Fig. 15.1
Coronal human Weigert stain (myelin stains black) brain slice with schematic depiction of common anatomic structures targeted for movement disorders surgical therapy, including the subthalamic nucleus (STN), globus pallidus interna (GPi), and ventral intermediate thalamus (VIM). Also depicted are neighboring structures such as the posterior limb of the internal capsule (IC) and optic tract (OT)
15.2 Deep Brain Stimulation of the Subthalamic Nucleus
Deep brain stimulation of the bilateral STN provides greater improvement in motor symptoms, motor fluctuations, and quality of life than best medical therapy in patients with PD. However, the surgical therapy does have an increased risk of adverse events [6–8]. Unilateral STN DBS also improves motor symptoms and quality of life in these patients [9–11]. Because of the growing recognition of the prevalence and severity of non-motor symptoms of Parkinson’s disease, investigators have increasingly evaluated the effects of STN DBS on sleep and other non-motor symptoms.
Several studies report changes in sleep quality and sleep architecture following bilateral STN DBS. Arnulf and colleagues evaluated 10 subjects 3–6 months after STN DBS with 2 nights of polysomnography (PSG): 1 night with the stimulator on and 1 night with the stimulator off [12]. Compared to the night with DBS off, subjects had a 47 % increase in total sleep time and a 36 % increase in sleep efficiency when DBS was on. In this study, periods of prolonged wakefulness on the DBS-off night coincided with akinesia and dystonia, which were not present during the DBS-on night. Iranzo and colleagues evaluated 11 subjects pre-surgically and 6 months following bilateral STN DBS and showed post-surgical improvement in subjective sleep quality [13]. Polysomnography showed increased nocturnal mobility, fewer arousals, and an increase in the longest period of uninterrupted sleep. In this study, however, there was no improvement in sleep efficiency and no significant difference in other measures of sleep architecture. Monaca et al. studied 10 subjects with PSG pre-surgically and 3 months after bilateral STN DBS (1 night with DBS on and 1 night with DBS off) [14]. Compared to their pre-surgical evaluation, subjects reported improved sleep quality following STN DBS and post-surgical PSG showed increased sleep duration and sleep efficiency but no change in sleep architecture based on sleep stage percentage. There were no significant differences between the pre-surgical PSG night and the post-surgical DBS off night; therefore, the authors concluded that the lesional/subthalamotomy effect associated with electrode placement was not sufficient to improve sleep in these subjects [14]. However, Merlino and colleagues reported that microsubthalamotomy improved both objective and subjective sleep in 15 subjects who underwent PSG and questionnaire evaluation 1 week before and 1 week after STN DBS before the stimulator was turned on [15]. Cicolin and colleagues investigated polysomnographic changes before and 3 months after bilateral STN DBS in five patients with Parkinson’s disease [16]. They found a significant increase in sleep efficiency and a significant reduction in latency to rapid-eye-movement (REM) sleep. They also reported trends toward increased total sleep time and time spent in slow wave sleep and REM sleep, but these did not reach significance. In each of these studies evaluating sleep architecture with PSG, there were no changes in REM Sleep Behavior Disorder or periodic limb movements of sleep before and after STN DBS [12, 16, 13, 14]. However, a recent study by Nishida and colleagues found a reduction in REM sleep without atonia [17]. They evaluated ten subjects (two with unilateral and eight with bilateral STN DBS) 1 week pre-surgically and 1 week after initial DBS programming. In addition to improvement in subjective sleep quality, PSG showed reduced wake after sleep onset, increased time spent in REM, and an increase in normal REM (atonic REM). Of the four subjects who met criteria for REM without atonia pre-surgically, three had restoration of REM atonia following surgery.
Additional studies have evaluated subjective sleep quality before and after STN DBS. Hjort and colleagues evaluated changes in subjective sleep quality using the Parkinson’s disease sleep scale (PDSS) in 10 subjects 1 month before and 3 months after bilateral STN DBS compared to changes in sleep quality in 10 control subjects who were on the waiting list for DBS [18]. The subjects who underwent surgery had a significant improvement in sleep quality post-surgically while the control group had no significant change in sleep quality over the 4-month evaluation period, and the STN DBS group had significantly better sleep quality than the control group at the conclusion of the study. Lyons and colleagues evaluated 89 subjects before and 6 months after bilateral STN DBS and followed 83 of these subjects to 12 months and 43 of the subjects to 24 months post-operatively with 2 days of patient diaries at each time point [19]. This analysis showed an improvement in subjective total sleep time following surgery that persisted at 24 months. There was no change in daytime sleepiness post-surgically compared to baseline. Zibetti et al. evaluated 36 subjects with the UPDRS part IV at baseline and 12 and 24 months after bilateral STN DBS and reported an improvement in subjective sleep quality at both time-points based on item 41 of the questionnaire [20].
Unilateral STN DBS also improves subjective sleep quality. Our group evaluated 53 patients with the Pittsburgh Sleep Quality Index at baseline and 6 months after unilateral STN DBS and showed a significant improvement in subjective sleep quality over time [21]. Subjects who underwent right STN DBS had more improvement in sleep quality than subjects who underwent surgery on the left. Chahine and colleagues evaluated 17 subjects (12 with unilateral STN DBS and 5 with bilateral STN DBS) [22]. A combined analysis of all subjects showed improved subjective sleep quality and daytime sleepiness 4 weeks post-operatively that was sustained at 6 months. The 6 subjects in this group who had restless legs syndrome pre-operatively showed subjective improvement in symptoms at 4 weeks and 6 months compared to baseline.
The cause of the improvement in sleep quality and sleep architecture following STN DBS is likely multifactorial, related to improvements in PD motor symptoms, reduction of medications, improved quality of life, and possible stimulation-induced changes in sleep physiology. Further objective study with polysomnography in larger groups of subjects may help to further clarify how STN DBS alters sleep.
Despite the multiple reports of improvement in sleep following STN DBS, a single case report describes onset of severe insomnia following bilateral STN DBS [23]. The patient had continued right-sided motor symptoms and the left STN DBS electrode was determined to be positioned in the extreme external and anterior STN and was therefore repositioned. Following repositioning, the patient’s insomnia resolved, with average total sleep time changing from approximately 4 h per night prior to repositioning to 8 h per night afterwards. The authors postulate that the malpositioned electrode disrupted inhibitory connections between the anterior hypothalamus and the upper reticular formation. This report supports the idea that DBS may directly alter sleep architecture in some circumstances.
15.3 Deep Brain Stimulation of the Globus Pallidus Internal Segment
Bilateral DBS of the GPi is also superior to best medical therapy for treatment of Parkinson’s disease based on data from a prospective randomized trial [6]. Stimulation at this target is reported to be comparable to STN DBS in terms of motor outcomes up to 36 months post-operatively [24, 25]. Although some studies have shown more motor improvement and more adverse events in subjects undergoing STN versus GPi DBS, as reviewed: [26], a recent randomized study showed greater motor improvement with STN DBS with no difference between the targets in a composite outcome of cognitive and behavioral function [27]. While STN DBS was the preferred target for Parkinson’s disease surgical therapy for a number of years, these findings have renewed interest in the use of GPi as a target for treatment of patients with moderate to advanced PD.
Despite the increased interest in GPi DBS for PD, relatively few studies have evaluated the effects of this stimulation site on sleep. One study reported pre- and post-operative quality of life assessments (Parkinson’s Disease Questionnaire-39) in 11 subjects who underwent GPi DBS (7 bilateral and 4 unilateral). In this study, 6 of 11 subjects reported an improvement in daytime sleepiness following GPi DBS [28]. Volkmann and colleagues evaluated 20 subjects before surgery and at 6 and 36 months following bilateral GPi DBS with the Sickness Impact Profile as a measure of health related quality of life [29]. They reported a significant improvement in the Sleep and Rest subdomain of the scale at 6 months post-operatively that was not sustained at 36 months. To our knowledge, there are no published reports of polysomnographic outcomes in PD patients with GPi DBS. Future studies evaluating PSG and subjective sleep quality in these patients is important, particularly considering the increasing use of this therapy in PD patients.
15.4 Deep Brain Stimulation of the Ventral Intermediate Nucleus of the Thalamus (VIM)
DBS of the VIM was explored as an alternative to thalamotomy for the treatment of tremor in patients with Parkinson’s disease. While it is effective for tremor control in patients with PD and essential tremor, VIM DBS does not provide marked improvement in bradykinesia, rigidity, or dyskinesias in Parkinson’s disease [30]. Therefore, with the exception of select patients with tremor predominant Parkinson’s disease, thalamic stimulation has largely been abandoned in favor of use of STN or GPi DBS. The proximity of the VIM to the reticular nucleus of the thalamus, where sleep spindles are generated, prompted Arnulf and colleagues to investigate for changes in sleep architecture following VIM DBS in six subjects (four with Parkinson’s disease and two with essential tremor) [31]. They evaluated subjects 3–6 months post-operatively with 2 nights of PSG, 1 night with the stimulator at therapeutic settings and 1 night with the stimulator off. At high frequency stimulation (135–185 Hz), there were no changes in sleep architecture or sleep spindles compared to DBS off. In an unsuccessful attempt to induce sleep, the authors also evaluated subjects while awake during low frequency VIM stimulation (1 s bursts of 15 Hz or 30 s trains of 5 Hz) [31].
15.5 Deep Brain Stimulation of the Pedunculopontine Nucleus (PPN)
Due to its role in locomotion, the PPN has been explored as a DBS target in patients with Parkinson’s disease who have significant gait disorder refractory to other therapies. Initial studies demonstrated that stereotactic placement of PPN electrodes is safe and that there may be some improvement in gait with bilateral PPN stimulation [32, 33]. Subsequent studies have shown mixed results and have not definitively demonstrated improvement in motor outcomes from stimulation of this novel target. Stefani and colleagues reported motor outcomes in six subjects with bilateral STN and PPN DBS [34], and simultaneous stimulation of PPN and STN produced some degree of additive motor improvement, but was not significantly better than STN DBS alone. Because PPN stimulation was not superior to STN DBS in this group of patients, the authors recommend PPN DBS only as a supplemental therapy to STN DBS [34]. In contrast, Moro and colleagues evaluated six subjects with unilateral PPN DBS and found no significant change in the UPDRS part III. Subjects did report a subjective improvement in falls in the open label phase of the study [35]. Ferraye and colleagues studied six subjects who had previously undergone bilateral STN DBS and evaluated the effects of bilateral PPN DBS. They reported a reduction in falls related to freezing in the off-medication state without significant changes in other gait measures [36]. Khan and colleagues studied seven subjects who underwent bilateral caudal zona incerta (cZI) and PPN DBS with evaluations prior to surgery and 1 year post-operatively [37]. In the on medication state, PPN DBS alone, cZI stimulation alone, or simultaneous stimulation of both targets resulted in statistically significant improvement in motor outcomes compared to baseline, and the combination stimulation was significantly more effective than either target alone [37]. In summary, published reports suggest that PPN DBS may improve balance when used in combination with STN stimulation in the on medication state. However, PPN studies to date have evaluated small numbers of subjects with different surgical approaches (unilateral versus bilateral) and in the context of parallel stimulation at other targets. Further study is needed to determine the benefit of this therapy for gait dysfunction in PD.
In addition to its function in locomotion, the PPN plays a critical role in regulation of behavioral state and sleep. For this reason, several groups have investigated the impact of PPN DBS on sleep in patients with PD. Romigi and colleagues reported polysomnography in one subject before surgery and after bilateral STN and PPN DBS [38]. PSG was performed post-operatively with STN DBS alone and PPN DBS alone. STN stimulation improved sleep efficiency compared to the pre-surgical PSG, but did not alter REM sleep. In contrast, PPN DBS increased the percentage of REM sleep and reduced REM latency. Sleep questionnaires from this patient and four others with bilateral PPN and STN DBS [39] in multiple conditions (PPN and STN on; PPN off and STN on; and PPN-cyclic on (on at night) and STN on) were evaluated at 3 months and 1 year post-operatively. At 3 months, the subjects had improvement in sleep quality based on the Parkinson’s disease sleep scale (PDSS) in all three stimulation conditions. Evaluation of individual items from the PDSS suggested more improvement in insomnia when PPN stimulation (either continuous or cyclic) compared to STN stimulation alone. PPN cyclic on also caused further improvement in nocturnal restlessness and daytime dozing compared to the other two stimulation conditions. At 1-year follow-up, the improvement in sleep quality was maintained and subjects also had improvement in daytime sleepiness as measured by the Epworth sleepiness scale [39]. The authors also reported PSG recordings in two of these five subjects (one previously reported [38]) and found reduced REM latency and increase REM sleep [39].
Lim and colleagues investigated the effect of unilateral PPN DBS on sleep in five subjects (three with Parkinson’s disease and two with progressive supranuclear palsy) [40]. Polysomnography was performed with PPN DBS on during the first night and with DBS off during the second night. PPN DBS resulted in higher percentage of REM sleep (14 %) compared to DBS off (8 %). REM time was also higher during the PPN DBS on night. PPN DBS did not induce any significant change in total sleep time, non-REM sleep, or wake after sleep onset. Interestingly, two of the five subjects had REM Sleep Behavior Disorder, and REM without atonia was unchanged with PPN DBS on versus off in these subjects [40].
In addition to these reports on the nocturnal effects of PPN DBS on sleep, another group has observed profound effects of PPN DBS on wake-time vigilance. Arnulf and colleagues initiated an investigation using a cross-over, double-blind design after observing that two patients with STN and PPN DBS fell asleep during routine programming of the PPN stimulator when high-frequency stimulation was applied [41]. During the daytime, the two subjects were evaluated with polysomnography for at least 5 min at each parameter: stimulation off, right, left, or bilateral at high frequency or low frequency with 3-min washout between each setting. At low frequency stimulation bilaterally or on either side, both subjects remained alert, but at high frequency stimulation, both subjects reported drowsiness and entered non-REM sleep, predominantly stage N1 and rarely stage N2. Interestingly, in one of the two subjects, abrupt cessation of low frequency PPN stimulation induced REM sleep on five different occasions [41].
In summary, DBS of the PPN likely alters arousal and sleep in complex ways and thus provides a unique opportunity to investigate the effects of stimulation at this site on sleep architecture. The distinct outcomes from high and low frequency PPN stimulation observed in these case reports suggests that altering the stimulation pattern in the PPN region can yield markedly different behavioral states. Further investigation may provide additional clues to the role of the PPN and its connections in both normal sleep architecture and sleep dysfunction in patients with Parkinson’s disease.
15.6 Lesional Therapies for Parkinson’s Disease
Deep brain stimulation became preferred over lesional therapies for Parkinson’s disease because it is reversible, adjustable, and less likely to be associated with speech and bulbar dysfunction when performed bilaterally [42]. Despite this, lesion therapy is still performed commonly worldwide, and there is new interest in focused ultrasound for movement disorders [43]. In this context, review of the available literature on the effects of lesion therapies on sleep and vigilance may provide insights into sleep dysfunction in this patient population. An early case report documented induction of profound insomnia following bilateral thalamotomy for Parkinson’s disease. The subject had baseline insomnia that was relieved by initial left thalamotomy. However, 2 years later following subsequent right thalamotomy, he developed severe insomnia and was observed in the hospital with no sleep for more than 96 h. Although he eventually was able to sleep again, he continued to have difficulty with insomnia after discharge [44]. A more recent case report documented a similar outcome following simultaneous left VIM DBS and right VIM radiofrequency ablation. The patient developed severe insomnia following surgery that was not relieved by turning DBS off or by adjusting DBS parameters. The sleep changes were therefore attributed to lesion effects. The patient reported improvement in insomnia over time, but PSG recording 16 months post-operatively showed continued poor sleep efficiency, prolonged sleep latency, reduced sleep time, and abnormal sleep architecture [45]. Interestingly, the report by Bricolo also mentions anecdotal experience by the author that other patients undergoing bilateral thalamotomy often experience sleep disruption in the immediate post-operative period, but that these symptoms usually resolve quickly [44].
Roth and colleagues investigated the effects of bilateral stereotactic pallido-thalamic tractotomy in three subjects with Parkinson’s disease with PSG before and after the surgery. The patients had a significant increase in total sleep time and sleep efficiency and a reduction in latency to REM sleep [46]. The authors propose that the alterations in sleep could be related to improved motor symptoms or post-surgical reduction of medications. Favre and colleagues reported subjective changes in motor and non-motor symptoms based on questionnaires before and after unilateral or simultaneous bilateral pallidotomy in 44 patients [47]. One of the parameters evaluated was sleep, with 59 % of patients reporting improvement in sleep following unilateral pallidotomy and 47 % of patients reporting improvement in sleep following simultaneous bilateral pallidotomy. It is unclear if these changes were related to improvement in motor symptoms, altered medications, or lesional effects on sleep architecture.
15.7 Other Surgical Therapies for Parkinson’s Disease
In addition to DBS and lesional therapies for Parkinson’s disease, another surgical therapy includes placement of an intrajejunal tube to administer continuous drug delivery with levodopa/carbidopa intestinal gel (LCIG) [48]. In addition to its influence on motor symptoms, the effects of this therapy on sleep quality and other non-motor symptoms have also been investigated. In an open label assessment, 22 subjects who received levodopa/carbidopa intrajejunal infusion were evaluated at baseline and after 6 months with the non-motor symptoms scale (NMSS) as the primary outcome measure. Additionally, 13 subjects were evaluated with the Parkinson’s disease sleep scale (PDSS). The results showed a significant improvement in several domains of NMSS, including the sleep/fatigue domain and a significant improvement in the PDSS [49]. In another open label evaluation, 14 subjects were evaluated with the NMSS and the PDSS at baseline and at an average of 24 months after beginning therapy with LCIG. This study also showed a significant improvement in PDSS and a trend toward improvement in the sleep/fatigue domain of the NMSS [50]. Zibetti and colleagues report an additional open label evaluation of 12 subjects at baseline and 2–4 months after initiation of LCIG [51]. They report a 30 % improvement in subjective sleep symptoms and a 31 % improvement in subjective daytime sleepiness. The improvement in subjective sleep quality in these three studies is likely multifactorial, related to improved motor symptoms, altered dopaminergic medications, placebo effect, or other causes. As more patients undergo this therapy, controlled trials with more subjects and with objective PSG evaluation of sleep architecture should help to further elucidate these changes.
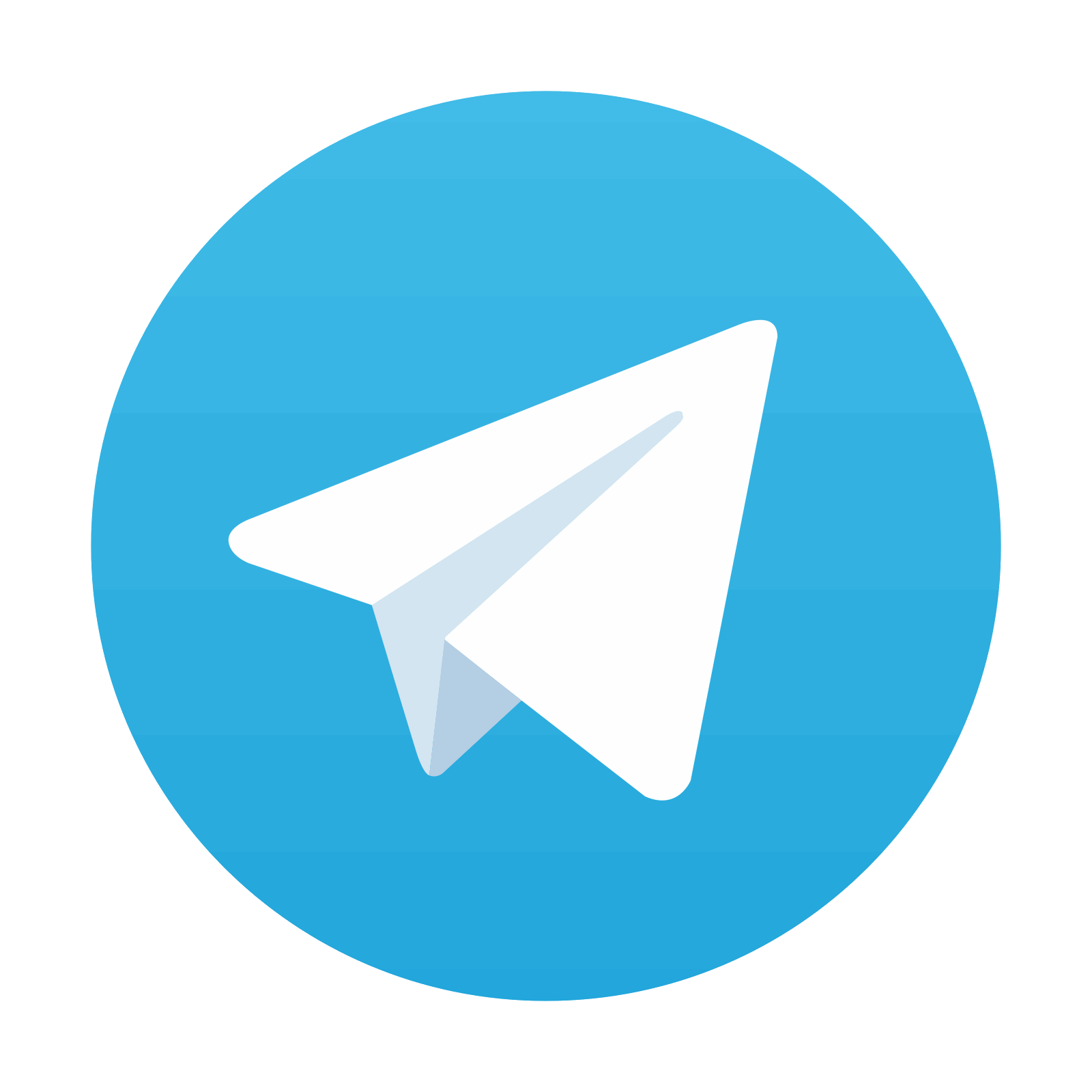
Stay updated, free articles. Join our Telegram channel

Full access? Get Clinical Tree
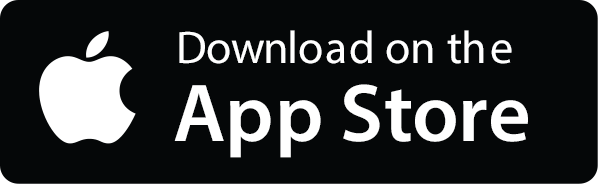
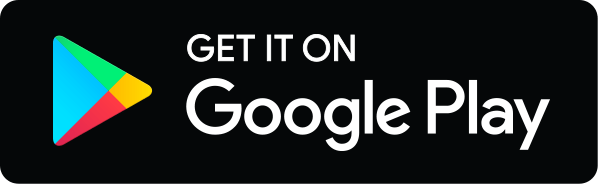