Fig. 10.1
(a) A designed porcine urinary bladder-derived ECM. An arrow indicates a flap for a vocal fold ridge. (b) A left partial hemilaryngectomy was made as indicated by an arrow. S superior, I inferior, R right, L left. (c) An asterisk indicates the implanted scaffold. (d) An intraluminal image 6 months after the operation. An arrow indicates the surgically affected site (left vocal fold). (e) A hematoxylin- and eosin-stained image indicates regenerated epithelium (asterisk), muscle tissue (M), and cartilage (arrow) at 6 months postoperatively
Recently, a decellularized laryngeal scaffold has been explored as a new material for laryngeal regeneration [36, 37]. Hou et al. [37] showed the potential for laryngeal regeneration using a chemically decellularized whole rabbit larynx. They found that the scaffold contained few intact cells and, when implanted into the omentum after recellularization with mesenchymal stem cells, vascularization was clearly seen within 4 weeks after implantation and integrated cartilage frameworks remained after 8 weeks.
Decellularized (acellular) materials are useful tools for laryngeal framework regeneration. Decreased antigenicity contributes to reducing the immune-allergic reaction, excessive inflammation and rejection of scaffolds. Biodegradable scaffolds are also ideal when considering the long-term side effects caused by implanted materials. In the future, regenerative surgeries using biodegradable scaffolds may be preferred for use in children and adolescents, once the mechanical strength of these materials has been fully established.
10.2.2 Synthetic Materials
Various studies on tracheal prostheses have been conducted since the 1960s; however, the materials used have not been ideal. Nakamura et al. [19, 20] reported successful tracheal regeneration in canine models using polypropylene-based artificial scaffolds, which were designed to be infiltrated with native recipient tissue based on the concept of in situ tissue engineering. This scaffold was composed of a cylindrically shaped polypropylene mesh framework with spongy porcine dermal collagen. Polypropylene is a commonly used plastic material. This type of polypropylene mesh has already been utilized widely in the clinical setting as a permanent and nondegradable implant for the surgical repair of abdominal herniation cases. This polypropylene mesh has benefits in terms of its high biocompatibility and morphological flexibility. The polypropylene mesh framework is covered in freeze-dried spongy collagen derived from porcine skin. This collagen is composed of 70–80 % collagen type I and the remaining 20–30 % of collagen type III. This collagen treatment is the key to better cellular attachment and sealing off from the airway after the preclotting procedure with autologous peripheral blood during surgery. This preclotting procedure can enhance the processes of tissue regeneration when bone marrow aspirates or bone marrow–derived stem cells [38] are applied to the scaffolds. Nakamura et al. [38] observed faster epithelialization and fewer complications in these types of regenerative experiments in canine trachea.
Following the successful use in tracheal regeneration, Omori et al. [26] applied this material to cricoid regeneration in a canine model. They utilized a total of nine dogs for cricoid cartilage resections (n = 5) and cricoid with cervical tracheal resections (n = 4). Two animals showed minor formations of granulation tissue and one showed exposure of the mesh framework; however, these lesions were asymptomatic. Mechanical tests of the regenerated cricoid showed equivalent mechanical strength comparable to native cricoid cartilage. Favorable luminal epithelialization of this material was observed 6 weeks after implantation. Regeneration of ciliated epithelium was confirmed by scanning electron microscopic examination.
Omori et al. [24] then applied this material to four patients in an attempt to repair the cricoid and trachea, including, in one case from 2002, a portion of the thyroid cartilage. One case of subglottic stenosis and three cases of thyroid cancer underwent in situ tissue engineering surgeries using this scaffold. During the 8–34-month observation period, every case showed a well-epithelialized airway lumen without any obstruction or major complication. Their study thus indicated that this technique could be a useful tool for laryngotracheal reconstruction, which had sometimes resulted in failure due to granulation, scar, and fibrosis. It took approximately 2 months to achieve sufficient luminal epithelialization in this study. Since the polypropylene-based scaffold is nonbiodegradable and free from growth factors, in situ tissue engineering of the cricoid and trachea may be a good indication for adult cases with stenosis and malignancies. Moreover, the adjustable mechanical power suits the need for sustaining inner airway structure, although this material cannot be utilized in children yet because of its rigid and nonbiodegradable structure.
Kanemaru et al. [39] reported the combination of this polypropylene-based scaffold in conjunction with a growth factor, basic fibroblast growth factor (b-FGF), in three clinical cases of cricotracheal stenosis after long term intratracheal intubation. Although the follow-up period only lasted up to 6 months, they showed preferable airway regeneration using the scaffold combined with the growth factor. The slow-release effect of b-FGF in combination with the surrounding spongy collagen was thought to enhance angiogenesis in the scaffold. Given the reduced possibility of tumor formation after local b-FGF application during the short postoperative period, this tissue engineering technique may be a viable approach for cases with cricotracheal stenosis.
Yamashita et al. [27] revised the polypropylene scaffold framework for the subsequent regeneration of the thyroid cartilage. Replication of the intricate luminal shape of the canine larynx was performed using a dental cast (Fig. 10.2a). Two-ply polypropylene mesh sheets with similar concavity and convexity of the vocal folds were designed (Fig. 10.2b). In preliminary studies, the sheets were coated with spongy collagen (Fig. 10.2c) and were implanted after hemilaryngectomy in their canine model; these initial attempts resulted in failure, even though the same methods were successfully used for cricoid and tracheal regeneration. This failure might be due to movements resulting from swallowing and barking as well as infection of the scaffold from secretions which are inevitable because of the anatomical location. Next, they utilized bone marrow-derived stromal cells (BSCs) as an enhancer of the regenerative process and peripheral blood for preclotting the scaffold (Fig. 10.2d) for their hemilaryngectomy model (Fig. 10.2e, f). One out of three dogs showed favorable epithelialization with preferable luminal contour (Fig. 10.2g), although no histological assessment was performed. While a beneficial contribution of BSCs was observed, it is still unknown how they behaved in the scaffold in vivo.
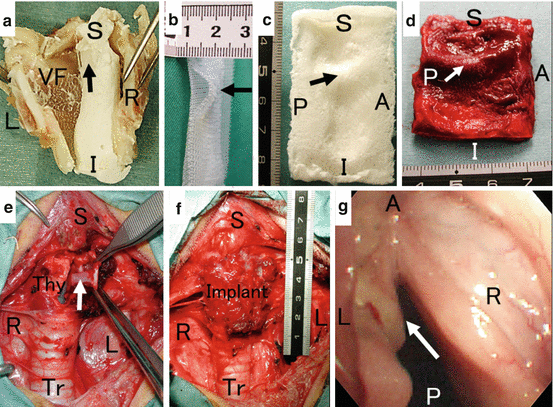
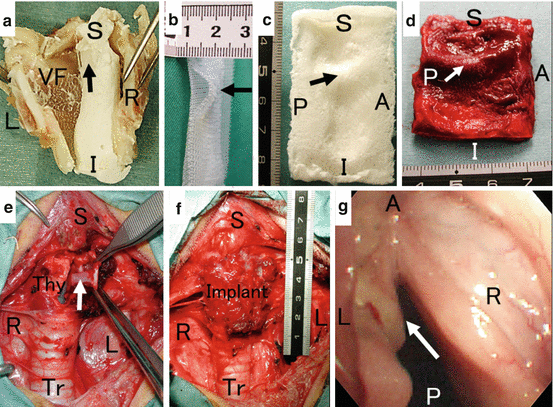
Fig. 10.2
(a) Casting procedure. The intraluminal shape of left vocal fold (VF) was reflected on the cast (arrow). S superior, I inferior, R right, L left. (b) Contours of the vocal fold were replicated on a dual-layered polypropylene scaffold (arrow). (c) An image of the scaffold covered with freeze-dried spongy collagen. P posterior, A anterior. (d) An image after preclotting procedure with BSCs and peripheral blood. (e) A left hemilaryngectomy was performed. An arrow indicates an original left vocal fold. Thy thyroid cartilage, Tr trachea. (f) The scaffold implant was anastomosed to the surgical defect. (g) A fiberscopic image taken 3 weeks after the operation. A left vocal protrusion covered with epithelium was observed
Yamashita et al. [29] reported in situ tissue engineering of the canine thyroid cartilage after a partial window defect (size of 1.2 × 0.7 cm). In the experimental group (n = 5), they utilized a single polypropylene mesh sheet with spongy collagen covered with autologous fascia lata after preclotting with peripheral blood (Fig. 10.3a–c), and in the control group (n = 3) a strap muscle flap was used for their window defect model (Fig. 10.3c, d). Four out of five dogs showed good vocal fold eminence under fiber-optic examination; typical cases showed residual tissue of the fascia covering until the 7th postoperative day and epithelialization was completed within 1 month (Fig. 10.3e). The histological data at 3 months postoperation revealed the regeneration of lined epithelium, subepithelial tissue, and muscle (Fig. 10.3f) in both groups without any new cartilage formation. Vibratory assessments from the experimental group showed suboptimal results. The protective effects from infection at early time points seemed very important for obtaining favorable regeneration.
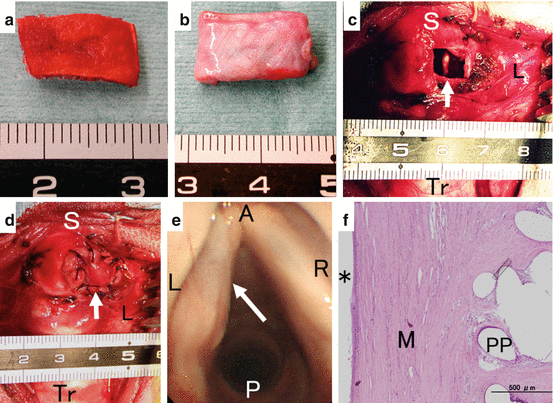
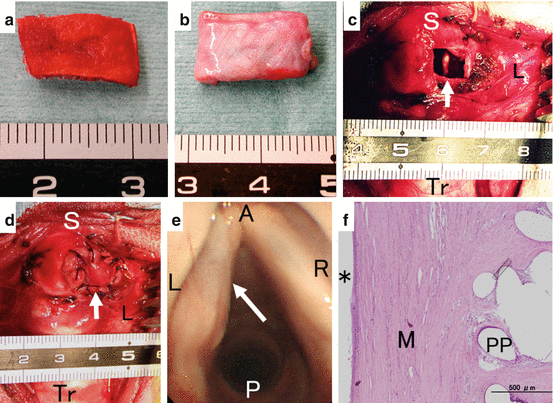
Fig. 10.3
(a) A polypropylene-based scaffold preclotted with peripheral blood. (b) The scaffold was covered with autologous fascia lata. (c) Membranous portion of left vocal fold was resected through a window defect created in a left ala of thyroid cartilage. S superior, Tr trachea, L left. (d) The scaffold implant was anastomosed to the resected site. (e) A fiberscopic image taken 3 months after the surgery. A preferable luminal shape with epithelialization was obtained at the surgical site. R right, A anterior, P posterior. (f) A hematoxylin- and eosin-stained image 3 months postoperatively. Luminal surface is covered with squamous epithelium (asterisk). Sparse muscle tissue is observed (M) between epithelium and scaffold. No inflammatory reaction is seen. PP polypropylene framework
Kitani et al. [30] reported on a canine partial hemilaryngectomy model (with a size of 1.8 × 1.0 cm) and compared the 1 % classical type (n = 6) and 3 % new type (n = 6) of spongy collagen which covers the polypropylene mesh framework (Fig. 10.4a, b). Fascia lata was also used for their artificial scaffolds (Fig. 10.4c). While the 1 % collagen model showed a half successful ratio without mesh implantation, the 3 % collagen model showed successful results in all cases after hemilaryngectomy (Fig. 10.4d–g). They stated that the 3 % collagen model contributed to tissue regeneration with harder mechanical stiffness and slower absorption than the 1 % collagen model. Histological data indicated the presence of a fine epithelial lining with subepithelial tissue without any cartilage formation in successful cases. Vibratory data were suboptimal mainly due to a difference in vertical levels of the regenerated vocal folds.
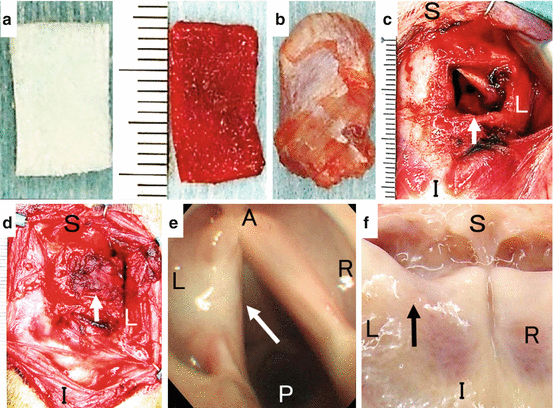
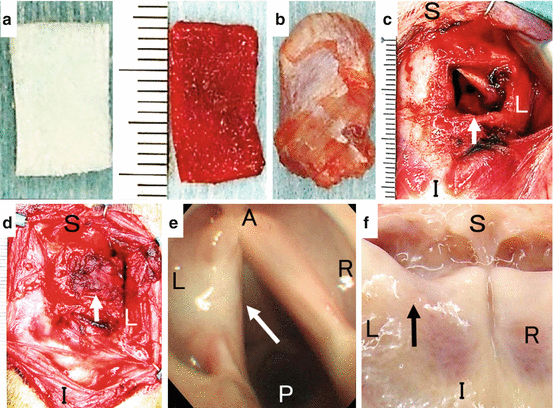
Fig. 10.4
(a) A polypropylene-based scaffold covered with spongy collagen. (b) The scaffold after preclotting procedure. (c) Autologous fascia lata was used to cover the preclotted scaffold. (d) A left partial hemilaryngectomy was performed (arrow). S superior, I inferior, L left. (e) The scaffold implant was anastomosed to the defect site. An arrow indicates the scaffold implant. (f) A fiberscopic image taken 6 months after the operation in 3 % collagen group. An arrow indicates regenerated left vocal prominence. A anterior, P posterior, R right. (g) A macroscopic intraluminal image from excised larynx revealed difference in vertical levels of vocal folds (arrow), which resulted in suboptimal vibratory data
Metallic substances have also been investigated for cricoid regeneration. Tan et al. [40] examined the efficacy of a porous metastable β-type titanium alloy for regeneration of the cricoid and trachea (20 mm in length) in 10 mongrel dogs. The titanium alloy with tiny pores (diameters of about 70–90 μm) was designed to a final porosity of 30–35 %. The final prostheses showed a cylindrical C-shape and were 0.5 mm thick, 20–22 mm in inner diameter, and 20 mm in length. Two dogs died of an accidental complication with anesthesia and pneumonia. Granulation was observed in four cases out of eight, and one showed exposure of the metastable β-type titanium alloy plate, although these animals were asymptomatic. During the 3–8-month postoperative follow-up period, all of the prostheses had completely incorporated into the host tissue and histological data showed favorable epithelial lining with simple squamous cells at the midposition and ciliated columnar cells near the anastomoses of the prostheses. The mechanical power of this material was sufficient to prevent airway collapse; however, the scaffolds had to be designed and prepared prior to the regenerative surgeries. This means that the resection area must be precisely estimated before surgery. This study showed remarkable progress in manufacturing metallic scaffolds and their potential application as bioartificial materials. As they stated in the article, further studies related to the long-term outcomes and regenerative surgeries for larger defects should be performed in the future.
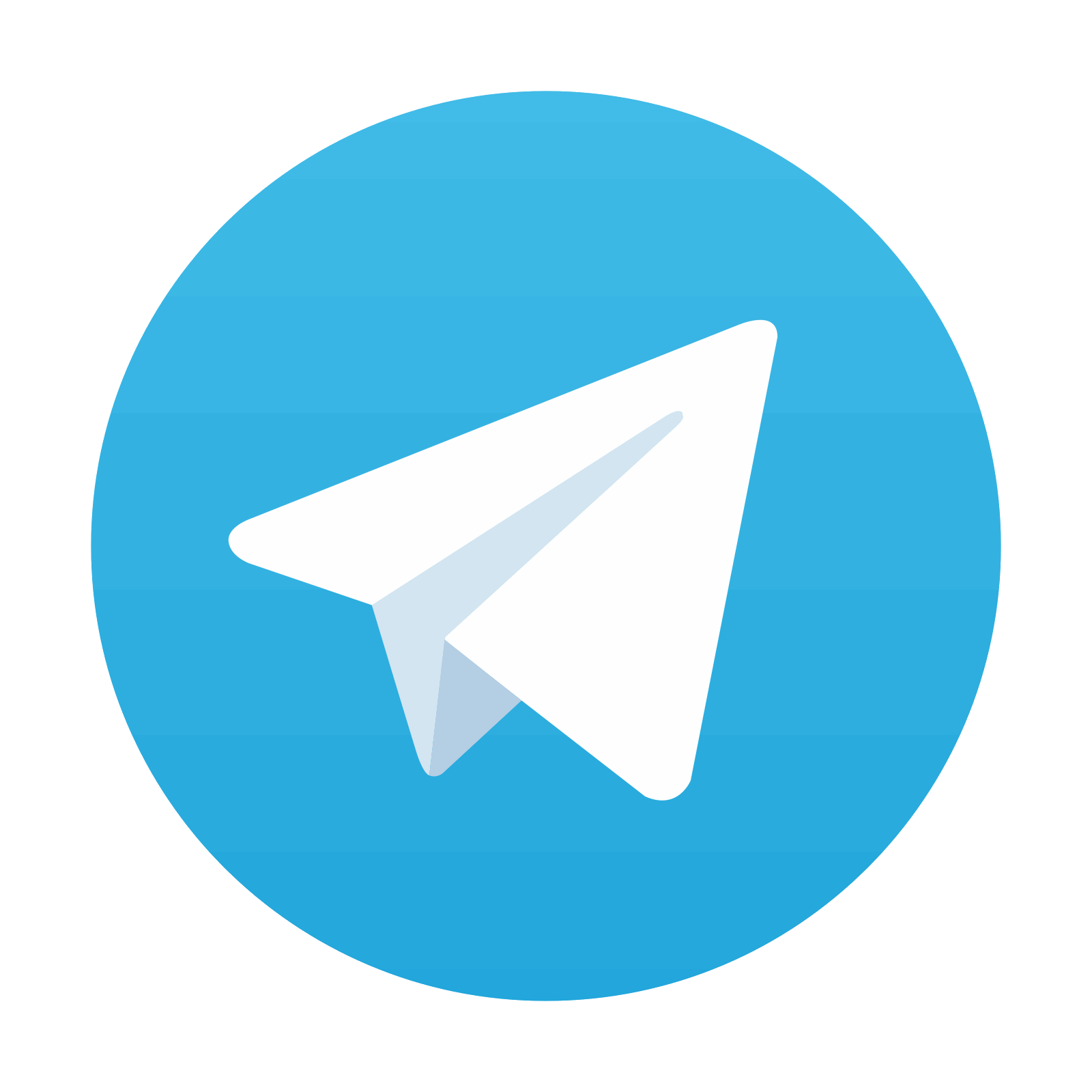
Stay updated, free articles. Join our Telegram channel

Full access? Get Clinical Tree
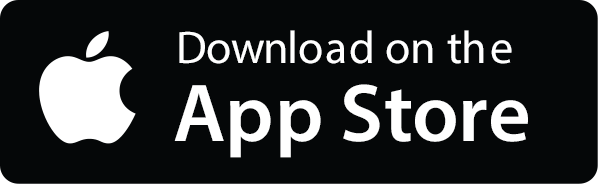
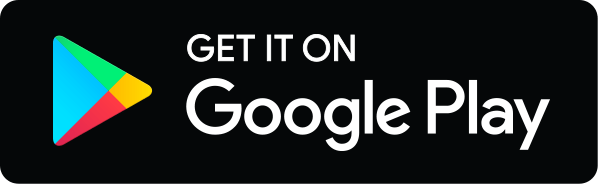