Autosomal Recessive Inheritance
If a phenotype is only manifest when both copies of an autosomal gene are abnormal, then the condition is called autosomal recessive. Females and males are affected equally. When only one copy of the gene is affected (heterozygote), an individual is considered to be a carrier, and does not develop the disease, although they may have detectable signs of the carrier state such as reduction of an enzyme level in the blood (e.g., hexosaminidase A in Tay–Sachs disease), mild physical findings (e.g., mild iris transillumination in carriers of oculocutaneous albinism), or changes detectable only by other testing (e.g., mild electroretinogram (ERG) changes in carriers of autosomal recessive retinitis pigmentosa [RP]). The phenotype of the affected patient may depend on the nature of the mutation. If the mutation on each allele is identical, then the patient is homozygous. If the mutation on each allele is different, then the patient is a compound heterozygote.
The chance for parents that are both carriers of having an affected child is 25% for each pregnancy. There is a 50% chance with each pregnancy of having an asymptomatic carrier and 25% for a noncarrier. A carrier and an affected individual both have to mate with another carrier to have an affected child. Constricted populations in which couples are knowingly or unknowingly consanguineous or members of a constricted gene pool increase the possibility of autosomal recessive conditions. Although autosomal recessive pedigrees usually show more than one affected member in the same generation (e.g., siblings), in multiply consanguineous families, the likelihood that an affected individual will mate with a carrier is higher, thus leading to the transmission from one generation to the next, a pattern called “pseudo-dominant.” Another characteristic of autosomal recessive disease in nonconsanguineous families is the wide separation of affected individuals often by many generations, as the chances of a carrier mating with another carrier is low. In nonconstricted gene pools, this chance is estimated to be less than 3%.
2.3 Autosomal Dominant Inheritance
If a condition is manifest in individuals that are heterozygotes for an autosomal gene abnormality, then the condition is autosomal dominant. Females and males are affected equally. The phenotype often appears in multiple generations, as each affected patient has a 50% risk with each pregnancy to have an affected child. Exceptions may be de novo mutations or cases in which the condition is not expressed (nonpenetrant) or is mildly expressed (variable expression) and therefore unknown. Clinically normal family members may have subclinical findings (e.g., peripheral ischemia seen only on fluorescein angiogram of autosomal dominant familial exudative vitreoretinopathy) and still transmit the full phenotype to their children. For example, neurofibromatosis type 1 is caused by heterozygous mutation in the gene NF1 on chromosome 17. An intellectually normal parent may simply have Lisch’s nodules of the iris and café au lait birthmarks of the skin, while their affected child might have these findings as well as severe developmental delay.
2.3.1 Dominant Negative Mutations
A mutation that leads to a mutant protein that disrupts the activity of the normal protein is a dominant negative mutation. This mechanism has been described in tumor suppressor genes.
2.4 X-Linked Recessive Inheritance
X-linked recessive phenotypes are not clinically manifest when there is a normal copy of the gene on one allele. In males, X-linked recessive disorders are clinically manifested because they have only a single copy of the X chromosome, and thus no normal copy of the mutated gene. Females are usually not affected by X-linked recessive conditions as they do have a normal copy of the mutated gene. It also may occur that a carrier has subtle or subclinical findings, such as electroretinogram mild scotopic alterations in a female carrier of X-linked recessive RP. If both copies of the gene are mutated, as seen in conditions where the population frequency of mutated genes is high (e.g., red–green color vision deficiency) or if there is skewed inactivation of the normal X chromosome, then a female may show a clinical phenotype.
In X-linked recessive disorders, there is no male-to-male transmission. All daughters born to an affected father will be carriers (heterozygous). A carrier female must conceive with an affected male to have an affected child. X-linked recessive disorders may also occur in males due to a de novo mutation. The pedigrees of families of X-linked recessive disease usually show unaffected heterozygous females as transmitting individuals with affected fathers and/or sons with only rare affected females.
2.5 X-Linked Dominant Inheritance
In X-linked dominant disorders, such as incontinentia pigmenti, only one copy of the mutated gene is needed to cause the disease. Therefore, heterozygous females and hemizygous males who have a single mutated gene on the X chromosome are affected. Males tend to be much more severely affected than the females and often die pre- or postnatally from the disease, as in incontinentia pigmenti. Males who survive may have XXY or other genotypes that provided them with a normal copy of the gene in addition to the mutated copy.
Each male and female offspring of affected females have a 50% risk of inheriting the condition. Like X-linked recessive, the pedigree is also characterized by not only the absence of male-to-male transmission, but also a preponderance of affected females and often a higher frequency of male fetus miscarriage.
2.6 Mitochondrial Disease
Mitochondria are organelles found in the cytoplasm of all cells. Mitochondria possess multiple copies of a circular deoxyribonucleic acid (DNA) strand that is different than nuclear DNA and encodes some of the transfer ribonucleic acid (tRNA) and proteins necessary for mitochondrial function. The primary function of mitochondria is the creation of energy for cellular metabolism. Thus, many diseases transmitted by mitochondrial inheritance affect multiple organs, especially those characterized with high-energy use such as the skeletal muscle, heart, or the eyes. Disorders of mitochondrial function may result from mutations in nuclear genes that encode proteins that are transported into the mitochondria. In this case, the inheritance pattern follows those patterns mentioned earlier. If the mutation is in a gene within the mitochondrial genome, then the typical maternal transmission pattern of mitochondrial inheritance applies. Some disorders, such as chronic progressive external ophthalmoplegia, may be inherited in more than one way.
Homoplasmy describes a cell whose copies of mitochondrial DNA (mtDNA) are all identical. Most mitochondrial mutations are heteroplasmic, meaning they only occur in some copies of mtDNA. The degree of heteroplasmy in specific tissues determines the phenotype. Only females can transmit a mitochondrial condition to offspring, because mitochondria are transmitted only from the egg. Male and female offspring are affected equally (with the unexplained exception of Leber hereditary optic neuropathy, which for unknown reasons affects males more than females). All children of females homoplasmic for a mutation will inherit the mutation. There is no male-to-male transmission. Females who are heteroplasmic for mitochondrial genome mutations will also pass them on to all of their children. However, the proportion of mutant mitochondria in the offspring, and therefore the expression and severity of disease, can vary considerably.
2.7 Other Patterns of Inheritance
2.7.1 Digenic
Monogenic inheritance refers to genetic expression of a phenotype by a single gene resulting in the patterns discussed earlier. Less frequently, the interaction of two genes is required for expression of a phenotype, which is known as digenic inheritance. In this situation, mutations in at least one copy of each gene are required for the expression of a phenotype. If the patient only has a mutation in one of the two genes, then they will be clinically normal. In RP, individuals with heterozygous ROM1 or PRPH2 mutations may be normal, but individuals who are heterozygous for both a ROM1 and a PRPH2 mutation have RP. Another example is the manifestation of Bardet–Biedl syndrome which may also occur as a result of digenic mutations (e.g., BBS2 and BBS6) or even triallelic mutations in which two mutations are found in one gene (compound heterozygote) and a third in a different gene.
Sometimes, one gene acts as a modifier of the other. In families with autosomal dominant primary glaucoma with both adult and juvenile onset, segregation of both MYOC and CYP1B1 mutations may be observed. All affected who carry the MYOC mutation have the adult onset form, and those with both MYOC and CYP1B1 mutations have the more severe juvenile presentation. Individuals carrying only a heterozygous CYP1B1 mutation are not clinically affected.
2.7.2 Polygenic
A polygenic phenotype is one that is influenced by more than one gene. Typical examples include height or skin color, which displays a continuous distribution. Many polygenic traits are also affected by the environment and are called multifactorial.
2.7.3 Multifactorial or Complex Inheritance Patterns
Many diseases and other phenotypes have multifactorial inheritance patterns. Examples include Alzheimer’s disease, heart disease, obesity, diabetes, age-related macular degeneration, or even intelligence level. These conditions are not caused by a single gene mutation, but rather are a result of interaction between genetic and environmental factors. A mutation may predispose an individual to a disease, but does not directly cause the condition. Other factors contribute to whether or not the disease develops.
Because of this complexity, multifactorial conditions are more difficult to trace through pedigrees although they do have a higher prevalence in a given family. Diseases with complex inheritance often demonstrate familial segregation because relatives of a patient are more likely to have disease-predisposing alleles in common. However, relatives may still be discordant for phenotype. An example is the lack of identical phenotypes in monozygotic twins.
The underlying mechanisms by which genetic and environment factors interact are still largely unknown and may involve direct effects on DNA by external factors or effect on noncoding RNA. Thus, counseling is based on empirical estimates of recurrence risks. The recurrence risk is higher for first-degree relatives of affected family members than for more distant relatives. In general, the recurrence risk is also increased by the presence of more than one affected patient in a family, a severe form, or an early onset of the disorder.
2.7.4 Loss of heterozygosity
If a patient has mutation in one copy of a gene that by itself does not cause disease, and then acquires a mutation of the remaining copy of that gene, the patient is said to have loss of heterozygosity (LOH) and becomes affected with the disease. This process is particularly common in tumors. Individuals with retinoblastoma who are heterozygous for an RB1 gene mutation only develop retinoblastoma when the second RB1 gene becomes abnormal in a retinal cell. LOH represents the “second hit” of the remaining allele. LOH may occur by interstitial deletion, mitotic recombination or nondisjunction. The tumors of neurofibromatosis and tuberous sclerosis are also examples of this phenomenon.
The initial abnormal gene is only transmitted to offspring if it is found in the sperm or eggs (germ line mutation) of the affected individual. The offspring would still need to acquire their own “second hits” to develop tumors. Therefore, the inheritance pattern is autosomal dominant although within the tumors, there is not a normal functioning copy of the gene.
2.7.5 Isoparental/Uniparental Disomy
This phenomenon occurs when a patient inherits both copies of all or part of a chromosome from only one parent with no contribution for that region from the other parent. Prader–Willi syndrome can result from paternal deletion or maternal uniparental disomy (UPD) of 15q11-q13, due to lack of allele inherited from the father. This region of the chromosome is imprinted (methylated) such that we only use the paternal allele. If there is no paternal allele, the disorder becomes manifest. Similarly, Angelman syndrome can happen from maternal deletion or paternal UPD of 15q11-q13, due to lack of allele inherited from the mother. Angelman locus within that region is imprinted such that we only use the maternal allele. UPD should be considered (1) in an autosomal recessive disorder with only one carrier parent (assuming correct paternity), (2) in a known syndrome with uncommon features to the disorder, (3) if the parent and patient have an autosomal recessive condition in the absence of consanguinity, and (4) in male-to-male transmission of an X-linked recessive disorder.
2.7.6 Mosaicism
When a patient has a chromosome abnormality, the abnormality is usually present in all of the cells of the body. However, if the chromosome aberration, or single gene mutation, occurs after fertilization in the developing embryo, only the cells that descend from the first abnormal cell will have the same karyotype. The individual thus has two (or more) different genetic complements. Only tissues with the aberration show signs of the clinical abnormality. This is called mosaicism. The earlier the mutation occurs in embryonic development, the more cells and tissues that will be affected. For example, although Sturge–Weber syndrome represents a somatic mosaic mutation in the GNAQ gene, which may occur late in development and thus only affect unilateral periocular skin (port wine birthmark) and the globe (glaucoma, choroidal hemangioma), if the mutation occurs early in embryogenesis, the child may have severe bilateral disease with extensive port wine birthmarks, severe developmental delays, seizures, and glaucoma. Mosaicism is confirmed by sampling more than one tissue for analysis (e.g., skin and blood).
A mosaic abnormality can only be passed to offspring if the aberration or mutation is also found in germ cells. Germ cells may also be mosaic for the change (germ line mosaicism). Although theoretically one could test sperm for the change, it is impractical to do so and eggs are inaccessible. Therefore, germ line mosaicism is implied through the pedigree. For example, if a mother has two children with a genetic disorder, by different unaffected fathers, but her blood tests negative for the mutation found in her two children, then we can presume that the mutation is in her eggs.
2.7.7 Trinucleotide repeat expansion
These conditions are defined by an expansion within the affected gene of a segment of DNA consisting of repeating units of three nucleotides in tandem. For example, the repeat unit often consists of a run of CCGs (CCGCCGCCGCCG). The number of repeats can increase from generation to generation, until the normal range is exceeded, causing a disruption of gene function. Examples are Huntington’s disease, Fragile X syndrome, spinocerebellar ataxia with retinal dystrophy, and Fuchs’ endothelial corneal dystrophy. Although the disorders are inherited in an autosomal dominant fashion, families may show anticipation, wherein the disease appears to be increasingly severe with each successive generation.
2.8 Problems
2.8.1 Case 1
A 15-year-old male with neurofibromatosis comes for genetic counseling. Clinical examination confirms the diagnosis. Family history reveals no history of consanguinity. According to the pedigree (▶ Fig. 2.1), which inheritance pattern is most likely?
Fig. 2.1 A 15-year-old male with neurofibromatosis comes for genetic counseling. Clinical examination confirms the diagnosis. Family history reveals no history of consanguinity. According to the pedigree, which inheritance pattern is most likely?
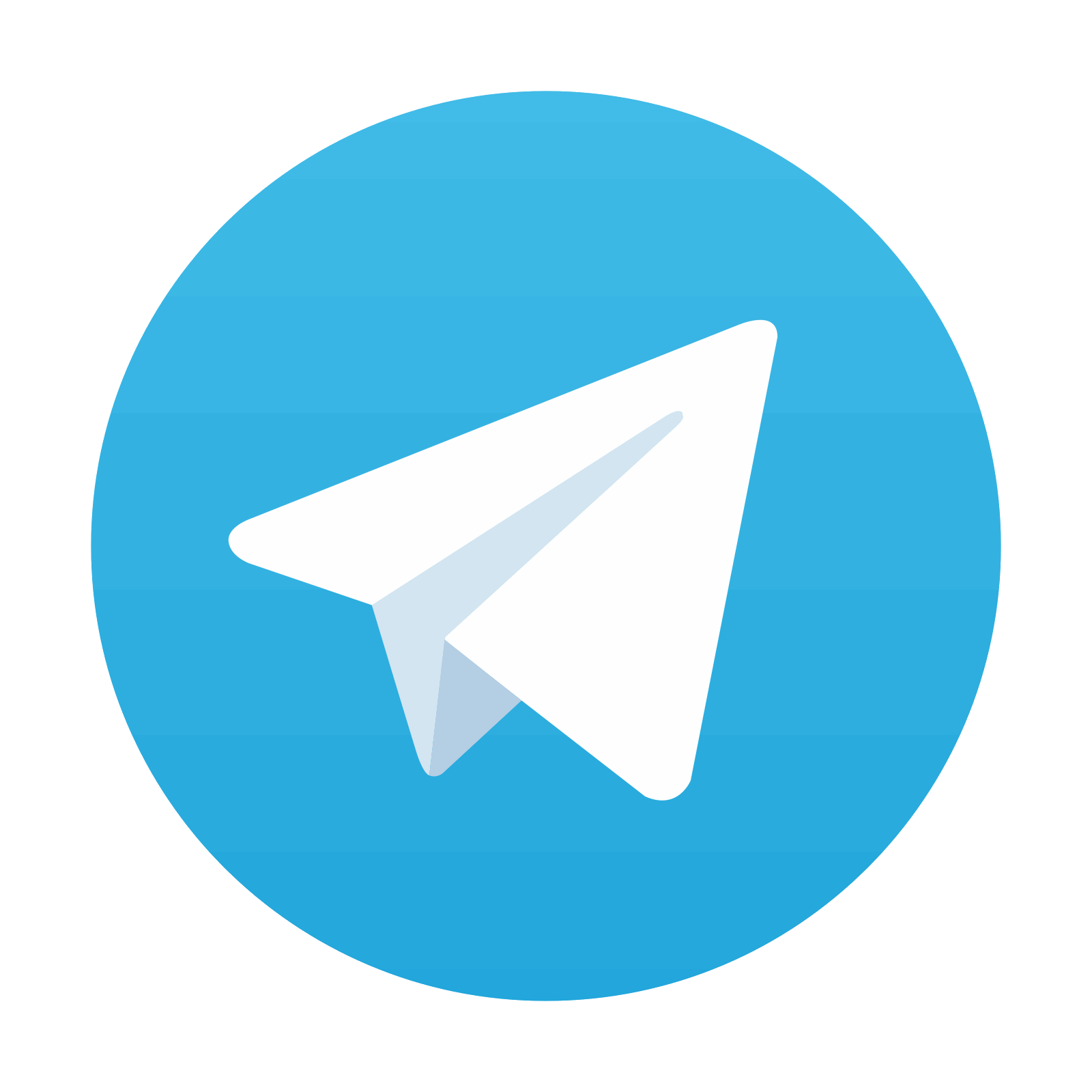
Stay updated, free articles. Join our Telegram channel

Full access? Get Clinical Tree
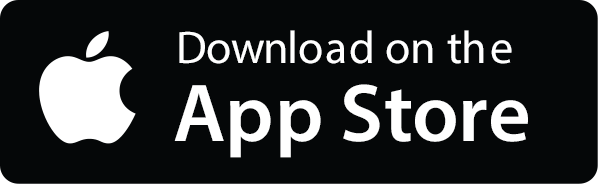
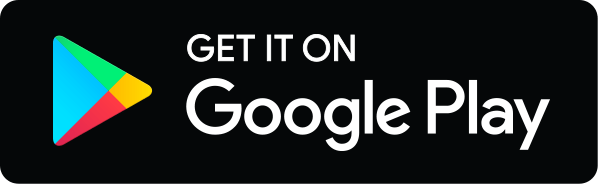