Immunology and the Nose: From Basic Science to Clinical
Summary
The ultimate dream of any scientist or physician is likely to be the discovery of the single factor that is responsible for his or her favorite disease: the one gene to rule them all. Here we discuss the approach to this goal, but we also provide a reality check to show that there might not be such a single factor. Traditionally, the field of immunology is described first by its many components, the different cell types and their specific functions, and the distinction between general defense mechanisms (innate immunity) and specific defense mechanisms (adaptive immunity) against pathogens. In this chapter, we take a more clinical approach and follow the body′s attempts to defend against external and internal threats, then investigate what happens when things go wrong.
Introduction
Immunology is a complex field that deals with a simple question: Who am I? To take this question out of the realm of esotery and into science, we can rephrase it as “How can we recognize what is a threat to our existence?” To resolve this issue, our body needs to be able to deal with a variety of potential pathogenic organisms lurking in the outside environment when they invade. In such circumstances, the body needs to distinguish between “them” and “self” to remove only the potential pathogen and not cause damage to the entire system. Yet this same system should be able to deal with aberrant cells that are due, for instance, to some mutagenic process.
Dangers Lurking in the Outside World
As an organism, we are forced to interact with the outside world, and our airways, gut, and skin are the most important sites where such interactions occur. Without taking anything away from the importance of the skin, it does have a relative easy task. Yes, in the skin we get the tactile and thermal impressions of the environment, but as these can be remotely sensed by specialized nerve endings, the skin in its most simple form can be seen as a passive layer that will hold potential pathogens at bay by acting as a physical barrier. To a certain extent this is also true for the airways and gut. The epithelial lining of these organs do offer physical protection just like the skin, but this protection is hampered by the requirements of these organs to allow physical interaction. These interactions include the uptake of oxygen in the lungs, the uptake of nutrients in the gut, and the detection of odors and pheromones in the nose. However, even considering the specific differences between these organs, when the physical barrier has been breached, all these sites need to be able to defend against the potential dangers lurking in the outside world.
The Nose: A Multifunctional Organ
We have alluded to some of the functions of the nose. For further details regarding the anatomy of the nose, its role in heating, humidification, and filtering of air before it reaches the lungs, and different aspects of the sense of smell, we refer the reader to Chapters 1 and 2.
Here we would like to describe the ultrastructural organization of the nasal mucosa, with Fig. 4.1 depicting schematic and real-life overviews. Most of the outer lining of the nose consists of pseudostratified ciliated epithelium, as the distribution of the nuclei of individual epi thelial cells gives a stratified appearance, but each cell is directly connected to the basal membrane. At least three types of epithelial cells can be detected: ciliated cells, mucus-producing goblet cells, and basal epithelial cells. In the layer beneath the basal membrane, or lamina propria, we find the vascular elements of the nasal mucosa with arteries/veins, capillaries, and sinusoids. The latter structures are expandable/contractible blood vessels that control swelling of the nasal mucosa, or the nasal cycle (see Chapter 2), thanks to smooth muscle actin-positive fibroblasts that align these structures. Also considered part of the vascular structure are the efferent vessels of the lymphatic system, through which tissue-resident inflammatory cells would be able to reach the draining lymph nodes of a given section of mucosa. The final components found in the lamina propria are the glandular structures that produce and secrete mucus into the nasal lumen through efferent ducts. The epithelium can take on different forms, depending on the site within the airways. For instance, at the head of the inferior/medial turbinate we encounter squamous epithelium, where this outer lining adds to a better physical protection from airborne particles that may impact these areas upon inhalation.
Protecting Us from the Environment
It is not easy for microorganisms to gain entrance to our body via the airways. Most of the outer lining of the nose is protected by a mucus layer that is able to trap particulate matter and microorganisms. The mucus layer is comprised of an upper gel-like phase of higher viscosity and a lower more fluid sol-like phase. The forward stroke of the cilia extends into the gel phase, moving the mucus layer through well-defined patterns at 20 beats per second out of the sinuses and the nasal cavity toward the oropharynx, where it is ultimately swallowed and digested in the stomach. During the return stroke, the cilia move within the more fluid sol phase close to the cell surface, where they encounter little drag and resistance, after which the cilia are poised again for a new coordinated forward stroke. The importance of this mucociliary clearance in maintaining homeostasis becomes clear in several situations where ciliary function is hampered, for example, in diseases such as primary and secondary ciliary dyskinesia and cystic fibrosis and by pollutants (e.g., nitrogen oxides and sulfur) and smoking.
On the microbial side we should consider that bacteria will try to colonize the nasal cavity despite the physical forces that try to evict them. The very structures relevant for clearance (the cilia) can be targeted for direct binding by bacteria, bacterial products may inhibit cilia mobility, or bacteria may initiate biofilms by forming an extracellular matrix that provides a stable and well-shielded platform for the bacteria. The formation of biofilms also has a direct clinical consequence, as bacteria in biofilms are less susceptible to the action of antibiotics. The observed link between the presence of biofilms and the underlying damage to tissue remains a point of debate. Although the link can be detected in diseases such as chronic rhinosinusitis (CRS), it is not clear whether the damage to local tissue is the consequence of the biofilm or whether biofilms are more readily formed when local tissue is damaged.
The physical or passive aspect of protection from the environment is not the whole story, as the nasal cavity also could be considered a site of active protection. On the one hand, we can detect small biologically active peptides that are able to inhibit the growth of micro-organisms (defensins); furthermore, even inflammatory cells are observed in the nasal cavity. For macrophages and eosinophils, this might not come as a surprise, as these cells would be able to deal with potential threats by opsonization (macrophages) or the secretion of toxic compounds (eosinophils). On the other hand, T lymphocytes and dendritic cells can be detected in the nasal cavity, and these cells would not necessarily have a direct role in killing potential threats. At the moment it is not clear whether all inflammatory cells within the nasal cavity have an active role in the detection and/or eradication of potential threats or whether these cells could have been discarded from the local tissue into the lumen.
Examples
Primary Ciliary Dyskinesia
Primary ciliary dyskinesia is an inherited disorder with specific ultrastructural defects that affect ciliary motion, thereby impairing mucus clearance. Previously referred to as Kartagener syndrome in 1935 and immotile cilia syndrome in 1976, the name change in 1981 reflecting the fact that cilia are not necessarily immotile, although their movement may not be rhythmic; only 50% of cases are associated with situs inversus totalis. Continuous rhinorrhea in the first 3 to 6 months of life is typical for this disease and makes this diagnosis more likely.
Secondary Ciliary Dyskinesia
Much more important for our daily practice is secondary ciliary dyskinesia, the result of environmental, infectious, or inflammatory stimuli that disrupt normal ciliary motility or coordination. During CRS, the ciliary beat frequency is often decreased or absent, which contributes to the symptomatology of the disease. The ciliary beat frequency can be greatly enhanced by rinsing with saline, resulting in significant clinical improvements.




Cystic Fibrosis
In cystic fibrosis, due to mutations in the gene CFTR (cystic fibrosis transmembrane conductance regulator), the physical aspect of the mucus changes. The rheological defect of the secretion hampers mucociliary clearance, leading to local inflammation and reduced clearance of bacteria.
Sensing the Environment
With a multitude of potential threats in the environment, detection is a complicated issue. On one hand, we would like a high degree of specificity, but this could come at a high price when the immune system is faced by new, emerging threats. On the other hand, if we only had a generic detection system, we would need to make sure that we would be able to discriminate between “them” and “us” or “harmful” versus “harmless” threats. In classical textbooks, the immune system is divided into the innate and the adaptive immune response, two branches that together cover these challenges in the detection of potential threats. The innate immune system comprises cells that use general characteristics of microorganisms for their detection. Typical cells belonging to the innate immune system are macrophages that deal with potential threats by engulfing these microorganisms and breaking them down. Traditionally, the innate immune system is seen as a simple first line of defense that allows the adaptive immune system some extra time to produce immunoglobulins and trigger a more targeted defense mechanism based on the specificity of the immunoglobulin. Today the innate immune system has grown more complex, with many aspects of the additive immune system also reflected in the innate immune system, as we will discuss later. For now we want to point out that epithelial cells, being at the interface of the outside and inside world, play an active role as part of the innate immune system.
Note
The distinction between innate and adaptive immune response is largely for the benefit of the professional, as both systems work hand in hand to fight off potential threats. However, they do this in different ways, with the innate response acting in a pathogen-nonspecific manner and the adaptive response acting in a pathogen-specific manner.
Given the challenge of detecting a multitude of different microorganisms, a central role has been attributed to pattern recognition receptors (PRRs) on the cell surface of innate immunity cells.1 As the name implies, these receptors recognize commonalities of micro-organisms (pathogen-associated molecular patterns [PAMPs]) rather than specific species. The archetype of these PPRs is the toll-like receptor (TLR). This group of receptors in humans has 10 members that, depending on the specific receptor, can be found on the cell surface or within the endosome compartment. On the cell surface, we find receptors specific for bacterial PAMPs, such as TLR-4, which is activated by the bacterial cell wall component lipopolysaccharide (LPS), and TLR-5, which is activated by the protein flagellin (an essential part of the propulsion system in some bacteria), as well as other bacterial cell wall components that are activated by heterodimers of TLR-1/TLR-6 and TLR-2/TLR-6. The TLRs of the endosomal compartment are activated by the genetic material (deoxyribonucleic acid [DNA] or ribonucleic acid [RNA]) of microorganisms. The most prominent receptors here are TLR-3, which is activated by double-stranded RNA found in some viruses; TLR-7, which is activated by single-stranded RNA; and TLR-9, which is activated by CpG (cytosine–guanine linked by phosphodiester bonds)–containing DNA. Interestingly, the CpG sequence is relatively depleted in eukaryotic DNA, making this sequence prokaryote specific. Ligation of the TLR family of receptors, through several signaling cascades, leads to activation of the NF-κB (nuclear factor kappa-light-chain-enhancer of activated B cells), activator protein 1 (AP-1), and interferon regulatory factor (IRF) families of transcription factors, which in turn switch on a broad panel of proinflammatory mediators.
This principle can also be found in the NLR (NOD-like receptor) family of receptors (nucleotide oligomerization domain [NOD] and leucine-rich) that partly derive their name from distinct functional elements within the receptors. These receptors, which are all found within the cytoplasm, have the same leucine-rich repeat as can be found in the “extracellular” part of the TLRs. These elements are required for the detection of ligands, and as such it is probably not a coincidence that one of the NLR family members, IPAF (interleukin [IL]-1β-converting enzyme protease-activating factor, also known as NLRC4), is stimulated by flagellin, whereas other members, such as NOD-2 and NALP-1, can be activated by the bacterial cell wall component muramyl dipeptide (MDP). Signaling of these receptor members is diverse and involves NF-κB for NOD-1 and NOD-2, with similar effects as described for the TLRs, and the recruitment of the caspase family of proteases for the other members. The intracellular caspases play an important role in the control of apoptosis and the conversion of pro-IL-1 to its active form.
Caution
Although pattern recognition receptors form an essential part of the innate immune response, they are present on and in many cell types, including those of the adaptive immune response. This makes the distinction between innate and adaptive immune responses less clear.
The role of proteases as signaling molecules is probably best known as part of the mechanism of subsequent protein activation leading to blood clotting. However, extra-cellular proteases are frequently observed in relationship to microorganisms and as part of allergenic mixtures. A specialized class of protease-activated receptors (PARs) has an ingenious way of detecting proteases. Within the extracellular domain of these receptors is a cryptic domain that can act as a ligand for the receptor. Just upstream of this domain is a combination of amino acids that can function as a target for some proteases. After cleavage by the protease, the cryptic domain becomes available for binding to the ligand pocket of the receptor, leading to activation of the signaling cascade associated with the receptor. A final example of PARs are receptors that are able to recognize specific sugar moieties on proteins derived from microorganisms.
Relevance of Pattern Recognition Receptors for Disease
Mechanisms and mutations in genes associated with PRR signaling emerge in different diseases, showing the relevance of these signaling cascades in health and disease. Point mutations in the protease inhibitor SPINK5 (serine protease inhibitor Kazal-type 5) cause Nether-ton syndrome, and mutations can be linked to eczema and asthma. Furthermore, mutations in the protease inhibitor ADAM33 are associated with increased risk of asthma. Point mutations in the cytoplasmic NOD receptor are associated with Crohn′s disease, and TLR-3 and TLR-4 signaling is linked with allergy. TLR-3 is a likely candidate for why viruses can induce asthma exacerbations, whereas the TLR-4 ligand LPS, depending on its concentration, is associated with either the prevention of the development of asthma (at low concentrations, hygiene hypothesis) or the induction of exacerbations (at high concentrations). A novel mechanism by which these receptors can affect disease is related to molecular mimicry. For example, it has been shown that one of the major allergens of house dust mite Der-p2 is a functional homologue of MD2, an important coactivator of TLR-4. In gut and airway epithelia that respond poorly to LPS due to the absence of MD2, the presence of the house dust mite can be detected indirectly to the facilitation of LPS signaling due to Der-p2. It is not clear, however, whether epithelial cells use this mechanism to really detect the house dust mite or that the mite would use this mechanism to create beneficial conditions, as in both cases the house dust mite seems an unlikely candidate for colonization of the nose. On the other hand, it is also hard to imagine that this mechanism is there to induce or prevent allergy.
The Adaptive Immune Response and Its Relationship to the Innate Immune Response
The contrast between the adaptive and innate immune responses can best be explained in this way: The adaptive immune response deals with a specific attack on potential pathogens that have breached the barrier function of epithelial surfaces. It involves many different cell types with complex interactions, but the whole cascade starts with a specialized cell type that surveys peripheral tissues. This dendritic cell owes its name to the dendritic extensions that originally led to its misclassification as a nerve cell. When this cell type encounters a pathogen in peripheral tissues, it opsonizes the pathogen through the endocytic pathway and generates small peptides from its proteins that are presented on the cell surface in the context of major histocompatibility complex (MHC) class 2. After migration to the draining lymph node via the lymphatic systems, these peptides on MHC class 2 are probed by the resident naive CD4 T lymphocytes via their T cell receptor (TCRs). Only those T lymphocytes with TCRs that are able to recognize the presented peptides on MHC class 2 will start to expand and differentiate into T helper lymphocytes. In the second phase of this humoral part of the adaptive immune response, these activated T helper lymphocytes activate B lymphocytes that present the same or very similar pathogen-derived peptide on their MHC class 2 molecules. As loading of B cell MHC class 2 is initiated by immunoglobulin-mediated uptake of pathogens that have been produced at a low level by the B lymphocyte itself, this mechanism ensures that only B cells that produce pathogen-specific immunoglobulin become activated. The antibodies produced by these plasma cells deal with the pathogen in distinct fashions depending on the subtype of immunoglobulin produced by the plasma cell. First, binding of immunoglobulin G (IgG) to free pathogen prevents the infection of other host cells. Second, binding of IgG or immunoglobulin M (IgM) to antigens on the cell surface of microorganisms (or IgG to small circulating antigens) can lead to complement activation. This blood-borne system, through a succession of protease-activated steps, starts by binding of complement factor C1q to the Fc parts of IgG and IgM. This cross-linking induces the formation of immune complexes with antigens, immunoglobulins, and several successive complement factors, the last one being C3b. This C3b complement factor can bind to CR1 molecules on erythrocytes, solubilizing these immune complexes and aiding their removal by macrophages in the liver and kidneys. Patients with defects in the complement system may not clear these complexes, leading to vascular deposition of these complexes and endothelial damage. Third, antibodies may deal with potential threats through binding of microorganism-specific immunoglobulin E (IgE) to the surface of mast cells/basophils or eosinophils. These effector cells upon binding secrete toxic mediators in the vicinity of the pathogen that lead to its ultimate destruction.
Note
There are five classes of immunoglobulins or antibodies in the blood: IgG, IgA, IgM, IgD, and IgE. The most abundant class is IgG, followed by IgM and IgA. IgD is much lower, and IgE is present in only minute amounts in the blood. Of these immunoglobulin classes, it is primarily IgM and IgG that protect the body internally from infection. IgA antibodies are transported in secretions to mucosal surfaces and play a major role in protecting these surfaces from infection.
The adaptive immune response also has a cellular component that starts when dendritic cells are infected by pathogens or when they opsonize other infected cells. Under these conditions, small pathogen-derived peptides are presented on MHC class 1 that are probed by naive CD8 T lymphocytes. This activation process induces CD8 cytotoxic T lymphocytes that after migration to the periphery are able to recognize and kill infected cells, as these cells, just like the infected dendritic cells, present pathogen-derived peptides on MHC class 1 on their surface.
At least two links between the adaptive and innate immune responses exist. First, the cells of the adaptive immune response also carry typical innate PRRs, so that it would be able to be co-stimulated by generic pathogen signals. Second, the dendritic cells in the periphery that take up the pathogen would do so in a local environment that is created by the innate immune response. Although dendritic cells patrol peripheral tissues, they are able to detect potential threats even when they have not yet penetrated into the tissue. In gut epithelia, it has been shown that tissue-resident dendritic cells can extend dendrites into the lumen, which would allow them to sample the environment for potential threats. The mechanism and the effect on the innate immune response would trigger or preactivate dendritic cells for the appropriate response.
Dendritic Cells and T Lymphocytes: The CD4 Helper and Effector Story
From the short description of the immune response provided above, it should be clear that the correct response is important to prevent us from becoming diseased. Depending on the potential threat, different parts of the immune system need to be activated. In this section we focus on T helper lymphocytes, which traditionally are linked with the humoral immune response. Until recently the T helper family had only two members: T helper 1 (Th1) and T helper 2 (Th2). The Th1 response was held co-responsible (see the discussion on the cellular CD8 response below) for the eradication of viruses and bacteria by triggering the production of IgG, whereas the Th2 response was responsible for the eradication of parasites through the triggering of IgE.
The Complex World of T Helper Subsets
Although in general terms this concept has not changed, it has grown much more complex over the last few years (and is expected to grow even more complex in the coming years). The reason for this increased complexity is the growing number of different T helper lymphocytes that have been identified. In addition to Th1 and Th2, we are now aware of Th9, Th17, Th22, Tr1, and T-reg helper T lymphocytes. Each of these T helper classes seems specific for the activation of defined parts of the immune response ( Fig. 4.2a ). These parts can be related to the eradication of intracellular pathogens (Th1), extracellular pathogens (Th2, Th9), and fungi (Th17), through the activation of B cells (Th1, Th2), eosinophils (Th2, Th9), neutrophils (Th17), epithelial cells (Th17, Th22), or the suppression of immune responses (T-reg and Tr1). Each of these T helper cells secretes cytokines and expresses cell surface molecules specific for these tasks. For the individual T helper subclasses, these would be TNF-α (tumor necrosis factor-alpha) and IFN-γ (interferon-gamma, Th1), IL-4, IL-5, IL-9, IL-10, and IL-13 (Th2), IL-9 and IL-10 (Th9), IL-17 (Th17), IL-22 (Th22), IL-10 (Tr1), and TGF-β (transforming growth factor-beta, T-reg). Although, all these T helper subsets seem to be under the developmental control of the specific transcription factors T-bet (Th1), GATA-3 (Th2), cMAF (Th9), RORc (Th17), and Foxp3 (T-reg), there is a high degree of plasticity, and transdifferentiation of these subsets may occur.
The driving force behind this differentiation pattern of the T helper cells is the dendritic cell.2–4 Three specific signals derived from the dendritic cell are important to guide naive T cells into the different directions of T helper subsets ( Fig. 4.2b ). The first signal is the presentation of the antigen in the context of MHC class 2 to the T cell receptor. This signal would normally ensure that the T cell response is specific for a given antigen or epitope. However, a group of proteins from certain pathogens are able to bypass this antigen-specific interaction between dendritic and T cells. Collectively, these proteins are known as superantigens. The best known examples are the superantigens produced by Staphylococcus aureus. This organism is thought to contribute to the pathophysiology of atopic dermatitis and nasal polyposis by its ability to induce polyclonal activation of the humoral immune response. The second signal is a collection of activating or inhibiting surface molecules on dendritic cells that interact with matching receptors on T lymphocytes. The most common of these are co-stimulatory CD (cluster of differentiation) 80, CD83, and CD86 on dendritic cells that are recognized by T cell–activating CD28 or inhibiting CTLA-4 (cytotoxic T lymphocyte antigen 4) receptors, CD40 recognized by CD40L, and the inhibitory PD-L1/PD-L2 (programmed cell death 1 ligand 1/ligand 2) molecules recognized by T cell–borne receptor PD-1. The third signal covers specific cytokines produced by dendritic cells. Depending on the pathogen and local tissue environment encountered by the dendritic cells, different sets of cytokines are produced that trigger the differentiation of the naive T lymphocyte into different T helper subsets.5–7


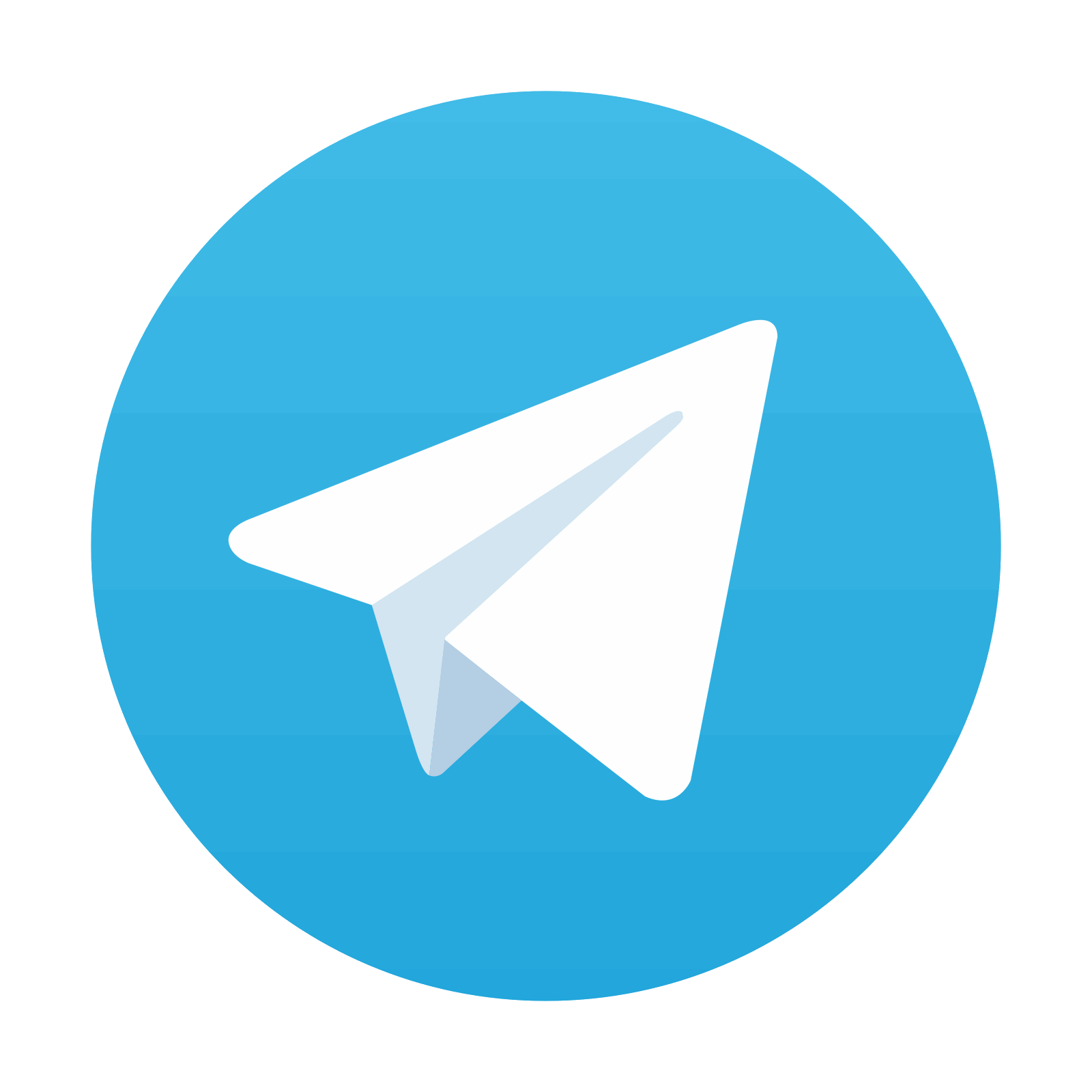
Stay updated, free articles. Join our Telegram channel

Full access? Get Clinical Tree
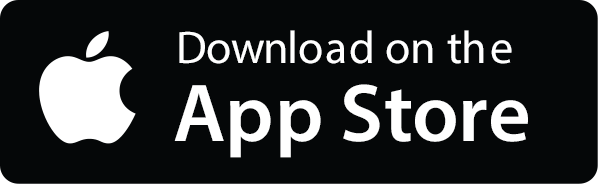
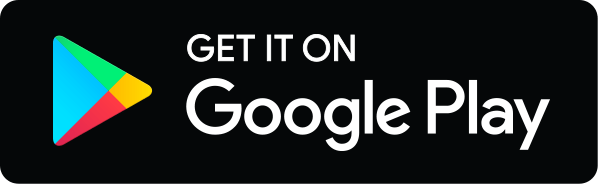
