimulator should permit the modulation of the stimulation parameters based on the individual needs of each patient and synchronization with the respiratory cycle.
14.5 Candidates for Laryngeal Pacing
The perspective clinical use of laryngeal pacing should preferably be considered when the target PCA reinnervation, particularly favorable synkinetic reinnervation, is established and no vocal fold movement recovery occurred [35]. However, in principle, laryngeal pacing can also be used in patients who have been suffering from BVFP for more than a decade. BVFP patients who underwent a previous surgical glottis enlargement but nonetheless are unable to sustain their physical activities are potentially suitable candidates for laryngeal pacing as long as at least one vocal fold isn’t impaired in its mobility. The selection of potential candidates for laryngeal pacing in BVFP shall require the documented evidence that at least one PCA is able to respond to selective neuromuscular stimulation with vocal fold abduction. The application of laryngeal pacing should not be considered in patients suffering from pathologies that impair larynx functionality not related to the BVFP onset, such as ankylosis of the cricoarytenoid joint, tumor infiltration of the vocal fold or extensive scar formations. In the same way, laryngeal pacing is not considered suitable for the therapy of subjects suffering from respiratory problems caused by pharyngeal obstruction, laryngotracheal stenosis, asthma, pulmonary or heart failure or underlying neurologic diseases.
Future applications of a laryngeal pacemaker systems may include the implantation of such a system early upon onset of BVFP, after microsurgical selective reinnervation [36] of the larynx in BVFP or after larynx transplantation (please see Chap. 15) [37]. The aim of such an early implantation could be the transient relief from respiratory symptoms, avoiding the otherwise necessary tracheostomy, until either the spontaneous recovery of at least one vocal fold occurs or a definitive treatment can be performed. In these cases, it is important to remember that BVFP onset correlates with a gradual denervation that, in the first weeks following the paresis, is associated with a shift of the current threshold required to efficaciously stimulate the PCA towards the range used for muscle rather than nerve stimulation. This may increase the risks related to current spreading. In this indication the laryngeal pacing device would be a life support system like a heart pacemaker in complete A-V block with all the associated device failure risks.
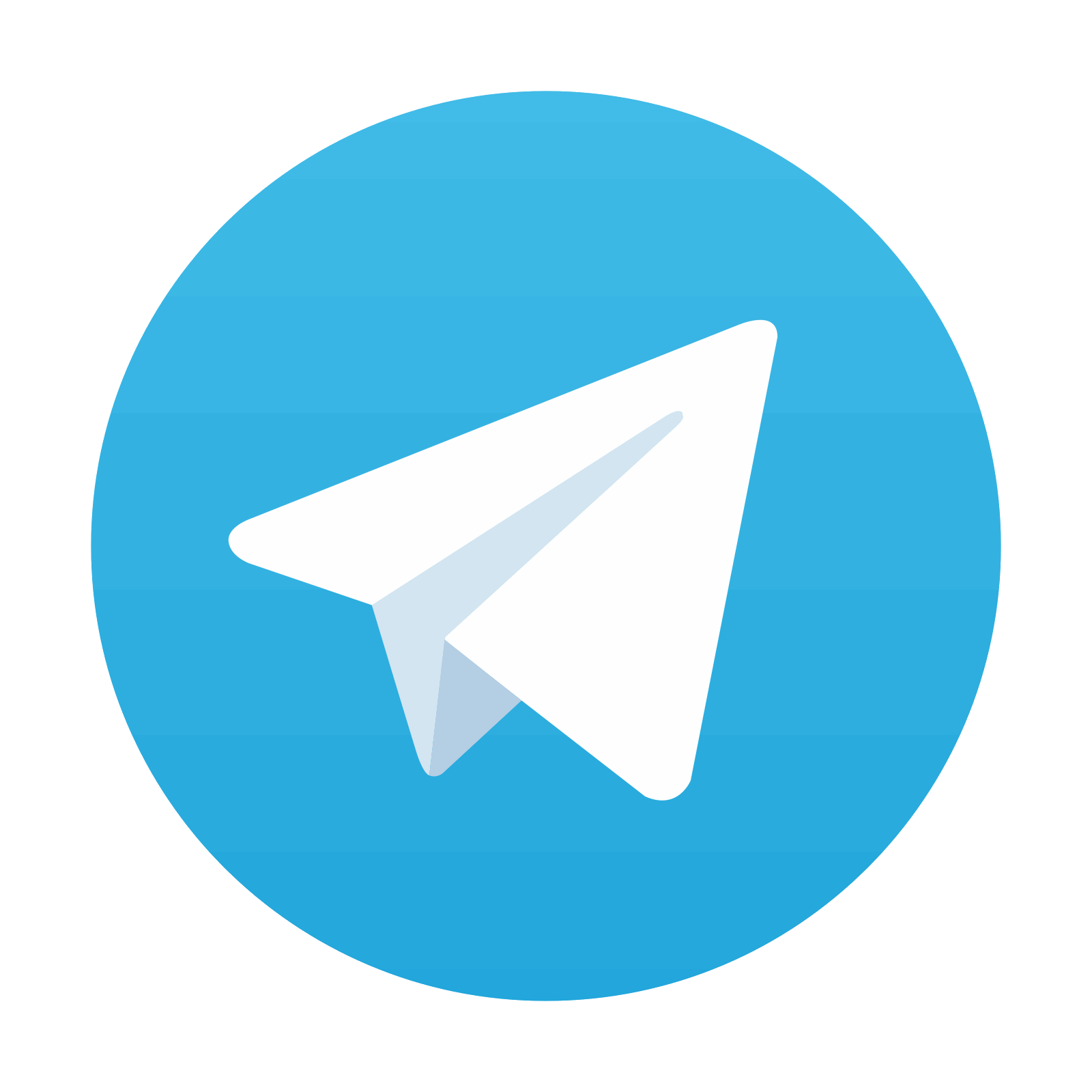
Stay updated, free articles. Join our Telegram channel

Full access? Get Clinical Tree
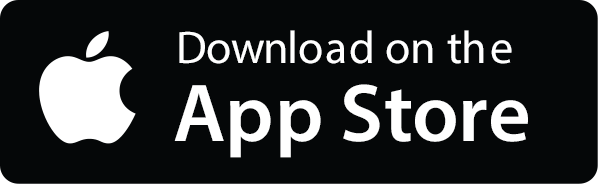
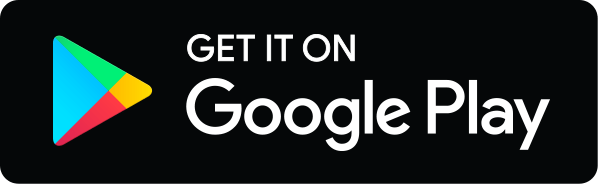