Implantation of the ossified and dysplastic cochlea presents many unique challenges to both the surgeon and programming team. Altered embryology and physiology of these labyrinthine dysplasias may result in forms and functions unfamiliar to those casually involved with cochlear implants. Remarkable developments in diagnosis, surgical technique, electrode design, processing strategies, and programming have all contributed to the ability to successfully implant patient populations previously excluded from this life-changing intervention.
Implantation of the ossified and dysplastic cochlea presents many unique challenges to both the surgeon and programming team. Altered embryology and physiology of these labyrinthine dysplasias may result in forms and functions unfamiliar to those casually involved with cochlear implants. However, with a thorough understanding of the specific issues attendant to this fascinating and varied patient population, the goal of successful implantation and performance can become a reality.
The ossified cochlea
Introduction
In the earlier days of cochlear implantation, ossification was considered a contraindication to implantation. This belief was based on concerns that even if the anatomic obstructions that preclude electrode insertion were properly addressed, the damage caused to the spiral ganglion cells by ossification would be too great to result in any significant auditory precept. However, subsequent refinements in the understanding of pathophysiology, early diagnosis, electrode design, processing strategies, and surgical techniques have allowed cochlear implantation to significantly benefit patients previously considered inoperable. In patients with partial ossification, performance may even equal those observed in patients with normal cochleae.
Pathophysiology
Ossification of the labyrinth can occur following infection via 3 main pathways: meningogenic, tympanogenic, or hematogenic. Irrespective of the pathway, a sequence of inflammatory-mediator migration, fibroblast proliferation, and ossification can begin within days and may continue for decades. Ossification itself may directly destroy spiral ganglion cells, ultimately leading to poorer outcomes in cochlear implant recipients. In these patients, auditory brainstem implants may be the only means to access auditory information.
Infectious and inflammatory mediators enter the inner ear via the internal auditory canal or cochlear aqueduct (meningogenic) or by traversing the cochlear fenestra (tympanogenic). These two most common routes both result in ossification that progresses from the base to the apex, and in the scala tympani more commonly than the scala vestibuli. Therefore, the scala tympani of the basal cochlea is the most commonly affected area of labyrinthine ossification. This fact has important clinical implications, as the degree of cochlear ossification affects both surgical technique and hearing outcomes.
Although the incidence has decreased significantly over the last 30 years, bacterial meningitis remains one of the leading causes of hearing loss in children. Severe to profound hearing loss occurs in 10% of children following bacterial meningitis. Although Streptococcus pneumoniae is more likely to result in postmeningitis hearing loss, different bacteriology has not been shown to predict the severity of ossification. Fibrosis can begin within days of infection, but can also slowly progress over years, often making diagnosis challenging. Ossification following other less common causes of cochlear ossification have been reported, including otosclerosis, chronic otitis media, trauma (including iatrogenic), labyrinthine artery occlusion, ototoxic medications, leukemia, temporal bone tumors, viral infection, Wegener granulomatosis, and autoimmune and idiopathic processes.
Evaluation
A thorough history of all patients with severe to profound hearing loss, irrespective of age, is essential to elicit processes that may lead to labyrinthine ossification. A high-resolution computed tomography (HRCT) scan is necessary to assess the preoperative bony anatomy of the temporal bone ( Fig. 1 ). However, its use alone may not provide sufficient detail regarding the degree of ossification, as a wide variance of sensitivities for identifying ossified cochlea has been reported. Attenuation of intracochlear fluid signal seen on T2-weighted magnetic resonance imaging (MRI) has proved to be a more sensitive method of assessing blockage. Similarly, contrast-enhanced T1-weighted images can detect early fibrosis and may aid the decision to implant earlier. The routine of both HRCT and T2-weighted MRI is the authors’ method of choice for all patients with suspicion of labyrinthine ossification. Despite advances in imaging, the true extent of ossification is determined intraoperatively.
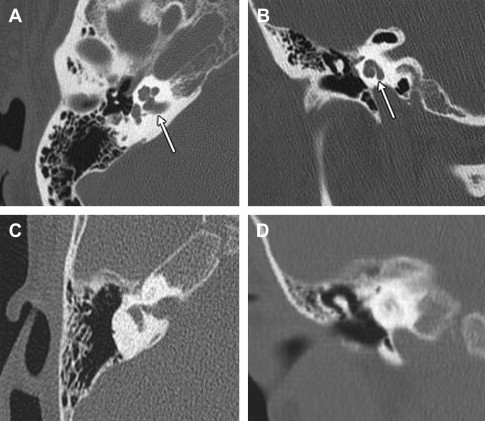
Preoperative Planning
Given the potential for extremely early onset of intracochlear fibrosis, timing of implantation becomes a critical decision. Animal studies have shown that ossification of the basal cochlea can occur within 72 hours of meningitis. Aschendorff and colleagues verified intraoperative ossification of the scala tympani 4 weeks after meningitis. Such ossification may impede full electrode insertion, leaving precious little time in which to achieve ideal insertion and outcomes. Timely identification of postmeningitic bilateral deafness may be difficult. Patient age and/or general medical condition may delay recognition. Even if recognized, the concomitant morbidities of meningitis may leave the patient as a suboptimal candidate for general anesthesia. Furthermore, the patient must be completely free of the infectious agent that caused the meningitis in the first place. Once acute infectious and neurologic sequelae are stabilized, audiologic evaluation and (if necessary) immediate amplification should be implemented. As not all patients experience rapid onset of severe or profound hearing loss, serial audiometric evaluations are performed. If hearing deteriorates to severe to profound levels, the implant team should be ready to implant swiftly, as hearing loss is likely a reflection of progressive fibrosis and ossification. This implantation should be done even if the patient is younger than 12 months. All postmeningitis patients should ideally receive their pneumococcal vaccination before implantation.
Surgical Technique
Scala tympani insertion
The goal of all cochlear implantations, including for postmeningitic patients, is atraumatic full insertion of all electrodes into the scala tympani of the basal turn ( Fig. 2 ). One hundred percent cochlear ossification leads to deafness, though not all deafness has 100% ossification. As a result, full insertion can often be achieved despite a history suggestive of labyrinthine fibrosis or ossification.
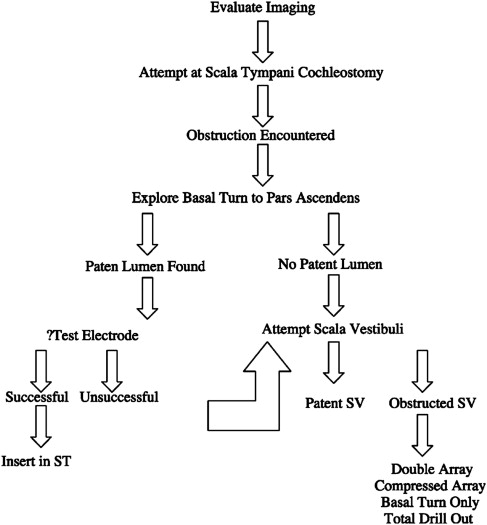
For those patients in whom full scala tympani insertion of a multichannel device is not possible, several options exist.
- •
If only limited basal cochlear ossification had occurred, drilling is continued along the basal turn until a lumen is visualized or encountered. The obstructing bone is often whiter and softer than the surrounding otic capsule, and serves as a guide to the axis of the cochlea.
- •
The basilar obstruction can also consist of uncalcified osteoid or fibrous tissue, which can be removed with picks, rasps, or drills to open into a lumen.
- •
If a patent lumen of the scala tympani is found before or at the ascending turn of the cochlea, a full insertion is performed.
- •
The judicious use of a test electrode or depth gauge can assist the surgeon with luminal patency determination, especially when the obstructive ossification or fibrosis extends beyond the lower basal turn into the pars ascendens.
Occasionally the lumen is extensively filled with hard bone, and no space can be visualized, necessitating a basal-turn drill-out procedure. Drilling with a 1-mm diamond burr is performed in the anteromedial direction for not more than 9.5 mm or until the internal carotid artery is visualized. This procedure allows for the placement of enough electrodes (7.5- to 10.5-mm drill-out) to obtain multichannel performance.
As the number of electrodes implanted generally corresponds to performance, attention has been focused on design modifications of standard, long arrays. At present, Cochlear Ltd (Sydney, Australia) offers the straight (ST) electrodes and Med-El (Innsbruck, Austria) offers the Medium and Compressed electrodes, in which the same number of electrodes are compressed into a shorter electrode array.
Scala vestibuli insertion
Early surgical techniques attempted to insert as much of a multichannel implant in the basal turn as possible, with the understanding that results might be limited. Steenerson and colleagues suggested attempted full insertion into the scala vestibuli as an alternative, though many of these patients had similar obstruction in that scala as well. This lumen is often patent in otosclerosis and postmeningitis cases, and full scala vestibuli insertion is desirable when the scala tympani is occluded. Before the introduction of the double-array device, when the scala vestibuli was obstructed the surgeon settled for a partial insertion of whichever basal scala resulted in the most intracochlear electrodes. Subsequent, basal drill-out procedures of the neo-ossified cochlea (discussed later) allowed for further, though partial insertion. Results were mixed. Cohen and Waltzman found a wide range of performance in 8 patients, and Parisier and Chute found only 2 of 5 partially implanted children with limited open-set speech understanding. More recently, Kirk and colleagues found substantial improvements in speech with both closed-set and open-set speech perceptions, similar to the majority of matched full-insertion control subjects. Rotteveel and colleagues reported no relation between speech perception and number of active electrodes (between 8 and 13) for a group of partially inserted implants.
Total cochlear drill-out
In cases of complete cochlear obliteration, total circum-modiolar drill-out has been described. In this technique the tunnel of the basal turn is created, in similar fashion to that already described. A smaller drill bit is used to enter the anterior aspect of the pars ascendens; this requires unroofing the bone overlying the distal/anterior basal turn. The abnormal white, chalky bone is encountered and followed posteriorly to the anterior edge of the oval window. Further drilling can open a channel in the inferiorly bending second turn of the cochlear, but great care must be taken not to enter the modiolus or injure the horizontal/labyrinthine facial nerve. To achieve adequate visualization, a radical mastoidectomy (with or without obliteration) can be performed. Once the receiver-stimulator is securely situated, the electrode is passed through the cochleostomy, guided into the trough, and secured with bony spicules. The entire promontory is then packed with muscle or fascia. This procedure is technically demanding, and has been abandoned in many centers in favor of double-array electrode insertion, even in cases of second-turn obliteration. Despite the use of these techniques for some patients with subjective auditory precept, there are insufficient objective long-term patient data to support total drill-out as a superior method for implantation of the totally ossified cochlea.
Double/split-array implantation
To address the possible shortcomings of partial insertion, attention was given to the design of the electrode itself. The Nucleus Double Array (Cochlear Ltd, Sydney, Australia) cochlear implant was designed for the obliterated or surgically inaccessible cochlea, and was first implanted in 1995. The double array is based on the belief that there is a positive correlation between the number of activated intracochlear electrodes and speech recognition. Similarly, two electrode arrays in different areas of the cochlea allow for a potentially greater number of spiral ganglion neurons stimulated. The number of activated intracochlear electrodes is higher than when only partial (basal) insertion is performed. Currently manufactured with the Nucleus 24 technology, the device has 11 active electrodes on each lead with two references electrodes—one on an additional electrode lead and one in the casing of the receiver/stimulator. The Med-El Corporation (Innsbruck, Austria) also markets a split-electrode array (C40 + GB), which has 5 and 7 pairs of electrode contacts, respectively ( Fig. 3 ).
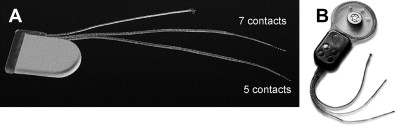
A basal array is placed in the inferior basal-turn tunnel as previously described. After the incus bar, the incus, and the stapes superstructure are removed, a second cochleostomy is placed in the second turn by drilling immediately adjacent to the anterior oval window ligament ( Fig. 4 ). Care is taken to direct the 1-mm diamond burr parallel to the tensor tympani muscle below the cochleariform process to avoid damaging the facial nerve. The drilling is performed with irrigation to avoid heating. The more apical array can be placed in either a retrograde or an anterograde orientation, if a lumen is encountered, depending on the recommendations of the manufacturer (Cochlear Ltd prefers anterograde and Med-El prefers retrograde). In a totally obstructed second turn, retrograde drilling and insertion is preferred to avoid damage to the modiolus. Fluoroscopy can serve as a valuable adjunct in the insertion of electrode array(s) in ossified cochleae.
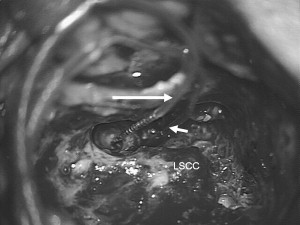
For speech performance, Lenarz and colleagues have demonstrated that both arrays of a double-array electrode lead to marked improvement compared with the basal array only, including pitch discrimination. The contribution of the apical electrodes seems to be limited when compared with the basal array, for several reasons:
- 1.
The number of active electrodes in the basal turn is greater because the basal turn is larger in diameter than in the second turn, and insertion may be less traumatic
- 2.
The density of neurons is lower at the apex than at the base
- 3.
Apical neurons lie more centrally in the modiolus than those at the base
- 4.
Many apical electrodes may need to be programmed off because of facial nerve stimulation or pain
Of note, the article by Lenarz and colleagues also reported several patients with cerebrospinal fluid (CSF) leaks, likely caused by entering the modiolus, and thus affecting performance because of spiral ganglion cell damage. Although apical electrodes provide reduced pitch discrimination than basal electrodes, they do provide important information for speech recognition. Roland and colleagues found the double array allows for more usable electrodes than the partially inserted cochlear implant, and compared with adults, children with ossified cochlea do well both in partial standard and double-array insertion.
Auditory brainstem implant
For patients with complete ossification, the decision may be made preoperatively to forgo cochlear implantation altogether in favor of auditory brainstem implant (ABI) insertion ( Fig. 5 ). Originally used in patients with bilateral vestibular schwannomas (neurofibromatosis type II), the ABI has been described with varying degrees of success for nontumor patients. Colletti and colleagues published a 10-year experience of 31 ABI patients with “altered cochlear patency” (including both labyrinthine ossification and otosclerosis), demonstrating significant improvements in open-set speech recognition. The rationale for ABI lies in the belief that even after cochlear implantation the ossification process persists and ultimately affects the remaining spiral ganglion cells. This factor may explain the decrease in performance over time of some cochlear postmeningitic cochlear implant recipients, and the poorer performance seen in adults implanted many years after the onset of meningitis-induced deafness.
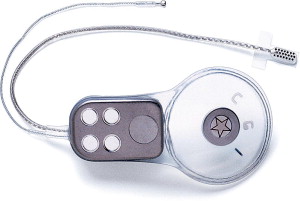
Dysplastic cochleae
Introduction
As comfort and experience with implantation in patients with normal cochleovestibular anatomy has grown, currently more and more patients with abnormal anatomy are considered as candidates for this life-changing technology. Approximately 20% of all children with sensorineural hearing loss will have associated radiologic abnormalities of the temporal bone. These abnormalities are associated with disparate clinical presentation including the severity and duration of hearing loss, and are associated with nonotologic abnormalities. In general, the more dysplastic the deformity, the worse is the hearing expected.
Nomenclature of cochleovestibular anomalies has historically confounded adequate classification. “Mondini dysplasia,” for example, had been widely and incorrectly used for 200 years to describe any inner ear malformation. Mondini’s original description detailed incomplete apical turn partitioning, enlarged vestibular aqueduct, and bulbous medial IAC. The authors caution against the use of the term “Mondini” deformity for any other inner ear anomaly.
In 1987, Jackler and colleagues proposed a classification system, facilitated by advances in computed tomography (CT) resolution, and based on the theory that different deformities were the result of arrest of specific developmental stages in inner ear embryogenesis. Though a landmark scheme adopted widely by otologists, Jackler’s classification does not completely address the possibility of multiple, isolated, independent arrests in development leading to unique phenotypes. To address this, several investigators subsequently proposed new or modified classifications systems.
- •
Phelps described a system based on the appearance of the basal turn of the cochlea, believed to correspond better with cochlear implant outcomes. Patients with abnormal cochleae, including abnormal basal turns, were grouped as “severe labyrinthine dysplasia,” whereas those with normal basal turns were grouped as “Mondini dysplasia.”
- •
Zheng and colleagues further modified this classification depending on presence (type A) or absence (type B) of the bony modiolar base. This modification attempted to correlate increased risks of intraoperative complications as a result of altered intracochlear fluid pressure dynamics.
- •
In 2002, Sennaroglu and Saatci revisited the concept of arrested development at different stages leading to specific dysplasias ( Fig. 6 ). Unlike Jackler, these investigators considered cystic cochleovestibular anomaly as a severe form of incomplete partition that occurred before differentiation into cochlear and vestibular elements.
Fig. 6
The normal cochlea and cochlear malformations. ( A ) Normal cochlea, midmodiolar section. Mo, modiolus; CA, cochlear aperture; B, basal turn; M, middle turn; A, apical turn; arrowheads, interscalar septa. ( B ) Normal cochlea, inferior section passing through the round window niche (RWN). Arrowhead, interscalar septum between middle and apical turns. ( C ) Cochlear aplasia with normal vestibule. ( D ) Cochlear aplasia with enlarged vestibule. ( E ) Common cavity. ( F ) Incomplete partition type I. ( G ) Incomplete partition type II. ( H ) Incomplete partition type III. ( I ) Cochlear hypoplasia, bud type (type I). ( J ) Cochlear hypoplasia, cystic cochlea type (type II). ( K ) Cochlear hypoplasia, with less than 2 turns (type III).
( Modified from Sennaroglu L. Cochlear implantation in inner ear malformations—a review article. Cochlear Implants International 2009; doi: 10.1002/cii.416 ; with permission.)
- •
In 2005, Papsin eloquently hypothesized several distinct anomalous pathways of development that fit well with distinct clinical presentations observed.
The difficulty seen in creating a simple, linear, and widely accepted system of classification for cochlear dysplasias underlies the current notion that such malformations are the result of more than just arrested embryogenesis. For example, certain described genetic inactivations (single and/or multiple) result in clear genotype/phenotype relationships (eg, Hmx3, Pax2). Despite significant advances, there is still much work to be done in the genetics of cochleovestibular development before complete understanding of dysmorphology can be achieved.
Evaluation
Despite controversies in nomenclature, inner ear dysplasias are readily identifiable by thin-section HRCT. As mentioned, 20% of patients with sensorineural hearing loss have osseous abnormalities of the cochleovestibular complex. However, not all patients have hearing loss severe enough to warrant cochlear implantation, and those with more severe dysplasia usually have worse hearing. Therefore, the percentage of patients who require cochlear implantation with osseous dysplasia is higher. In general, the index of suspicion of inner ear malformations should be higher in patients whose hearing loss is part of a syndrome. T2-weighted MRI can be helpful evaluating cochlear patency, and can be particularly useful in determining the presence of septations within a cavity. MRI is especially important when no reliable auditory thresholds are obtainable with behavioral/play audiometry. In these patients, the presence or absence of a cochlear nerve can be ascertained by evaluating high-quality sagittal views of the IAC in the CISS (constructive interference into steady state) or FIESTA (fast imaging with steady-state acquisition) sequences.
Surgical Technique
Patients with cochlear abnormalities were initially precluded from implantation over concerns of electrode placement, array stability, and perceived risks to surrounding structures (including facial nerve, modiolus, and carotid artery). Advances in radiologic accuracy as well as surgical technique prompted implant centers to offer implantation to those with cochlear dysplasia. Coupled with reports of beneficial outcomes of speech perception, this further bolstered support.
The type of cochlear malformation dictates the surgical approach. Nearly all cochlear dysplasias can be implanted, with the exception of complete inner ear aplasia (Michel deformity—see previous section on ABI). For the vast majority of implantable inner ear malformations, a standard transmastoid facial-posterior tympanostomy (facial recess) approach to the middle ear can be applied. The main exception to this is the common cavity malformation, which is best approached through a transmastoid labyrinthotomy.
Posterior tympanotomy
Cochlear implantation via the posterior tympanotomy can be accomplished in the vast majority of dysplastic cochleae. It is a standard approach with which otologists and those experienced in routine cochlear implantation are quite comfortable.
- •
The mastoidectomy is performed using conventional burrs.
- •
The superior and posterior cortical bony margins are not saucerized, allowing for later stable coiling of the electrode within the mastoid cavity.
- •
The short process of the incus is identified, and serves as a valuable landmark for the development of the posterior tympanotomy.
- •
The posterior bony external auditory canal is thinned.
- •
Confirmation of normal facial nerve anatomy by preoperative imagine is critical. If normal, the facial nerve is identified at the second genu and followed inferiorly while taking care to preserve a thin layer of bone over the nerve.
- •
The chorda tympani is identified anterior to the facial nerve superiorly, but joins it laterally and inferiorly in the mastoid at the annular edge, thus creating a triangle of dissection that can be followed medially until the middle ear space is encountered. Often this is facilitated by an air cell tract between the mastoid and middle ear that runs through the posterior tympanotomy.
- •
The space is widened, taking care not to injure the facial nerve, chorda tympani, incus, ear canal, or fibrous annulus of the tympanic membrane. Smaller burrs will be necessary to accomplish this, as the recess itself rarely exceeds 2 or 3 mm.
- •
With the facial recess opened, one can easily visualize many structures of the middle ear, including the round window niche. The round window niche usually lies just inferior to the stapedius tendon and oval window, though the location may vary in dysplastic cochleae.
- •
The round window niche is removed, revealing the round window membrane.
- •
Gentle palpation of the ossicles may elicit a round window reflex, which can be helpful in confirming location.
- •
The cochleostomy is made just anteroinferior to the round window annulus. Size is determined by the type of electrode array inserted.
- •
The cochleostomy is then packed with fascia to make a tight seal.
- •
Intraoperative monitoring (impedances, telemetry) is performed.
- •
The incisions are closed, keeping the electrode lead array in the confines of the mastoid cavity.
- •
Conventional radiographs or fluoroscopy confirm electrode position after closure, making sure the array has not migrated.
- •
This image then serves as a record of the position of final placement.
Transmastoid labyrinthotomy
The posterior tympanostomy is not necessary for all inner ear malformations. For the common cavity malformation, the inner ear can be accessed directly on opening of the mastoid antrum, usually obviating the need a facial recess approach and/or extended incudal fossa approach and their attendant risk of complications.
Although the transmastoid approach to the common cavity is fairly straightforward, it is the actual implantation of common cavity malformations that presents unique challenges to the surgeon. The cavity lacks a central modiolus, hence the usual goal of perimodiolar electrode positioning does not apply. Histologic studies show that many rudimentary forms of normal cochlear structures are present in a common cavity malformation. An equivalent to the organ of Corti is observed, as is a vascular equivalent to the stria vascularis. Most importantly for cochlear implantation consideration, the auditory neural tissue present lies peripherally, in the walls of the common cavity. Clusters of cell bodies (spiral ganglion cell equivalents) are present in the wall, as are many of the support cell structures. Reviews of histologic studies of malformed cochleae show that their spiral ganglion cell numbers are significantly lower than those in a normal ear. This finding has not, however, been shown to correlate with a reduced performance using a cochlear implant. It has been suggested that a minimal number of spiral ganglion cells is needed for effective electrical stimulation of the auditory nerve. Beyond this, the presence of additional spiral ganglion cell populations may not be essential for obtaining good outcomes from a cochlear implant, perhaps because the operating system of the implant is relatively simpler than the intricate functioning of the normal inner ear and its full complement of spiral ganglion cells. It is not only the number but the location of the neural elements that must be considered in common cavity cases.
Approach to the common cavity is determined based on preoperative images, and mostly can be approached directly via the mastoid antrum ( Fig. 7 ). Using continuous facial nerve monitoring, the surgery commences.
- •
Well and tie-down holes are drilled, followed by the trough and mastoidectomy.
- •
Subperiosteal pockets are made for the receiver-stimulator package and ball electrode.
- •
Mastoid bone around the cavity is removed with the drill for adequate exposure of the posterior surface of the cavity in the antrum.
- •
Drilling the cochleostomy commences (approximately 1.0–1.5 mm in diameter), attempting to keep the endosteum intact.
- •
By using intraoperative imaging (plain film, fluoroscopy, CT) to better demonstrate common cavity architecture, a site directly opposite the IAC is avoided.
- •
The authors prefer to make the cochleostomy laterally, at the inferior portion of the cavity because it bulges into the mastoid, although others have described opening through the site of the remnant of the ampullated portion of the lateral semicircular canal.
- ○
Both techniques allow for introduction of the electrode array and insertion of the full complement electrodes, although in the authors’ experience the ampullated end of the lateral canal is usually poorly formed and not easily identified.
- ○
Other surgeons advocate a canal wall–down mastoidectomy to better visualize and approach the middle and inner ear structures.
- ○
- •
These procedures are completed by obliterating the external meatus in one case and reconstructing and reinforcing the canal wall with temporal bone and temporalis muscle in the other.
- ○
It is thought that these steps may also help in preventing a subsequent prolonged CSF leak.
- ○
To stabilize the electrode within the cavity and avoid intrameatal placement, Beltrame and colleagues developed a “double posterior labyrinthotomy” technique in which a customized implant array is fed through one labyrinthotomy and manipulated/secured through a second. Manolidis and colleagues described the addition of a third labyrinthotomy that is used to endoscopically confirm placement of the electrode array. However, with fewer than 2% of all cochlear implant recipients having common cavities, insufficient data exist to support the impact of these techniques.
- ○
- •
The receiver/stimulator is placed in the well and tied down.
- •
The ground electrode (if separate) is placed under the temporalis muscle.
- •
After copious irrigation, the cochlear endosteum is opened.
- •
At the authors’ institution, fluoroscopic guidance is used to insert the electrode along the lateral wall, preventing kinking and bending, so that intrameatal (IAC) placement is avoided.
- •
Intraoperative use of CT has also been described.
- •
The electrode array can be manipulated with gentle rotation if insertion is impeded by a septation.
- •
The insertional stop point is reached when the outer wall of the cavity is covered by electrodes. Further insertion will initiate kinking or bending or fold over in the cavity, resulting in shorting of electrodes. This distortion is unnecessary and might be detrimental to performance and number of usable electrodes.
- •
The authors currently implant a straight array with concentric banded electrodes so that outer wall contact can be achieved. Newer perimodiolar electrode arrays would not appose the neuroepithelium and might affect performance.
- •
After the electrode is securely in place, the remainder of the procedure is exactly as is performed during traditional posterior tympanostomy cochlear implantation.
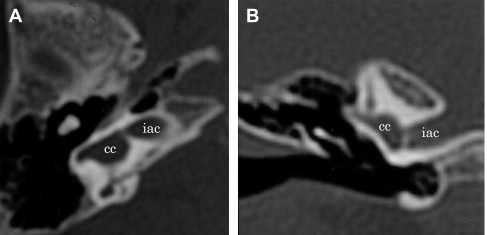
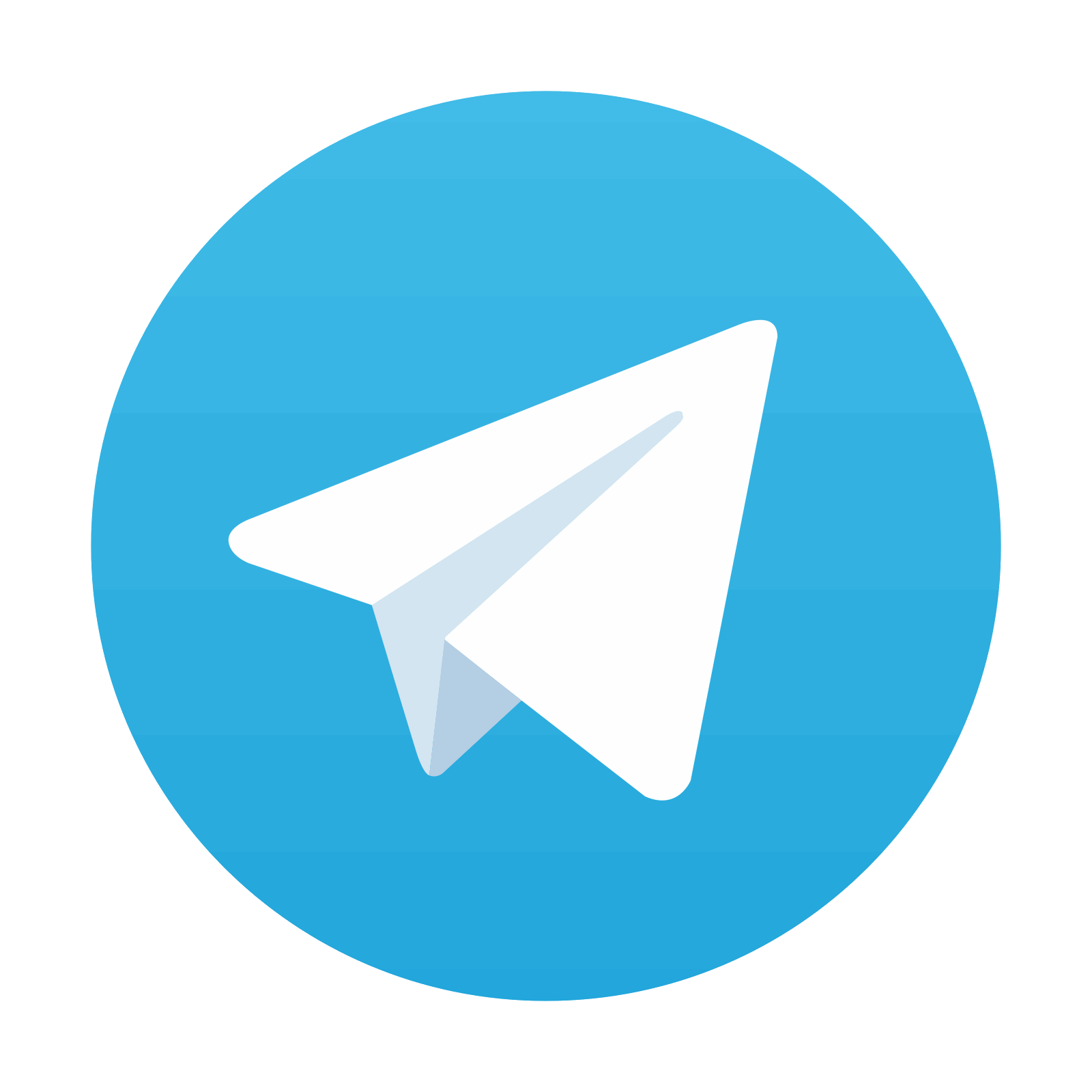
Stay updated, free articles. Join our Telegram channel

Full access? Get Clinical Tree
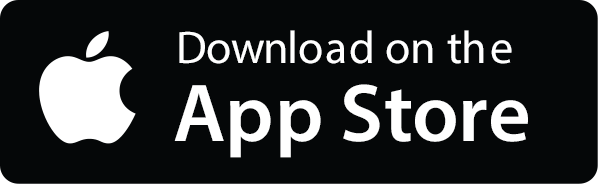
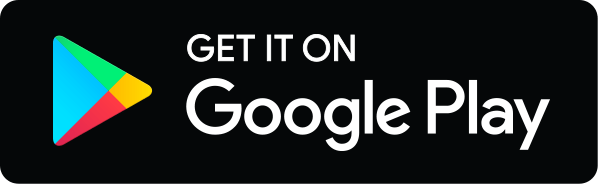
