Indocyanine Green Angiography of the Ocular Fundus
Michael D. Ober
Howard F. Fine
Louis Chang
Christina M. Klais
Lawrence A. Yannuzzi
Fluorescein angiography (FA) has revolutionized the diagnosis, classification, and management of retinal diseases. It allows visualization of the retinal circulation and the integrity of the blood retinal barrier. Although the resolution of the retina provided by FA is unparalleled, it has limited transmission through the retinal pigment epithelium (RPE), restricting its ability to image the choroidal circulation (1). Furthermore, relatively small amounts of blood, lipid, pigment, or serosanguineous fluid can significantly impair the penetration of fluorescence.
Indocyanine green (ICG) angiography functions best in several areas where FA has limited utility, such as imaging the choroidal vasculature or through hemorrhage. The recognition of the role of the choroid in retinal diseases as well as the wide availability of digital angiography has increased interest in ICG angiography. The applications for ICG angiography continue to expand, particularly in differential diagnosis, disease management, targeting of therapy, and furthering our understanding of the etiopathogenesis of various diseases.
PHYSICAL AND CHEMICAL PROPERTIES
ICG is a water-soluble tricarbocyanine dye. It contains sodium iodide (less than 5%), which increases solubility in blood. It comes supplied with an aqueous solvent which yields a solution with a pH between 5.5 and 6.5. ICG will precipitate at high concentration or when mixed in normal saline. Once mixed with the supplied solvent, it tends to decay at a rate of approximately 10% over 10 hours and should be used within this time.
ICG shows peak absorption at 605 nm and maximal fluorescence at 835 nm, which are both in the near-infrared portion of the electromagnetic spectrum. These wavelengths permit superior penetration of ocular tissues allowing detection through pigmented barriers such as the RPE and hemorrhage. ICG is rapidly and nearly completely bound to plasma proteins (98%) which help to retain the dye within vascular spaces. This property aids in delineation of the choriocapillaris, which is difficult to image with fluorescein because of rapid extravasation into the extravascular space (2).
ICG is excreted exclusively by the liver where is to secreted unaltered in bile. There is negligible renal, respiratory, or cerebrospinal uptake, and ICG does not cross the placenta (2). These properties are likely due to its strong binding to plasma proteins.
HISTORY OF MEDICAL USE
ICG was first used in medicine in 1956 to record dilution in whole blood (3). It was injected in a single bolus and samples of blood were removed at set time periods to analyze for ICG concentration. This technique was soon applied to determine cardiac output (4). Once it was established that ICG is excreted exclusively by the liver, the rate of concentration decrease was used to measure hepatic blood flood and function (5,6).
Kogure and Choromokos were the first to attempt ocular ICG angiography in dogs (7,8). After discussing their findings with David, they together performed the first ICG angiogram in monkeys (9). Later, David et al. published a discussion of the first human ICG angiogram in 1969 via an intracarotid injection (10). Flower and Hochheimer performed the first human intravenous ICG angiogram in 1972, which successfully imaged the choroidal circulation (11,12). Through the next several years, Flower, Patz, Hochheimer, and their colleagues refined the procedure and published reports detailing normal and pathological states of the choroidal circulation (12, 13, 14 and 15).
Videoangiography with ICG was introduced in 1984 by Tokoro (16) who worked along with Hayashi et al. to subsequently make adjustments to the excitation and barrier filters (17, 18 and 19). This new system provided an excellent movie format of the choroidal circulation, but individual images suffered from poor resolution and the halogen bulb risked retinal toxicity when used for extended periods. The progression to digital ICG videoangiography was pioneered by Guyer et al. who were the first to display their results immediately on a high-resolution monitor (20). Yannuzzi et al. further modified an ICG video angiographic system, by adding a synchronized flash rather than the continuous illumination in previous renditions, which reduced overall light exposure and allowed later images to be captured (21). This system was the first to show the characteristics of late phase ICG angiography which have become familiar today.
TOXICITY
ICG is a relatively safe dye and considered to have fewer adverse reactions than fluorescein (22). A classification system has been published by Hope-Ross et al., dividing adverse reactions into three categories (23). Mild reactions, which are defined as transient and resolve without treatment, occur in approximately 0.15% of patients. These include nausea, vomiting, extravasation, sneezing and pruritus. Moderate reactions occur in 0.02% and may require medical treatment although complete recovery occurs without severe sequelae. These include urticaria, syncope, fever, nerve palsy, and local tissue necrosis. Severe reactions occur in 0.05% of patients and exhibit prolonged effects requiring treatment with variable recovery including bronchospasm, laryngospasm, cardiac events, seizures, and anaphylaxis. At least three deaths attributed to ICG administration can be found in the literature (23, 24 and 25), although none of them occurred during ocular angiography.
Adverse reactions do not appear to be related to the dose injected (26). There also does not seem to be a significant correlation to previous allergies to ICG or other medications (23). There is, however, a relation to adverse reactions and renal failure (27,28). While impaired renal function should not change the excretion of ICG, patients undergoing hemodialysis may be hypersensitive due to many factors (28).
PHOTOGRAPHIC TECHNIQUE
To obtain ICG angiographic images using a digital fundus camera, typically color, red-free, and/or green-free images are taken in the area of interest. ICG angiography can be performed before or after FA because the barrier filters for each study will exclude the dye from the other. For eyes receiving combined FA/ICG angiography, the transit phase is typically shot using FA, unless identification of feeder vessels is required. When possible, images are taken immediately after injection of the dye to demonstrate the early phase of choroidal filling. Note that should the photographer wait until the dye is first noted on the alignment and focus monitor, the early phase of the study may be missed. Images are obtained in a rapid, sequential manner at approximately 1-second intervals until the retinal and choroidal circulations are at maximum brightness. There is no direct viewing of the fundus; therefore, the image exposure must be continuously adjusted by altering the flash illumination control or gain setting based upon the image displayed on a high-resolution monitor. Initially, the gain must be set high to compensate for low fluorescence levels during the early choroidal filling phase, but a reduction must be made immediately to compensate for the rapid influx of dye during the early retinal and later recirculation phases.
Once the point of maximal brightness has been captured, images are taken at 1-minute intervals until 5 minutes into the study. Thereafter, images are obtained at 3- to 5- minute intervals until satisfactory late images are available. Late ICG images are characterized by optic nerve and dark retinal vessels silhouetted against the relatively hyperfluorescent diffuse gray background of the choroid. Throughout the course of the study, the liver continuously removes ICG from circulation resulting in a gradual decrease of the fluorescence which requires a corresponding increase in the intensity of the flash illumination to maintain uniform images.
INTERPRETATION
The interpretation of ICG angiographic images is more complex than that of FA. It is based on the same principles, which include classification into areas of relative hyper- and hypo-fluorescence during specific phases of the angiogram. The early phase of the ICG angiogram shows the larger choroidal vessels individually. Over time the choriocapillaris continues to emit a diffuse hyperfluorescence while dye is removed from the intravascular circulation making the individual choroidal vessels indistinguishable. The degree of relative fluorescence in ICG is dependent on many variables, the most important of which is often the internal structure of the lesion itself, but also upon the intensity of the exciting light source, concentration of dye, the particular technique used (scanning laser ophthalmoscope versus digital fundus camera), as well as location and quality of the barrier and excitation filters.
Hyperfluorescence can occur for several reasons. Defects in the RPE yield areas of transmitted fluorescence (window defect) which are hyperfluorescent in early phases. The late phase appearance depends on the status of the choriocapillaris. When it is intact, the area becomes hyperfluorescent. In areas with choriocapillaris loss (from atrophy or other), however, hypofluorescence in the late phases predominates. Abnormal vessels are also a source of hyperfluorescence that can be distinguished from leakage, which occurs in later frames of the angiogram and increases in size and relative intensity over time. Autofluorescence can also occur, although it is much more uncommon than with FA. For example, certain blood breakdown products have fluorescent properties that overlap with ICG yielding hyperfluorescence prior to dye injection in some patients with subretinal hemorrhage.
Hypofluorescence in early phases typically occurs secondary to blocked fluorescence or vascular abnormalities. Blocked fluorescence is seen secondary to many conditions such as increased pigmentation, hemorrhage, myelination, exudation, and/or fluid, among others. Choroidal vascular abnormalities are emphasized over retinal circulatory conditions in ICG. Choriocapillaris atrophy, physiologic filling defects, choroidal ischemia, or infarctions, among others, will yield hypofluorescent areas in the early phases.
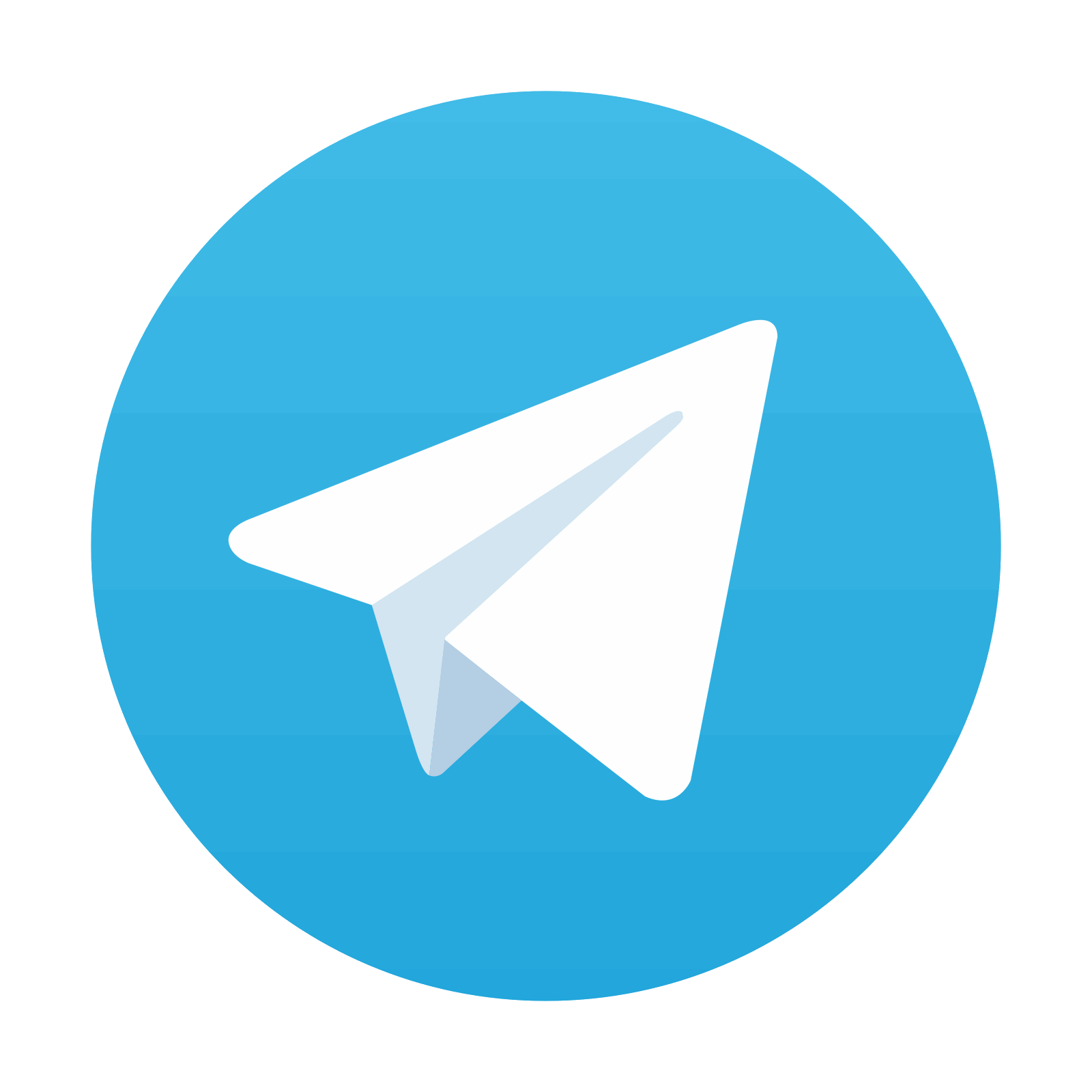
Stay updated, free articles. Join our Telegram channel

Full access? Get Clinical Tree
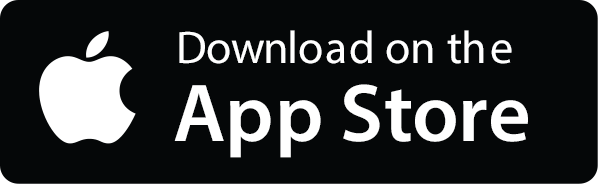
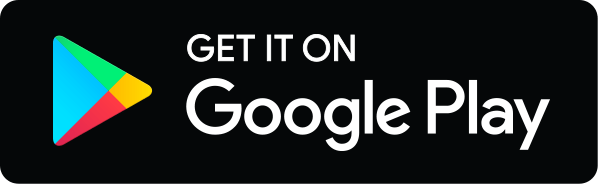