Purpose
To assess the effect of changing anterior eye refractive power with contact lenses on the transverse magnification of en face images and associated vascular indices from optical coherence tomographic angiography (OCT-A).
Design
Prospective crossover study.
Methods
Spherical soft contact lenses (–6 diopter [D] to +6 D in 2 D steps) were used to induce anterior eye refractive power changes in 11 healthy young adults and 3 × 3-mm macular scans were captured using OCT-A (Zeiss AngioPlex, software version 11.0; Cirrus HD-OCT 5000, Carl Zeiss Meditec Inc). Image transverse magnification was predicted based on refraction and biometry measurements and compared with empirical changes in the en face images measured with image analysis. Linear regression analysis was performed to assess the relationship between induced refractive ametropia and foveal avascular zone (FAZ) area, perimeter, circularity, and vessel density and perfusion density.
Results
The predicted transverse magnification was linearly related to induced refractive ametropia and to the empirical transverse magnification changes (average slope: 1.02, 95% CI: 0.90-1.34). All the OCT-A indices showed linear relationships with induced refractive ametropia ( P < .05) with the 12 D tested range altering the indices by 7% to 12%. After correcting for transverse magnification, all OCT-A indices except FAZ area were linearly related to induced refractive ametropia ( P < .05) and were reduced to 1% to 9%.
Conclusions
This study is the first to show that induced refractive ametropia can affect OCT-A image magnification and indices. These changes are clinically important and need to be considered along with biometry effects when interpreting OCT-A indices. Transverse magnification changes can affect the ability of OCT-A to precisely measure linear dimensions of blood vessels.
O ptical coherence tomographic angiography (OCT-A) is a recently developed imaging modality with great potential for research and clinical use. It is capable of producing high-resolution 3-dimensional angiograms of retinal and choroidal vasculature at various depths. Compared with conventional angiography methods, such as fluorescein fundus angiography and indocyanine green angiography, OCT-A is a more patient-friendly, noninvasive technique, because it uses motion contrast analytic methods instead of intravenous dye and requires shorter acquisition time. Previous studies have reported excellent reproducibility and repeatability of OCT-A indices in normal participants as well as in pathologic ocular conditions. Ocular factors such as age, gender, intraocular pressure, refractive error, axial length, and retinal thickness have all been shown to affect the interpretation of OCT-A indices. In general, the vessel and flow densities in the retina are reported to correlate with axial length and spherical refractive error.
Transverse magnification of retinal structures can be influenced by ocular refraction, corneal curvature, and the refractive indices and axial distances between ocular components. In optical coherence tomography (OCT), the eye geometry and optical properties can alter the transverse magnification and influence the location of measured structures. For instance, in an eye with higher transverse magnification, OCT may assess retinal nerve fiber layer (RNFL) thickness at positions closer to the optic nerve head, compared with an eye with lower magnification. Thus, transverse magnification can indirectly influence thickness measurement outputs of OCT devices. Most of the indices obtained from OCT-A, for example, foveal avascular zone (FAZ) metrics, vessel, and perfusion density, are transverse in nature, so it is most likely that these indices can vary with changes in axial length and refraction.
It is well known that most naturally occurring ametropia has a significant axial component that can significantly contribute to transverse magnification. The Littmann-Bennett formula is commonly used by researchers and clinicians to compensate for the transverse magnification changes due to axial length; however, it does have some limitations. The formula only incorporates axial length and assumes a constant (1.82 mm) for the position of the posterior principal point with respect to the cornea. It does not consider other inter-related factors such as refractive error, anterior chamber depth, and the curvature of different ocular refracting surfaces, which can all influence the magnification factor to some degree, particularly in cases of refractive surgery and corneal disease where there can be a change in refraction at the anterior plane of the eye (without changes in axial length) and in situations where high refractive errors are corrected using contact lenses. Thus, there are still refractive aspects of ametropia that are not related to axial length that should be considered. Patel and associates demonstrated that anterior segment power can influence OCT RNFL thickness measurements using contact lenses. They found that measurements of RNFL thickness artifactually decreased as contact lens power increased (–12 to +8 diopter [D]), because the change in magnification factors resulted in different sections of retina being measured. They used schematic eye calculations including anterior segment power and axial length to determine appropriate scaling factors that eliminated the magnification effect on the RNFL measures induced by changes in anterior segment power. Using a similar technique, Jung and associates examined the effects of with-the-rule astigmatism on OCT-A indices, by placing contact lenses with negative cylinders with vertical axes on their patient’s eyes. They found that some OCT-A indices including vessel and perfusion density within the entire macula diminished with increase in magnitude of cylinder, with the largest change found nasally. Such lenses will not only change the cylindrical component (ie, the J 0 vector) of the patient’s prescription but also affect the mean sphere component (ie, the M vector). There is a possibility that the changes in the OCT-A indices found were caused by the general magnification effects of the sphere change, rather than the differential magnification effects of cylinder.
In a review of 509 articles that used OCT-A, only 8% accounted for magnification in their images using axial length. We are unaware of previous studies that have considered magnification effects of other ocular parameters such as anterior segment optics or change in spherical refractive ametropia to understand their effect on the scaling of OCT-A images and associated measurements and could find no reference to it in a computerized search (in Google Scholar and PubMed databases). These factors are likely to artifactually change OCT-A indices. The current study, therefore, was designed to quantify the effect of magnification on OCT-A indices, by changing anterior eye power, and refractive error, using different spherical powered soft contact lenses. This repeated measures design allows for control of random between-subjects factors and assess the effects of anterior eye power on OCT-A indices, with fewer participants than would be required by a between-groups study. It also allows us to directly measure image magnification caused by the change in anterior eye power and assess that relationship with OCT-A indices in a controlled manner. A schematic eye model was developed to compensate for the effects of changing refractive error on transverse magnification by incorporating all the contributing ocular factors more comprehensively, especially axial length and refractive error. This model was tested against empirically measured transverse magnification changes in the images to assess its appropriateness for correcting OCT-A indices.
Methods
This was a prospective crossover single-center experimental study. A total of 11 participants aged 18-35 years were included in the study. The mean age of the participants was 26.4 ± 4.6 years, and 6 participants were female. For each participant, either the right eye (4 participants) or the left eye (7 participants) was randomly selected for testing. The participants were screened to ensure normal vision and eye health through a detailed medical and ocular history, visual acuity assessment, slit-lamp examination, intraocular pressure measurement, and fundus examination. Participants who had visual acuity worse than 6/9.5 with or without habitual correction, Van Herick grade <3, intraocular pressure >21 mm Hg, history of glaucoma, intraocular lens implants, any ocular or systemic condition, previous adverse experience with contact lens wear, or hypersensitivity to tropicamide or other dilating eye drop were excluded from the study. Participants with spherical equivalence within ±3.00 DS and astigmatism <1.50 DC were included, a range that is comfortably within the focusing range of the Zeiss OCT-A imaging system. All participants were provided with a clear explanation of the testing procedures, and written informed consent was obtained from all participants. The study was conducted in accordance with the Declaration of Helsinki and was approved by the Queensland University of Technology Human Research Ethics Committee.
Data Collection Protocol
The tested eye was dilated using a drop of 1% tropicamide. Once pupil dilation was confirmed 30 minutes after drop instillation, images were acquired using OCT-A (Zeiss AngioPlex, software, version 11.0; Cirrus HD-OCT 5000, Carl Zeiss Meditec Inc, Dublin, California, USA). Two consecutive baseline 3 × 3-mm angiography scans centered on the macula were captured in dark illumination while the participants fixated on the instrument’s internal green fixation cross, without any contact lenses present.
A series of 6 spherical soft contact lenses (1-Day ACUVUE moist, etafilcon A, base curve 8.5 mm, diameter 14.2 mm; J&J Vision Care, Jacksonville, Florida, USA) ranging between +6.00 to –6.00 D in 2.00-D steps were placed on the dilated eye to change the dioptric power of the anterior segment and induce refractive ametropia. The order of the lenses was randomized for each participant. A 5-minute lens settling time was included after the insertion of each contact lens. The contact lens was examined on-eye with slit lamp biomicroscopy to ensure adequate settling and lens fit. The refractive change induced by the contact lens was measured with an auto-refractometer (Tomey RC-800; Tomey Corp, Nayoga, Japan) each time the contact lens was changed. The induced refractive ametropia was calculated as the spherical equivalent.
Two sets of 3 × 3-mm angiography scans centered on the macula were captured with OCT-A through the dilated pupil with each contact lens in dark illumination by a single examiner. OCT-A scans were acquired with FastTrac retinal-tracking to minimize motion artifacts. Images were discarded and recaptured, if signal strength was <7, if the images had motion or segmentation artifacts in more than 10% of the image, or if the image was not centered on the fovea or exhibited image scan tilt >5 degrees to the horizontal. The en face image of the superficial retinal layer of 3 × 3-mm macular angiograms was exported in JPEG (Joint Photographic Experts Group) file format for further image analysis.
Outcome Indices
Five standard OCT-A vascular indices of the superficial retinal layer from the AngioPlex Metrix toolbox analysis were assessed: FAZ area, FAZ perimeter, FAZ circularity, vessel density, and perfusion density. The FAZ area (in square millimeters) is the area of the capillary-free zone within the macular region surrounded by interconnected capillary beds and the FAZ perimeter (in millimeters) is the length of the boundary demarcating the FAZ. FAZ circularity (unitless) quantifies how close the shape of the FAZ is to a perfect circle, with a value of 1.0 denoting a perfect circle. Vessel density (in millimeters per square millimeter) is defined as the total length of perfused vasculature per unit area in the region of measurement whereas perfusion density (%) is the area occupied by the perfused vasculature per unit area in a region of measurement. The perfusion density and vessel density for the central 1-mm macula zone (equivalent to the Early Treatment Diabetic Retinopathy Study [ETDRS] central foveal ring) of the OCT-A images were analyzed. The average of the 2 measurements of these OCT-A indices were taken for analysis.
Optical Modeling and Transverse Magnification
Ocular biometry parameters including axial length, anterior chamber depth, corneal curvature, and refractive error were measured without contact lenses on eye using the IOL master (version 5.4; Carl Zeiss Inc, Jena, Germany) and Tomey RC-800 autorefractometer and were used to model the eye and calculate transverse magnification. A 3-surface schematic eye model was developed using contact lens thickness and power, incorporating a single refracting surface for the cornea, and anterior and posterior surface for the crystalline lens. Corneal power was taken as the mean of the keratometry corneal powers in the principal meridians. The model was similar to that used by Patel and associates, except that their model assumed patients were emmetropic, and we accounted for induced ametropia and calculated transverse magnification with respect to the participant’s pupil position whereas Patel and associates referenced transverse magnification with respect to the posterior nodal point of the eye. The refractive error measured using an autorefractometer for different contact lens conditions were also adjusted for the infrared OCT measurement wavelength of 840 nm and used for further calculations.
Image Analysis and Transverse Magnification
To verify the model, the transverse magnification values were compared with changes in magnification empirically measured from the OCT-A’s exported en face images using a custom image analysis MATLAB (MathWorks, Natick, Massachusetts, USA) program. The superficial capillary plexus en face image captured without contact lens was used as baseline (reference image) and was compared to the images captured with various contact lenses. For each image, a set of minimum 3 corresponding pair-points was manually tagged by a single examiner. These manually selected points were then used to determine the scale changes and rotation changes of the image compared with the baseline image. The optical model predicted transverse magnification in microns/degree whereas the image processing measured a proportional change in transverse magnification. To compare these estimates on a common scale, the relative transverse magnification was calculated by dividing the transverse magnification values of each condition by the mean transverse magnification across all conditions for both empirical and optical model transverse magnification measures providing a unitless metric for the 2 systems.
Transverse Magnification Correction Factor
The transverse magnification values computed from the schematic eye were also used to correct magnification effects on the extracted OCT-A indices, based on the axial length assumed by AngioPlex for its analysis (ie, 24.46 mm giving a transverse magnification of 295.68 microns/degree).
The total transverse magnification correction factor was calculated for each participant and each contact lens condition using the formula:
Transversemagnificationcorrectionfactor=(Transversemagnificationpredictedwithschematiceyemodel(microns/degree))295.68microns/degree
A number of the Zeiss OCT-A indices can be corrected for transverse magnification as follows solely based on predicted scale changes.
CorrectedFAZperimeter(mm)=FAZperimeter×transversemagnificationcorrectionfactor
CorrectedFAZArea(mm2)=FAZarea×[transversemagnificationcorrectionfactor]2
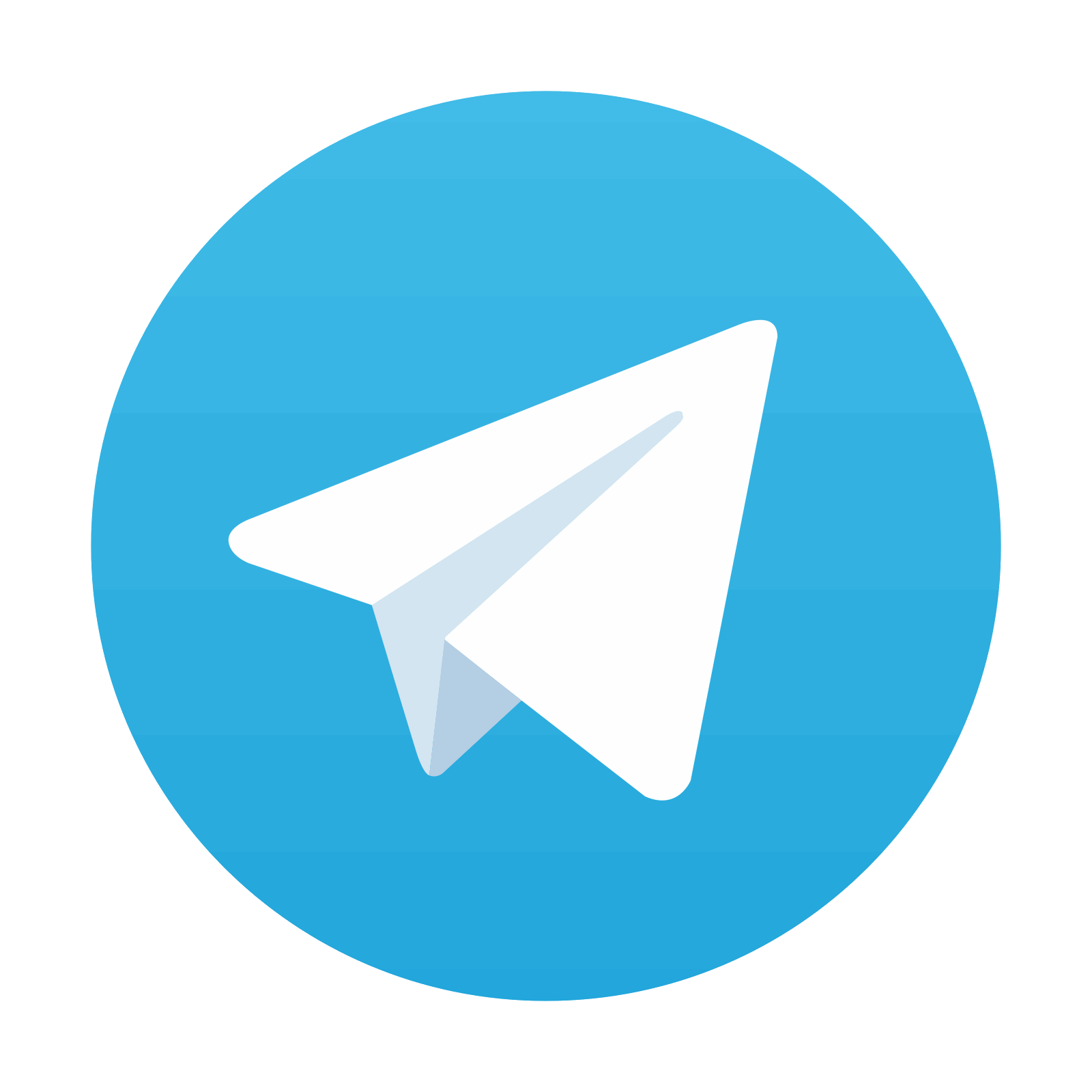
Stay updated, free articles. Join our Telegram channel

Full access? Get Clinical Tree
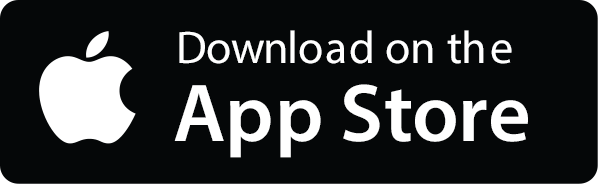
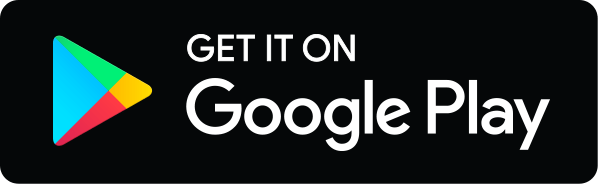
